Organ
RT side effect
Treatment
Level of evidenceb
Hematologic
Neutropenia
Consider G-CSF (see Chap. 15 for more detail)
1C
Thrombocytopenia
Platelet transfusion
1C
Anemia
Red blood cell transfusion; erythropoietin (EPO)-stimulating agents should not be utilized
1C
Skin
Dermatitis
Lotion, topical steroid 0.1 % mometasone furoate to prevent/treat irritation/pruritus; other treatments or no therapy may be equally efficacious
2C
Moist desquamation
Hydrocolloid/hydrogel dressings for comfort
2C
Ulceration/necrosis
Referral to wound care specialist
1C
CNS
Somnolence syndrome
Dexamethasone 4 mg/m2/day until improvement in symptoms
1C
Head and neck
Oral mucositis
Pain management with morphine PCA, fluid and nutritional assessment; benzydamine, LLLT, and palifermin not yet recommended (see Chap. 11 for more detail)
1C
Xerostomia
IMRT for salivary gland sparing; oral mucosal lubricants/salivary substitutes; consider pilocarpine, acupuncture
1C
Gastrointestinal
Esophagitis
Supportive care with nutritional assessment and H2 blocker or PPI for GER; consider promotility agent, rule out infectious etiologies
1C
Nausea/vomiting
Supportive care with nutritional assessment; 5-HT3 receptor antagonist ± dexamethasone (see Chap. 10 for more detail)
1C
GI mucositis
Bowel care and nutritional assessment; loperamide for diarrhea; atropine or octreotide for refractory cases; Lactobacillus spp. or sulfasalazine not yet recommended
1C
Lung
Pneumonitis
Antitussive; prednisone 1–2 mg/kg/day
2C
Bladder
Cystitis
Anesthetic agents (e.g., pyridium) and antispasmodics (e.g., oxybutynin); copious bladder irrigation for hemorrhagic cystitis, consider HBO or intravesical therapy
1C
13.2 Hematologic Toxicity
In a retrospective cohort of adult patients, MacManus et al. (1997) showed the most significant risk factors for neutropenia and thrombocytopenia were concurrent use of myelosuppressive chemotherapy as well as increasing percentage of marrow irradiated. They specifically describe that the odds ratio for myelosuppression increases for each additional 20 % of marrow irradiated. Children, unlike adults, have functional bone marrow in the appendicular skeleton, although the axial skeleton is most prominent with the pelvis and vertebrae serving the largest roles (Mauch et al. 1995). Pediatric protocols generally recommend treatment interruption for ANC between 0.3–0.5 × 109/L and platelet counts between 25–50 × 109/L.
13.2.1 Management of Neutropenia
The use of myeloid colony-stimulating factors (CSFs), specifically filgrastim (granulocyte colony-stimulating factor; G-CSF), pegfilgrastim, and granulocyte-macrophage colony-stimulating factor (GM-CSF), in the management of neutropenia during RT, whether resulting from radiation, concurrent chemotherapy, or both, is controversial. The 2006 American Society of Clinical Oncology (ASCO) guidelines for the use of CSFs make no specific recommendation with regard to pediatric patients receiving RT (Smith et al. 2006). The only specific mention of radiotherapy in these guidelines is a caution with concomitant use of CSFs, chemotherapy, and radiotherapy to the mediastinum due to a single adult trial in lung cancer patients which showed a highly significant increase in severe thrombocytopenia and pulmonary toxic deaths in the cohort receiving GM-CSF (Bunn et al. 1995). By contrast, the European Society for Medical Oncology (ESMO) released guidelines in 2009 recommending primary prophylaxis with CSFs in high-risk situations including ANC <1.5 × 109/L due to radiotherapy of >20 % of the bone marrow (Crawford et al. 2009).
Small adult studies have shown a decrease in RT treatment interruption secondary to neutropenia with the use of G-CSF; yet, what effect, if any, this had on local control and outcome are unclear (MacManus et al. 1993, 1995; Fyles et al. 1998a; Su et al. 2006; Kalaghchi et al. 2010). Larger meta-analyses on the use of G-CSF after chemotherapeutic regimens and hematopoietic stem cell transplant (HSCT) have failed to show an improvement in overall survival and transplant-related mortality (Dekker et al. 2006; Sung et al. 2007a; Center for International Blood and Marrow Transplant Research et al. 2009). A potential benefit in morbidity from radiation-induced neutropenia is unclear although no study has shown any significant risk or toxicity with the use of CSFs beyond bone pain and local reactions. Similarly, there are no data in regard to the timing of CSF delivery; for instance, CSFs could be used as primary prophylaxis to prevent the development of neutropenia in high-risk cases or secondarily to treat neutropenia once it has occurred. Current pediatric treatment protocols suggest the use of CSFs in both such cases. See Chap. 15 for more detail on CSF usage.
