© Springer International Publishing AG 2017
Jeffrey Y.C. Wong, Timothy E. Schultheiss and Eric H. Radany (eds.)Advances in Radiation OncologyCancer Treatment and Research10.1007/978-3-319-53235-6_11The Mammalian DNA Damage Response as a Target for Therapeutic Gain in Radiation Oncology
(1)
Department of Radiation Oncology, City of Hope National Medical Center, 1500 E. Duarte Rd., Duarte, CA 91010, USA
Abstract
Mutant cells that are defective for certain components of the mammalian DNA damage response (DDR) have been shown to display hypersensitivity to killing by ionizing radiations; these findings have prompted the idea that drugs that emulate these DDR deficiencies might serve as clinically useful radiosensitizers for improving results in cancer therapy. In this chapter, the ways in which several agents now established as radiosensitizers do in fact function by inhibiting parts of the DDR are first presented. The various subsystems of the DDR are next reviewed, and several potential molecular targets for discovery or design of chemical modifiers that could lead novel radiosensitizing drugs are discussed.
Keywords
DNA Damage Response (DDR)DNA RepairDouble Strand Break (DSB)Homologous Recombination Repair (HR)Non-homologous End Joining Repair (NHEJ)Radiation therapy (XRT)RadiosensitizerTherapeutic Ratio (TR)1 The Challenge of Continuing to Improve the Therapeutic Ratio for Radiation Therapy in Human Oncology
Radiation therapy (XRT) continues to be a key modality in modern cancer medicine for both curative and palliative management of a variety of malignant diseases. It has been suggested that the role radiation therapy plays in obtaining local-regional control of tumors might in fact become increasingly important in the coming years as innovative systemic therapies, such as molecularly targeted agents and immune checkpoint modulators, improve our ability to eradicate microscopic metastatic disease for some patients (Citrin and Mitchell 2014). The recent 1–2 decades have witnessed impressive technical advances in the planning and delivery of XRT including multiple imaging modality simulation, 4-dimensional treatment planning and delivery, practical multibeam intensity modulated XRT, and daily image guidance for target positioning verification; several of these have supported development of effective new XRT approaches such as stereotactic body radiotherapy. Improving the conformality of XRT dose delivery in both space and time through these approaches is expected to improve the therapeutic ratio (TR) for XRT by minimizing normal tissue toxicity (Citrin and Mitchell 2014).
An improved TR for XRT can also be achieved by selectively enhancing the lethal effects of ionizing radiation (IR) on tumor versus normal tissues (Citrin and Mitchell 2014; Jekimovs et al. 2014; Gavande et al. 2016). Such an outcome might be obtainable by targeting certain features of malignant disease such as intratumoral hypoxia or host antitumor immune responses for example, but much current interest is centered upon manipulating biological IR responses at the level of individual malignant cells (Citrin and Mitchell 2014; Jekimovs et al. 2014; Gavande et al. 2016; Higgins et al. 2015; Raleigh and Haas-Kogan 2013). The term radiosensitizer properly refers to a chemical that increases cell death in response to a given dose of IR while being completely innocuous to cells in the absence of IR treatment (Citrin and Mitchell 2014; Higgins et al. 2015), although the term is often informally applied to agents such a chemotherapeutic drugs that can themselves be toxic to cells at sufficient doses; the latter are instead properly called chemical modifiers of cellular radiation response (Citrin and Mitchell 2014; Higgins et al. 2015). Drugs that usefully enhance tumor responses to IR are already extremely important in the clinic. Recent large gains in our understanding of the molecular genetics of cancer, along with ever-improving approaches to protein structure-based drug design, point to the strong likelihood that new, potent chemical modifiers for use with XRT will contribute to advances in human cancer medicine. This brief chapter will update and expand upon several recent excellent reviews of this topic (Citrin and Mitchell 2014; Jekimovs et al. 2014; Gavande et al. 2016; Higgins et al. 2015; Raleigh and Haas-Kogan 2013; Begg et al. 2011).