13.2.2 Management of Thrombocytopenia
Platelet transfusion remains the mainstay of treatment for radiation-induced thrombocytopenia. Guidelines are lacking in both the adult and pediatric literature as to what the appropriate threshold for platelet transfusion should be and thus transfusion criteria are variable between pediatric institutions, clinicians, and chemotherapeutic protocols (Wong et al. 2005). Clinicians should take into account potential risk factors which will help aid in the determination of an appropriate platelet threshold. For instance, fever, minor bleeding, coagulopathy and mucositis are potential clinical complaints which might increase the platelet transfusion threshold from 10 × 109/L to 20–50 × 109/L though evidence-based data are lacking. From a radiation standpoint, radiation fields with a high degree of marrow involvement such as a pelvic or craniospinal field or those fields potentiating the risk of mucositis should also increase platelet transfusion thresholds from 10 × 109/L to 30–50 × 109/L though evidence-based data are lacking. Close monitoring and clinical judgment are warranted.
Thrombopoietin receptor agonists (TPO-RAs) including eltrombopag and romiplostim have recently been approved in adults for multiple indications including chemotherapy-induced thrombocytopenia (Bussel et al. 2006). As yet, very limited data are available on their efficacy for chemotherapy-induced thrombocytopenia and no pediatric data are available (Basciano and Bussel 2012). It is unclear if TPO-RAs will prove beneficial after chemotherapy- and secondarily after RT-induced thrombocytopenia. Additionally, concerns remain in regard to the safety of these agents specifically in pediatric patients due to reports of thrombocytosis, thrombosis, tumor/leukemia cell growth and bone marrow fibrosis (Kuter 2007; Gernsheimer 2008; Basciano and Bussel 2012).
13.2.3 Management of Anemia
Hypoxia in solid tumors, especially head and neck, uterine cervix, and bladder cancers, and possibly soft tissue sarcomas, has been shown to be an important component in the decreased effectiveness of RT to promote tumor cell death (Dische et al. 1983; Overgaard and Horsman 1996; Fyles et al. 1998b; Brizel et al. 1999; Vaupel et al. 2001; Dunst et al. 2003; Pinel et al. 2003; Harrison and Blackwell 2004; Nordsmark and Overgaard 2004; Nordsmark et al. 2005; Overgaard 2007). Causes for tumor hypoxia are multifactorial and include abnormalities in tumor microvasculature, increased diffusion distances and underlying anemia (Vaupel et al. 2001; Vaupel and Harrison 2004). In normal cells, tissue hypoxia eventually leads to cell death while tumor cells have adapted cellular pathways, such as by upregulation of hypoxia-inducible factor 1 (HIF1), to allow for survival and growth in such conditions (Harris 2002; Dewhirst et al. 2008). Tumor hypoxia has independently been shown to be a poor prognostic factor representing an aggressive phenotype (Brizel et al. 1999; Harris 2002; Harrison and Blackwell 2004; Vaupel and Harrison 2004; Nordsmark et al. 2005; Vaupel 2008).
Determining what role underlying anemia has in tumor tissue hypoxia is as yet to be fully understood. Brizel et al. (1999) showed that even in non-anemic patients, 50 % of tumors were still poorly oxygenated. Studies in patients with head and neck and uterine cervix cancers undergoing radiation therapy have shown that patient outcomes are worse with underlying anemia (Grogan et al. 1999; Thomas 2001; Dunst et al. 2003; Prosnitz et al. 2005; Hoff et al. 2011). Yet, it is not well understood whether anemia causes poor outcomes secondary to the ineffectiveness of therapy or, plausibly, is a marker of tumor aggressiveness and the severity of the underlying disease (Harrison and Blackwell 2004; Prosnitz et al. 2005; Hoff et al. 2011). Effectiveness of transfusion was mixed with Grogan et al. (1999) and Thomas (2001) showing that transfusion could overcome negative pretreatment and average weekly nadir hemoglobin during radiotherapy for cervical cancer, while Hoff et al. (2011) showed no improvement in outcomes based on transfusion for patients with head and neck cancers. Nordsmark et al. (2005) showed that tumor hypoxia, independent of hemoglobin concentration, was singularly associated with poor outcomes in adult patients with head and neck cancers.
Multiple xenograft studies have shown a potential benefit with the use of erythropoietin (EPO)-stimulating agents (ESAs) to increase tumor radiosensitivity and potentially improve patient outcomes (Pinel et al. 2003; Stüben et al. 2003; Ning et al. 2005). Yet, clinical studies with uterine cervix and head and neck cancers and a recent Cochrane review of adult patients with head and neck cancers have failed to show a benefit in outcome with ESAs concurrent with RT (Thomas et al. 2008; Hoskin et al. 2009; Lambin et al. 2009). Additionally, meta-analyses of ESA usage in adult patients are troubling due to increased risk of thromboembolism and possible increased mortality (Bohlius et al. 2006; Bennett et al. 2008). Pediatric data are lacking. Recently updated American Society of Hematology (ASH) and ASCO guidelines by Rizzo et al. (2010) recommend ESAs with caution for adult patients with chemotherapy-induced anemia and hemoglobin (hgb) <10 g/dL. No mention is made of ESA usage for treatment of radiation-induced anemia. Pediatric consensus guidelines by the French National Cancer Institute recommend avoiding systematic administration of ESAs in pediatric cancer patients with anemia (Marec-Berard et al. 2009).
Without any pediatric data, it is difficult to imply what potential benefit transfusion may impart to solid tumor patients, such as patients with soft tissue sarcomas, undergoing radiotherapy. Patients with leukemia, lymphoma and germ cell tumors will likely not benefit due to the inherent radiosensitivity of such tumor types. Additionally, it is unclear what level of hemoglobin would be optimal for radiosensitization. In the adult head and neck cancer studies, hgb <13 g/dL was prognostic although again transfusion did not prove useful in altering outcomes (Dunst et al. 2003; Prosnitz et al. 2005). The cervical cancer studies, on the other hand, showed benefit of transfusion, keeping the hgb ≥12 g/dL (Grogan et al. 1999; Thomas 2001). Survey of pediatric oncologists’ blood transfusion practice with concurrent radiotherapy showed a bimodal distribution, with 47 % of respondents transfusing for hgb >9 g/dL (Wong et al. 2005). This again underscores the lack of clear evidence-based guidelines to provide for a more uniform treatment strategy, with data to date not supporting transfusion.
13.3 Central Nervous System Complications
Risk factors for radiation-induced brain and spinal injury include higher total radiation dose, increased dose fractions (e.g., >180–200 cGy/dose), extended radiation field volume and concomitant usage of central nervous system (CNS) toxic drugs such as intrathecal methotrexate (New 2001; Butler et al. 2006; Chopra and Bogart 2009; Rinne et al. 2012). The developed brain is able to tolerate high total RT doses with a 5 % chance of radiation necrosis with daily fractionated doses to a total effective dose of 120 Gy (Lawrence et al. 2010). In comparison, the adult brain stem and spinal cord can tolerate effective doses of 54 Gy (Kirkpatrick et al. 2010; Mayo et al. 2010). Maximum standard of care doses are generally below these threshold levels.
Acute neurologic complications include paresthesias, seizures, encephalopathy, myelopathy, paralysis and coma and are most likely secondary to underlying brain and spinal pathology and the resulting alteration in the blood-brain barrier and tumor edema (and potential mass effect) which occurs with RT (Keime-Guibert et al. 1998; Chopra and Bogart 2009; Soussain et al. 2009; Rinne et al. 2012). Management of these symptoms may require hospitalization as well as medications such as anticonvulsants and steroids (e.g., dexamethasone) to reduce symptomatic edema. Unlike adults, radiation fatigue has not been reported in pediatric patients and is likely quite rare in this population. Methylphenidate can be used to treat fatigue as in adults if present (Butler et al. 2006). Somnolence syndrome, a subacute toxicity, has been reported in pediatric patients undergoing CNS irradiation (Sect. 13.3.1). Late complications include cerebral edema, radionecrosis, leukoencephalopathy, neuroendocrine dysfunction, neurocognitive delay and secondary development of brain tumors; a discussion of these is beyond the scope of this chapter (Keime-Guibert et al. 1998; Butler et al. 2006; Chopra and Bogart 2009; Soussain et al. 2009; Rinne et al. 2012).