2 Radiosensitizing Agents Targeting the DNA Damage Response: Established Radiosensitizing Agents
2.1 Overview
Formation of DNA double strand breaks (DSB) at sufficient levels in human and other mammalian genomes, as by IR or certain chemotherapeutic agents, activates a complex signal transduction cascade—the DNA Damage Response (DDR)—that culminates in a range of cellular outcomes including repair of the DNA damage, cell cycle arrest at specific checkpoints, and programmed cell death; the DDR and these various endpoints have been reviewed extensively (Begg et al. 2011; Goodarzi and Jeggo 2013; Ceccaldi et al. 2016; Jeggo and Lobrich 2015; Waters et al. 2014; Jackson 2009) and will be covered here only briefly. Natural and engineered mammalian cells and animals that have gene mutations causing functional defects for components of the DDR are commonly IR hypersensitive to varying degrees, along with displaying “genomic instability” (see later). Human patients and mutant mouse strains with germline DDR gene defects often display increased susceptibility to various malignancies; some also have immunodeficiency related to a failure to repair the programmed DSB formed during the course of immunoglobulin and T-cell receptor gene maturation (Goodarzi and Jeggo 2013; Jackson 2009; Curtin 2012; Weinberg and Hanahan 2011). The finding that DDR gene mutations can cause mammalian cell IR hypersensitivity has given the impetus in recent years for discovery of chemical compounds that could inhibit the functions of various DDR proteins and emulate the effects of such gene defects (sometimes called “pharmacological phenocopy”); these agents would be expected to be candidate preclinical cellular radio- and chemo-sensitizers that might ultimately lead to useful drugs for human cancer medicine (Citrin and Mitchell 2014; Jekimovs et al. 2014; Gavande et al. 2016; Higgins et al. 2015; Raleigh and Haas-Kogan 2013). The finding that inhibition of different facets of the DDR is in fact the molecular mechanism of action for some radiosensitizers now used clinically, as detailed next, serves to validate this strategy.
2.2 Targeting DNA Damage Sensing/Signaling Pathways
Following induction of DSB in human nuclear DNA, their presence is sensed by the Mre11/Rad50/NBS1 (MRN) protein complex, which is recruited to the break ends by the human single stranded binding protein 1 (hSSB1); MRN then promotes association of the key damage signaling kinase Ataxia Telangiectasia Mutated (ATM) with the DSB (Begg et al. 2011; Goodarzi and Jeggo 2013; Ceccaldi et al. 2016). ATM kinase is thereby activated toward phosphorylation of itself, of the components of the MRN complex, and of the variant histone H2AX (thereby generating γH2AX) (Begg et al. 2011; Goodarzi and Jeggo 2013; Ceccaldi et al. 2016). γH2AX formation then propagates for many thousands of base pairs from the DSB ends into the DNA strands, and initiates assembly of an array of additional DDR proteins to form a molecular macrostructure, the ionizing radiation induced focus (IRIF) (Goodarzi and Jeggo 2013). ATM signaling is amplified by interactions within the IRIF and then transduced to downstream effectors such as the CHK2 kinase-p53 axis to initiate cell cycle arrest or apoptosis (Begg et al. 2011; Goodarzi and Jeggo 2013; Ceccaldi et al. 2016). Cells with deficient MRN function show severely impaired ATM signaling (Begg et al. 2011; Goodarzi and Jeggo 2013; Ceccaldi et al. 2016). The MRN complex (in particular, the Mre11 nuclease) plays an additional key role in the cellular “choice” between utilization of homologous recombination (HR) versus non-homologous end joining (NHEJ) mechanisms for the repair of DSB in the late-S and G2 phases of the cell cycle ((Begg et al. 2011; Goodarzi and Jeggo 2013; Ceccaldi et al. 2016) and see below). The observed IR sensitivity of MRN function-deficient cells thus makes sense.