13.3.1 Somnolence Syndrome
Somnolence syndrome was first described in 1929 in children receiving scalp irradiation for the treatment of ringworm and has since become associated with prophylactic cranial irradiation in children with acute lymphoblastic leukemia (ALL) (Freeman et al. 1973). Freeman et al. (1973) noted that 39 % of ALL patients developed pronounced symptoms including lethargy, excessive sleeping (up to 20 h per day), anorexia, headache, irritability, fever, nausea and vomiting and transient cognitive dysfunction. An additional 39 % had mild symptoms. All patients received 24 Gy cranial irradiation (spinal irradiation ranged from 10 to 24 Gy) and there was no difference in frequency in somnolence between those children that received concomitant intrathecal methotrexate versus those who received RT alone. Mean onset of symptoms was 38 days after the completion of RT with symptoms resolving spontaneously without neurologic sequelae in a median of 18.5 days. Electroencephalogram done in a small subset of patients showed rhythmic slowing which improved with resolution of symptoms. Somnolence syndrome has also been reported after total body irradiation (TBI) with HSCT and in adult patients with primary brain tumors (Miyahara et al. 2000; Powell et al. 2011).
Follow-up studies have confirmed the findings of Freeman et al. (1973), showing a frequency of symptoms ranging from 58–71 % of patients (Parker et al. 1978; Ch’ien et al. 1980; Littman et al. 1984; Vern and Salvi 2009). Berg et al. (1983) performed neuropsychological testing on 48 children with ALL 1.5 and 3.75 years after somnolence syndrome and found no significant difference in cognitive function compared to 31 children with ALL who had not experienced somnolence after RT. Littman et al. (1984) compared the daily fractionated dose of RT, giving 100 and 180 cGy to a total dose of 18 Gy, and found the same rate of somnolence syndrome in both groups.
Symptoms of somnolence syndrome are thought due to demyelination injury of oligodendrocytes (Butler et al. 2006; Rinne et al. 2012). Two studies have looked at prophylactic administration of steroids during RT. Mandell et al. (1989) showed a 3 % incidence of symptoms in patients receiving daily prednisone ≥15 mg/m2 and Uzal et al. (1998) reported an incidence of 17.6 % in patients receiving 4 mg/m2 of daily dexamethasone (there was no significant difference between studies due to small patient numbers). Current pediatric ALL protocols do not recommend prophylactic steroids during RT secondary to the lack of large multicenter prospective trials. Steroid treatment at the onset of symptoms has also shown benefit in reducing the duration of illness (Butler et al. 2006; Kelsey and Marks 2006; Rinne et al. 2012).
13.3.2 Lhermitte’s Sign
Lhermitte’s sign is a transient spinal radiation myelopathy which occurs after cervical irradiation and presents with electric-like sensations in the spine and extremities with neck flexion thought to be secondary to transient demyelination of the cord (Keime-Guibert et al. 1998; Chopra and Bogart 2009; Soussain et al. 2009). It has not been specifically reported in pediatrics but could presumably occur with a spinal tumor, especially in adolescent patients considering their increased vulnerability to spinal cord injury with radiation or intrathecal chemotherapy (Bleyer et al. 2009). Spontaneous clinical improvement occurs in months (Chopra and Bogart 2009; Soussain et al. 2009).
13.4 Skin Complications
Acute radiation skin changes are seen in up to 85–95 % of adult patients undergoing RT (though may be decreased in incidence with IMRT) and typically occur several days to weeks after commencement of RT and after multifractionated doses totaling >20 Gy (Archambeau et al. 1995; Chopra and Bogart 2009; Salvo et al. 2010; Feight et al. 2011). Dose tolerance in normal skin is 45 Gy with increasing symptoms with greater cumulative RT doses and concomitant chemotherapy (Archambeau et al. 1995). Fluorouracil and epidermal growth factor receptor inhibitors such as cetuximab have been reported to worsen radiation dermatitis in adult patients (Archambeau et al. 1995; Budach et al. 2007). Concurrent chemotherapy with radiosensitizers such as dactinomycin and doxorubicin may play a role in the severity of radiation dermatitis in pediatric patients but has not been characterized in part due to the scheduling of these agents around RT (Archambeau et al. 1995; Krasin et al. 2009). Areas most affected are those containing skin folds such as the axillae, groin and inframammary folds (Feight et al. 2011). The earliest skin changes are pruritus, mild erythema, anhydrosis, and dry desquamation progressing to tender erythema, edema, and moist desquamation and, in severe cases, ulceration and necrosis. Data on incidence of dermatitis in children are lacking. Radiation recall, which can be precipitated by multiple agents and can occur days to years after RT, most often presents as low-grade dermatitis in a previously irradiated region although more severe reactions can also occur (Burris and Hurtig 2010).
Although there are a large number of adult studies which have reported on prevention and management of acute radiation dermatitis, many have small patient numbers with conflicting results. Pediatric data are almost completely lacking with only one reported study with 45 patients (Merchant et al. 2007). Multiple systematic reviews have been conducted though which are a useful guide from which to give direction (Bolderston et al. 2006; Kedge 2009; Kumar et al. 2010; Salvo et al. 2010; Feight et al. 2011; McQuestion 2011; Chan et al. 2012; Wong et al. 2013). General management recommendations include the use of loose fitting clothing, prevention of scratching or other abrasive activities, protection from the sun with hats and sunscreen, avoidance of temperature extremes, avoidance of cornstarch or baby powder especially to skin folds, use of an electric razor rather than a straight blade, use of non-aluminum-based deodorant on intact skin, avoidance of cosmetic products in the treatment field, avoidance of swimming in lakes or chlorinated pools, and use of gentle, non-perfumed soaps and lotions (Feight et al. 2011; McQuestion 2011). Although initially thought that washing of skin and hair in the radiation field would lead to increased toxicity, multiple studies have shown this to be safe (Bolderston et al. 2006; Kumar et al. 2010; Salvo et al. 2010; Feight et al. 2011; McQuestion 2011; Chan et al. 2012; Wong et al. 2013). It is vital though that the irradiated areas be dry at the immediate time of treatment to prevent increasing the RT dose to the skin surface (Bernier et al. 2008).
Multiple topical agents for the prevention and treatment of radiation dermatitis have been studied including aloe vera, steroid creams, trolamine (Biafine®), calendula (marigold extract), hyaluronic acid (Xclair®), sucralfate, silver sulfadiazine, 3M™ Cavilon™ no-sting barrier film, and petroleum-based ointment (Aquaphor), among other more obscure substances (Salvo et al. 2010; Feight et al. 2011; McQuestion 2011; Wong et al. 2013). Certain agents such as aloe vera, sucralfate, and trolamine are clearly without benefit, while others such as calendula, Aquaphor, hyaluronic acid, steroid creams, and silver sulfadiazine are lacking in evidence (Kumar et al. 2010; Salvo et al. 2010; Feight et al. 2011; Wong et al. 2013). Benefit of silymarin (milk thistle extract) has also been reported in a nonrandomized trial (Becker-Schiebe et al. 2011). Due to the lack of consistent and well-powered results, recommendations from the systematic reviews are variable with Salvo et al. (2010) and Chan et al. (2012) favoring no topical agent, McQuestion (2011) and Feight et al. (2011) endorsing calendula and hyaluronic acid, Bolderston et al. (2006) supporting topical steroids, Kumar et al. (2010) favoring calendula, Cavilon™, and topical steroids, and Wong et al. (2013) sanctioning topical steroids and silver sulfadiazine. All agree that larger, prospective, better designed studies are required to answer the many remaining questions about efficacy. Finally, the majority of studies address prevention of symptoms, and thus there is even less evidence to support topical therapy as treatment for radiation dermatitis (Salvo et al. 2010; Wong et al. 2013).
Prophylactic oral agents including zinc, proteolytic enzymes (Wobe-Mugos E), pentoxifylline, and sucralfate and dressings for management of moist desquamation such as hydrogels and hydrocolloids (e.g., DuoDERM®, Spenco 2nd Skin®, Intrasite™), silver leaf, moisture vapor permeable (Tegaderm™), soft silicone (Mepilex®), GM-CSF impregnated, gentian violet, and more obscure dressings are also discussed in the systematic reviews (Kedge 2009; Kumar et al. 2010; Salvo et al. 2010; Feight et al. 2011; McQuestion 2011; Wong et al. 2013). As with the topical agents, poor study design and lack of power limit conclusions that can be made on any of these agents (Kedge 2009; Kumar et al. 2010; Salvo et al. 2010; Feight et al. 2011; McQuestion 2011; Wong et al. 2013). Kedge (2009) notes in her review of hydrogels and hydrocolloid dressings that although improved healing may not occur with these agents, patient comfort may be an important benefit in some cases.