Heat treatment of sufficient temperature and duration very potently sensitizes malignant human cells to killing by IR, a phenomenon termed hyperthermic radiosensitization (HtRs) (Dewey 2009). Given the magnitude of this effect, clinical application of HtRs has been of interest for decades, but implementation of this has been hindered previously by the engineering challenges connected with heating deep tumors in situ; this obstacle might now be overcome, however, with the development of MR-guided focused ultrasound technology (see Chapter XX of this text). The molecular basis of HtRs has been elucidated using a genetic approach termed epistasis analysis; this strategy is predicated on the fact that two separate functional defects (“hits”) in the same mechanistic pathway should have no more consequence than either single hit alone does. In contrast, hits to separate pathways that function in parallel in a complementary fashion (for example, the NHEJ and HR mechanisms for DSB repair; see below) typically have a greater impact on cell physiology than either hit alone. Previous work had implicated one or more of the MRN complex protein components in HtRs, possibly via active export of these proteins out of the nucleus in response to heat treatment (Seno and Dynlacht 2004). The effect heating on clonogenic inactivation by IR was next investigated using cells having natural (cells derived from Mre11- or Nbs1-defective patients) or engineered (siRNA knockdown) deficiencies of Mre11, Nbs1, or Rad50 function (Dynlacht et al. 2011). For Nbs1 and Rad50, unheated cells were hypersensitive to killing by IR compared to normal cells, but that sensitivity was increased still further by the heat treatment. In contrast, heating of the Mre11 cells did not alter their already marked sensitivity to IR (Dynlacht et al. 2011). Purified Mre11 protein was also found to be unusually sensitive to heat denaturation in vitro (Dynlacht et al. 2011). The interpretation of these findings is that the Mre11-mutant cells are already maximally deficient for MRN-ATM signaling after DNA damage so that heat denaturation of any residual Mre11 protein has no consequence. In contrast, low levels of Nbs1 or Rad50 activity in the respective mutant cells did support some MRN-ATM signaling after IR, and this was abolished by total heat inactivation of cellular Mre11. In keeping with these findings, recently developed small molecule inhibitors of the Mre11 endo- and exonuclease activities are radiosensitizing agents in vitro (Shibata et al. 2014).
2.3 Targeting DSB Repair Pathways
Repair of DNA DSB in eukaryotic cell relies on two fundamentally distinct mechanisms, nonhomologous end joining (NHEJ) and homologous recombination (HR) repair (sometimes called homology directed repair) (Begg et al. 2011; Goodarzi and Jeggo 2013; Ceccaldi et al. 2016; Waters et al. 2014; Pannunzio et al. 2014; Moynahan and Jasin 2010). NHEJ entails the nucleolytic processing and direct ligation of DSB ends that may approximately restore the original configuration of a stretch of DNA (typically with loss of some of the nucleotides adjacent to the strand breaks). Alternatively, DNA ends from remote parts of the genome might be brought together by NHEJ repair, forming a chromosomal translocation or inversion (Begg et al. 2011; Goodarzi and Jeggo 2013; Ceccaldi et al. 2016). The kinetics of end joining by this mechanism is determined by the chemistry of the DNA termini and by the chromatin configuration of the DNA within which the DSB occurs; chemically complex ends produced by relatively high LET radiations, and the compacted chromatin associated with heterochromatic chromosomal regions lead to slower end rejoining (Begg et al. 2011; Goodarzi and Jeggo 2013; Ceccaldi et al. 2016). Quite recently, it has been possible to distinguish between so-called canonical NHEJ (the mechanism identified first) and one or more “alternative” NHEJ pathways (Begg et al. 2011; Goodarzi and Jeggo 2013; Ceccaldi et al. 2016; Pannunzio et al. 2014); these differ with respect to the specific proteins involved and the precise molecular details of DNA end synapsis during DSB end rejoining (Begg et al. 2011; Goodarzi and Jeggo 2013; Ceccaldi et al. 2016; Pannunzio et al. 2014). How these various NHEJ processes might be differently targeted for achieving radiosensitization remains to be seen.
HR repair of DSB is initiated by quite extensive exonucleolytic degradation of DNA, starting at the break ends and proceeding in the 5′ → 3′ direction, thereby forming long single strands having 3′ hydroxyl termini (Begg et al. 2011; Goodarzi and Jeggo 2013; Ceccaldi et al. 2016; Moynahan and Jasin 2010). Those strands invade nearby intact homologous DNA (this is typically afforded by the adjacent sister chromatid following replication) and prime the synthesis of new DNA corresponding to the regions surrounding the DSB, using the intact complementary strands as templates. The new DNA strands are then extracted from the sister chromatid and reannealed; ligation of the new DNA 3′ ends to 5′ ends of the broken chromatid completes repair of the DSB (Begg et al. 2011; Goodarzi and Jeggo 2013; Ceccaldi et al. 2016; Moynahan and Jasin 2010).