13.5 Head and Neck Complications
Radiotherapy to the head and neck can lead to complications in multiple different structures including the oral mucosa, salivary glands, bone, masticatory musculature, dentition, middle and outer ear, larynx, pharynx, and upper esophagus. The most common side effects with RT to the oral mucosa and salivary glands include mucositis, taste loss, parotitis/sialadenitis, xerostomia, trismus, and osteoradionecrosis (Kielbassa et al. 2006). Mucositis and taste loss are transitory, while xerostomia can be a long-term complication although conformal radiation therapy and proper planning have been shown to reduce this risk (Kielbassa et al. 2006; Jensen et al. 2010a). Head and neck cancer patients receiving RT must also be monitored for oropharyngeal candidiasis (Bensadoun et al. 2011). Serous otitis media and externa, laryngeal edema, dysphagia, and pharyngeal dysfunction can all occur. Tooth decay, sensorineural hearing loss, osteonecrosis, and bone growth arrest are long-term effects that must be considered but are beyond the scope of this chapter.
13.5.1 Oral Mucositis
Mucositis is a common and debilitating complication of chemoradiation occurring several days to weeks after RT initiation which can lead to treatment interruption, dose-limiting toxicity, and decreased patient quality of life and is most likely to occur in patients receiving high-dose radiation for head and neck cancers (e.g., 60–70 Gy), with 85 % of adult patients clinically affected (Sonis et al. 2004; Peterson et al. 2011). Incidence is dependent on the underlying therapeutic regimen with combination chemoradiation to the head and neck being a more likely culprit as well as total body irradiation (TBI) with HSCT (Sonis et al. 2004; Peterson et al. 2011). Incidence in children has not been widely reported but is likely similar to that for adults (Allen et al. 2010; Qutob et al. 2013b). Multiple scoring systems have been utilized in the literature, and only some have been validated for children (Sonis et al. 2004; Sung et al. 2007b; Tomlinson et al. 2007).
Due to the large number of adult trials reporting on management of oral mucositis, multiple clinical practice guidelines and systematic reviews from ASCO, ESMO, MASCC/International Society of Oral Oncology (ISOO), and the Cochrane Collaboration are available in the literature to help guide management (Keefe et al. 2007; Hensley et al. 2009; Clarkson et al. 2010; Peterson et al. 2011; Worthington et al. 2011; Gibson et al. 2013). Pediatric data are limited to small studies with variable treatment designs and therefore extrapolation from adult studies is required (Allen et al. 2010; Qutob et al. 2013b). Treatment of radiation-induced oral mucositis is similar to chemotherapy-induced mucositis; the interested reader should refer to Chap. 11 for a more detailed review of mucositis treatment guidelines.
Prior to the initiation of chemoradiation, the pediatric patient should have an oral exam to determine what, if any, treatments are required to help prevent the development of mucositis and to ensure there is no interference with the radiation field (Otmani 2007; Barbería et al. 2008; Qutob et al. 2013a). If possible, cavities should be filled and fixed appliances in the radiation field removed 7–10 days before the initiation of therapy (Barbería et al. 2008). Families and patients should be advised in regard to the importance of maintaining oral hygiene including regular tooth and tongue brushing with a soft brush and fluoridated toothpaste (in children ≥18 months) and regular mouth washing (Qutob et al. 2013 b). A standardized multidisciplinary hospital oral care protocol has been shown beneficial, as well as bland rinses such as saline or sodium bicarbonate; chlorhexidine has not been proven helpful (Keefe et al. 2007; Peterson et al. 2011; McGuire et al. 2013). Nutritional status should be monitored and those at risk for malnutrition (>5 % weight loss from baseline) should be started on total parenteral nutrition (TPN) if with orogastrointestinal mucositis or have percutaneous endoscopic gastrostomy (PEG) placement if with a head and neck tumor (Ladas et al. 2005). Additionally, hydration status in patients with severe mucositis must be monitored with intravenous fluids given if required.
Multiple well-studied agents in adult patients receiving RT have not been shown beneficial including cryotherapy (i.e., ice chips, oral cooling), antimicrobial lozenges, misoprostol mouthwash, amifostine, glutamine, and pilocarpine for the prevention of oral mucositis and oral sucralfate for treatment of mucositis (Keefe et al. 2007; Hensley et al. 2009; Gibson et al. 2013; Jensen et al. 2013; Nicolatou-Galitis et al. 2013; Peterson et al. 2013; Saunders et al. 2013; Yarom et al. 2013). Therapies that have shown potential benefit include prophylactic zinc, morphine mouth rinse, doxepin rinse, morphine patient-controlled analgesia (PCA), use of midline radiation blocks (with EBRT, not utilized with IMRT), benzydamine (locally acting nonsteroidal anti-inflammatory), low-level laser therapy (LLLT), and palifermin (keratinocyte growth factor-1 [KGF-1]) (Keefe et al. 2007; Hensley et al. 2009; Clarkson et al. 2010; Peterson et al. 2011; Gibson et al. 2013; Migliorati et al. 2013; Nicolatou-Galitis et al. 2013a; Raber-Durlacher et al. 2013; Saunders et al. 2013; Yarom et al. 2013). The strongest evidence in the adult literature exists for benzydamine, LLLT and palifermin. Of note, benzydamine has not been approved by the United States Food and Drug Administration.
LLLT is recommended in adult patients receiving HSCT with or without TBI and head and neck patients undergoing RT (Peterson et al. 2011; Migliorati et al. 2013). A pilot study of LLLT in children showed potential benefit in the prevention of chemotherapy-induced mucositis (Abramoff et al. 2008). Palifermin, 60 mcg/kg/day, for 3 days prior to conditioning and 3 days posttransplantation for autologous (and possibly allogeneic) HSCT with TBI has shown benefit in adult patients (Keefe et al. 2007; Hensley et al. 2009; Raber-Durlacher et al. 2013). Recent studies in adult head and neck cancer patients are promising in reducing the severity and duration of mucositis symptoms although without improvement in event-free and overall survival (Le et al. 2011; Henke et al. 2011). Data on palifermin usage in the pediatric population are significantly limited. Srinivasan et al. (2012) recently reported on a phase I dose-finding study in 12 children receiving myeloablative HSCT and found that 90 mcg/kg/day was tolerated with skin rash being the most common side effect; mucositis was seen in only 25 % of the cohort but without a comparative control group. See Chap. 11 for a full discussion regarding oral mucositis and mouth care.
13.5.2 Dysgeusia
Patients undergoing radiation for head and neck tumors are at risk for altered taste due to direct radiation effect on the fungiform papillae and taste buds (Otmani 2007). Taste loss can precede mucositis with histologic signs of degeneration and atrophy occurring after 10 Gy, with taste loss increasing exponentially with higher cumulative RT doses, and with bitter and acid flavors being most affected (Kielbassa et al. 2006). Pediatric patients may develop anorexia due to dysgeusia and therefore nutritional status must be monitored closely. Zinc and amifostine for prophylaxis or treatment of dysgeusia in adult patients have not consistently shown benefit and are not recommended (Hovan et al. 2010). Dysgeusia usually returns to normal weeks to months after the completion of RT though in a small subsegment of patients may persist.
13.5.3 Xerostomia
A reduction in salivary function is most commonly seen with head and neck RT and has also been noted post-HSCT with and without TBI (Jensen et al. 2010a). In a systematic review by Jensen et al. (2010a), xerostomia prevalence remained >70 % from the time of development to >2 years post conventional RT. Pediatric data are limited; it appears the risk of chronic xerostomia is low although has been described after both head and neck RT and TBI conditioning with HSCT (Jensen et al. 2010a). Xerostomia risk is dose related, with minimal risk at mean doses of 10–15 Gy to the parotid gland and a decrease in glandular function >75 % with mean doses >40 Gy (Deasy et al. 2010). Xerostomia risk has been reported to be significantly reduced with sparing of one parotid or even, potentially, one submandibular gland, with IMRT although in the review by Jensen et al. (2010a) prevalence of xerostomia after IMRT was similar to that noted with conventional RT at all time points due to variation in technique and ability to spare the parotid and submandibular glands (Saarilahti et al. 2006).
Multiple potential management strategies exist in the adult literature and have most recently been systematically reviewed by Jensen et al. (2010b) as part of MASCC/ISOO. Recommendations include the use of IMRT with salivary gland sparing when oncologically feasible, muscarinic agonist stimulation (pilocarpine over newer and less well-studied agents cevimeline and bethanechol) after RT completion (but not during RT), oral mucosal lubricants/salivary substitutes, salivary gland transfer in strictly selected cases, and acupuncture to stimulate salivary gland secretion (Jensen et al. 2010b). Agents that are not recommended include amifostine (as opposed to the 2008 ASCO guidelines by Hensley et al. [2009]), gustatory and masticatory stimulation (sugar-free lozenges, acidic candy, chewing gum), and hyperbaric oxygen (HBO) therapy (Jensen et al. 2010b). Pediatric data on such interventions are extremely limited.