HR contrasts with NHEJ in that the latter has no mechanistic requirement for the presence intact homologous DNA nearby (Begg et al. 2011; Goodarzi and Jeggo 2013; Ceccaldi et al. 2016). For this reason, NHEJ is practicable throughout the cell cycle, while HR is confined to the G2 phase and to those genomic regions that have already undergone replication during S phase (Begg et al. 2011; Goodarzi and Jeggo 2013; Ceccaldi et al. 2016). NHEJ appears to be responsible for the majority of DSB repair throughout the cell cycle in mammalian cells, this despite it being much more “error prone” than HR, given the likelihood of small DNA sequence changes in the vicinity of the DSB and the potential to form gross chromosomal aberrations (Begg et al. 2011; Goodarzi and Jeggo 2013; Ceccaldi et al. 2016; Pannunzio et al. 2014). The mechanistic basis for the cellular “decision” between use of NHEJ versus HR for the repair of a given DSB during G2 or S phase is at present poorly understood and is the subject of much research activity, although the MRN complex appears to be intimately involved (Begg et al. 2011; Goodarzi and Jeggo 2013; Ceccaldi et al. 2016; Shibata et al. 2014).
2.3.1 Targeting the NHEJ Pathway
Curative-intent treatment programs combining XRT and a platinum-containing chemotherapeutic drug such as cisplatin (CDDP) are widely used in human oncology, and have led to improved clinical outcomes for different forms of lung cancer, head and neck cancer, and carcinoma of the uterine cervix among other malignancies (Sears et al. 2016). The mechanistic basis for radiosensitization by platinum-containing drugs has been investigated in vitro. An epistasis analysis showed that CDDP treatment did not sensitize the killing of NHEJ-deficient cells by IR, while HR-defective were markedly sensitized (Raaphorst et al. 2005); this result indicates that NHEJ does not function properly in CDDP-treated cells. NHEJ can be assayed in vitro in mammalian cells extracts by following ligation of linear substrate DNA molecules (Sears and Turchi 2012; Diggle et al. 2005). The presence of CDDP damage in the substrate molecules inhibited NHEJ in vitro (Sears and Turchi 2012; Diggle et al. 2005), while extracts prepared from CDDP-treated cells were NHEJ-competent for repair of undamaged substrate DNA molecules (Sears and Turchi 2012); these results support the model that it is CDDP adducts in DNA near DSB are a block to repair by NHEJ. In keeping with this model, so-call host reactivation of transfected substrate DNA molecules by NHEJ is proficient for undamaged substrates transfected into CDDP-treated cells, but deficient for CDDP-damaged substrates transfected into untreated cells (Sears and Turchi 2012).
2.3.2 Targeting the HR Pathway
The HR pathway for DSB repair depends upon synthesis of long stretches of new DNA using an undamaged sister chromatid as the template (Begg et al. 2011; Goodarzi and Jeggo 2013; Ceccaldi et al. 2016; Moynahan and Jasin 2010); it might thus be anticipated that antimetabolite chemotherapy drugs which deprive mammalian cells of DNA synthetic precursors might serve as effective HR repair inhibitors. Gemcitabine is an antimetabolite chemotherapeutic agent that inhibits ribonucleotide reductase and the de novo synthesis of deoxynucleotide DNA precursors. Gemcitabine is also a potent radiosensitizers (Shewach and Lawrence 1995; Van Putten et al. 2001; Wachters et al. 2001). Using epistasis analysis, the mechanism of gemcitabine radiosensitization was investigated in mammalian cells deficient in NHEJ and HR (Van Putten et al. 2001; Wachters et al. 2001). Following treatment with gemcitabine, radiosensitization was observed in the NHEJ-deficient cells, but this was markedly decreased in the HR-deficient cell, demonstrating that this drug blocks the latter pathway (Van Putten et al. 2001; Wachters et al. 2001). Investigation of the mechanism of radiosensitization by various fluoropyrimidine family drugs, another class of clinically useful antimetabolite chemotherapeutic radiosensitizers, has proven to be more complex (Canman et al. 1994). This may be because, while these agents inhibit thymidine (TdR) DNA precursor synthesis, the simultaneously promote incorporation of deoxyuridine (UdR) into nascent DNA in place of TdR (Canman et al. 1994).