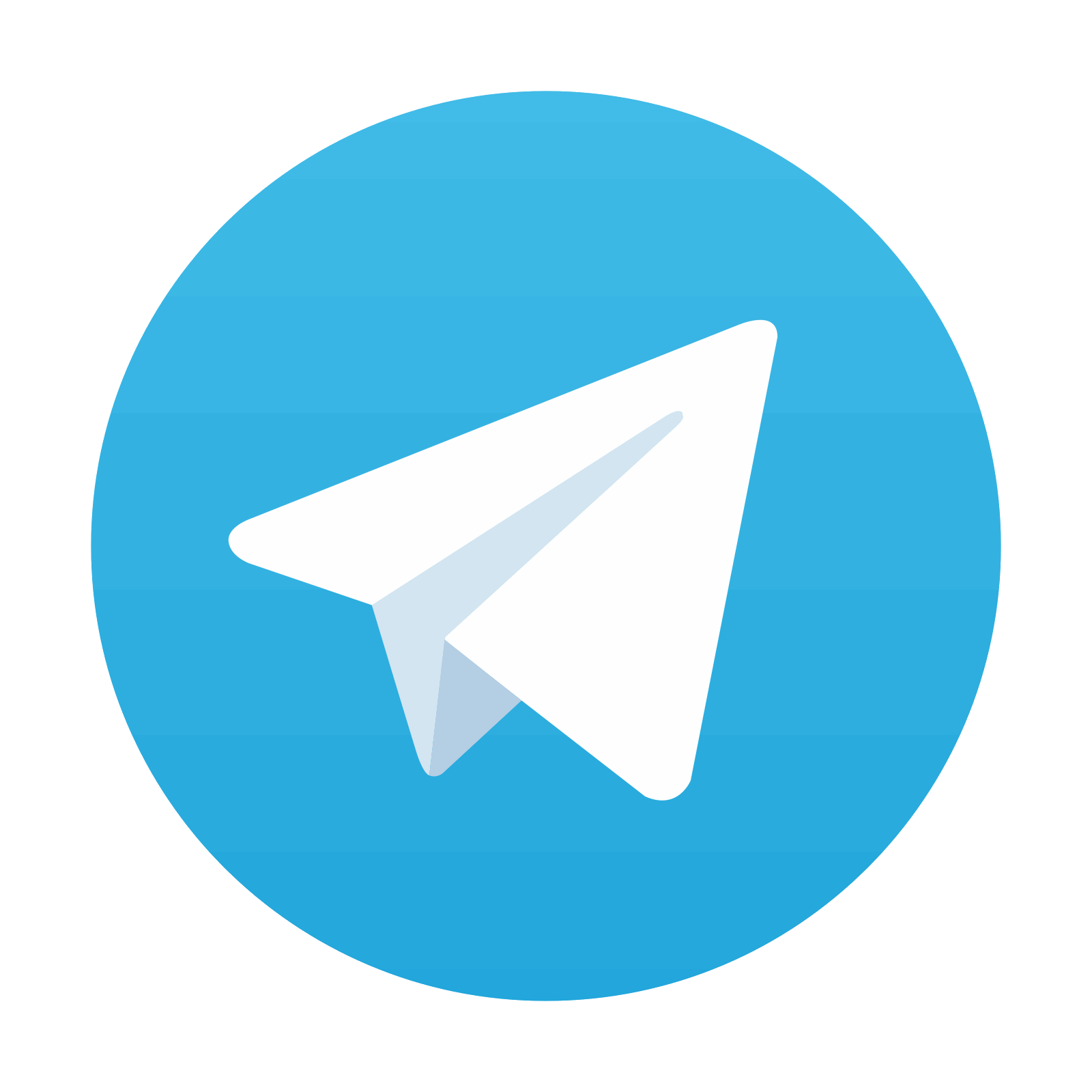
Stay updated, free articles. Join our Telegram channel

Full access? Get Clinical Tree
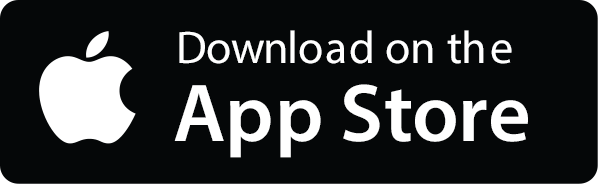
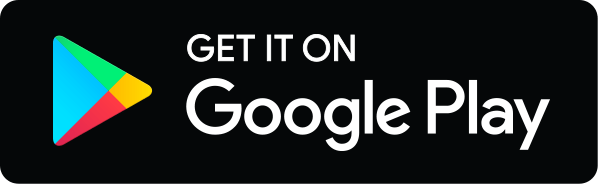