2.4 Targeting Prosurvival/Anti-apoptotic Signaling Pathways
Following DNA damage and several other cellular stresses, many normal cells and certain malignant cells undergo a process of programmed cell death termed apoptosis (Balcer-Kubiczek 2012). For cells exposed to IR, DDR signaling through ATM ultimately impinges upon the tumor suppressor protein p53 and activates it as a transcription factor (Begg et al. 2011; Goodarzi and Jeggo 2013; Ceccaldi et al. 2016; Balcer-Kubiczek 2012). Depending upon cellular context, p53 activation promotes either apoptosis or cell cycle arrest in G1 phase by transcriptional regulation of specific genes (Balcer-Kubiczek 2012). In the checks and balances of cellular governance, pro-apoptotic influences are countered by pro-survival signaling; the Akt serine/threonine kinases are key mediators of the latter process (Balcer-Kubiczek 2012; Toulany and Roderman 2015). Loss of proper apoptotic response to cellular stress appears to be an essential component of carcinogenesis for many cell types (Weinberg and Hanahan 2011); enhanced Akt signaling by several different mechanisms has been found to be one means by which malignant cells can achieve this abrogation of apoptosis (Cengel et al. 2007; Mckenna et al. 2003; Cheung and Testa 2013). Akt activation in tumors is associated with chemotherapy and radiotherapy treatment resistance (Cengel et al. 2007; Mckenna et al. 2003; Cheung and Testa 2013; Sekhar et al. 2011; Kao et al. 2007; Misale et al. 2012; Garrido-Laguna et al. 2012), and down regulation of Akt signaling by dominant negative inhibition or drug targeting of its upstream signaling partner PI3 kinase (PI3K) has been shown in some cases to cause radiosensitization (Tanno et al. 2004). These finding are believed to reflect, at least in some cells, mitigation of the killing effects of IR by pro-survival Akt signaling by these inhibitory interventions (Toulany and Roderman 2015; Tanno et al. 2004; Brognard et al. 2001). Importantly, however, more recent results indicate that activated Akt also plays significant, directs role in cellular responses to IR including DNA DSB sensing/signaling and regulation of NHEJ; targeting activated Akt may thus instead (or in addition) radiosensitize some cells by inhibiting DSB repair (Toulany et al. 2008, 2012; Park et al. 2009).
Akt is a downstream effector of several mitogenic (pro-growth) signaling cascades that are frequently found to be corrupted in tumor cells (Toulany and Roderman 2015; Cheung and Testa 2013); prominent among these are activating mutations and amplification of genes encoding cell surface receptor tyrosine kinases (RTKs) such as EGFR (Weinberg and Hanahan 2011; Toulany and Roderman 2015; Cheung and Testa 2013). One target of deregulated EGFR signaling in tumors, via PI3K, is Akt (Toulany and Roderman 2015; Cheung and Testa 2013). Significant correlations have been found for many tumors between EGFR activation and Akt activation (Cheung and Testa 2013; Nijkamp et al. 2011), and between EGFR activation and chemo/radiation resistance (Ang et al. 2002; Nakamura 2007); findings such as these have made EGFR signaling an attractive target for cancer therapy (Seshacharyulu et al. 2012; Dassonville et al. 2007). Cetuximab, a recombinant monoclonal antibody therapeutic that down regulates EGFR signaling has been investigated in combination with XRT patients with colon cancer, non-small cell lung cancer, and head and neck cancers (Seshacharyulu et al. 2012), and is now in routine use for the latter given its efficacy as a radiosensitizer (Bobber et al. 2010). Erlotinib, a small molecule EGFR TK inhibitor also shows radiosensitizing activity for some malignant cells, and has shown this activity in the clinic in a promising way for patients with brain metastases of non-small cell lung cancer (Zheng et al. 2016).
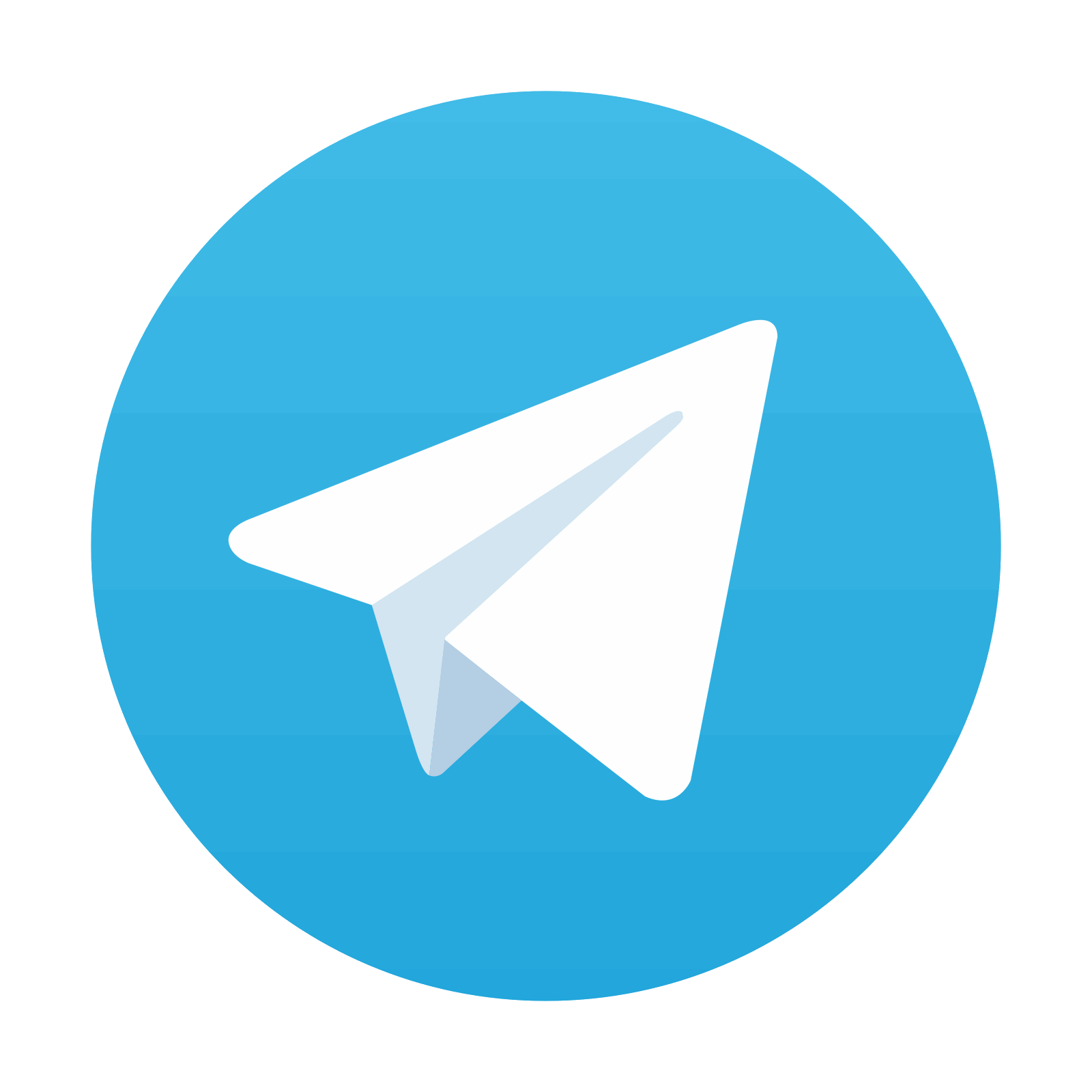
Stay updated, free articles. Join our Telegram channel

Full access? Get Clinical Tree
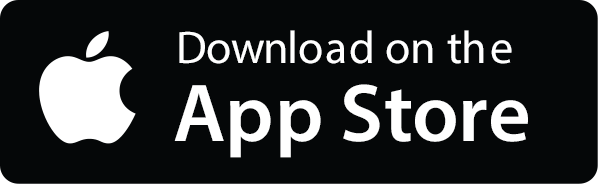
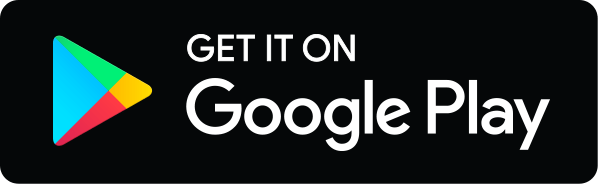