Fig. 10.1
Age-dependent incidence of melanoma. Age-dependent incidence of in-situ and invasive melanoma (top left). Age-dependent incidence of melanoma by sex (top right). Age-dependent incidence of AYA melanoma in face/scalp/neck (bottom left). Age-dependent incidence of AYA melanoma in sun-exposed trunk/thighs/shoulders/extremities (bottom right)
In the AYA population, the incidence of both invasive and in situ melanoma of the face, scalp, and neck was similar in females and males (Fig. 10.1, bottom left panel). However, the incidence of both invasive and in situ melanoma of the sun-exposed areas including the trunk, thigh, shoulders, and extremities was far more frequent in females than males (Fig. 10.1, bottom right panel).
10.2.2 Trends in Incidence of In Situ and Invasive Disease
In older adults, in situ melanoma occurred most frequently in the head and arms (Fig. 10.2, left panel). In AYA subjects, however, the predominant sites of in situ melanoma were the trunk, hip, and legs, and the least involved sites were the face, scalp, neck, and eye (Fig. 10.2, left panel). The predilection for intermittently sun-exposed areas in AYAs versus chronically sun-exposed areas in older persons implies that chronic solar exposure is not the primary mechanism of in situ melanoma in AYAs. These data are also in line with the rarity of the histologic subtype of lentigo malignant melanoma in AYA.
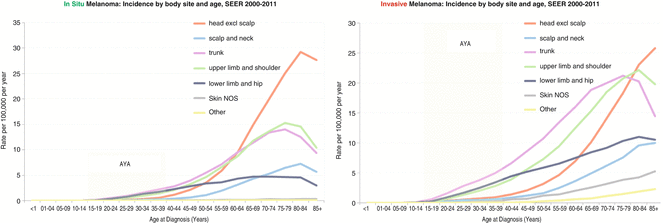
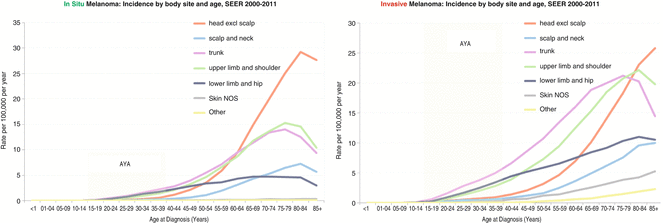
Fig. 10.2
Age-Dependent Incidence of Melanoma By Body Site and Age, SEER 2000-2011. In-situ Melanoma (left panel), Invasive Melanoma (right panel)
In AYA and persons up to the age of 80, the most frequent site of invasive melanoma was the trunk, and the least involved site was the scalp and neck. In AYA subjects, the hip and legs were the second most common sites of invasive melanoma. In older persons, the shoulder and arms were the second most common body sites (Fig. 10.2, right panel).
10.2.3 Trends in Incidence by Race/Ethnicity
At any age (except in children <10 years), non-Hispanic white individuals have a higher incidence of in situ (Fig. 10.3, top left panel) and invasive (Fig. 10.3, top right panel) melanoma than any other race or ethnicity, while blacks and Asian/Pacific Islanders had the lowest incidence. The vast majority (90 %) of melanoma diagnosed in the United States occurs in non-Hispanic white individuals.
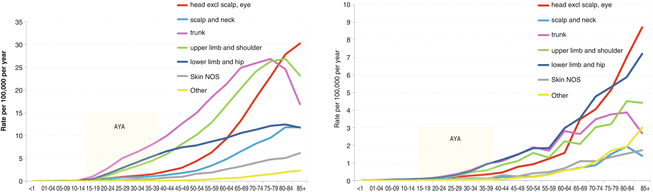
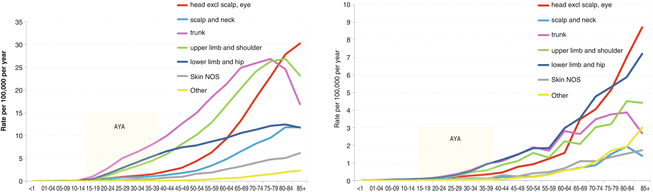
Fig. 10.3
Age-Dependent Incidence of Melanoma By Body Site in Non-Hispanic Whites (left panel). Age-Dependent Incidence of Melanoma By Body Site in Hispanic Whites (right panel)
In non-Hispanic whites, the trunk was the body site of greatest incidence in AYAs (Fig. 10.3, bottom left panel) and older adults up to the age of 80. In Hispanics, the trunk, hip, and legs were the body sites of greatest incidence in AYAs (inset) and middle-aged adults (Fig. 10.3, bottom right panel).
10.2.4 Trends in Mortality
Five-year melanoma-specific survival with in situ disease exceeded 99 % at all ages, while survival with invasive disease (stages I–IV) was lower but exceeded 85 % at all ages (Fig. 10.4, top left panel). Females had a survival advantage over males across all ages (Fig. 10.4, top left panel). Younger patients with invasive disease had greater 5-year melanoma-specific survival than older patients (Fig. 10.4, top right panel). Unsurprisingly, survival was worse in patients with no identifiable primary lesion (Fig. 10.4, bottom left panel). Five-year melanoma-specific survival of invasive melanoma was worse at all ages in blacks and Asians/Pacific Islander than in other major races/ethnicities, a difference that persisted irrespective of sex (Fig. 10.4, bottom right panel).
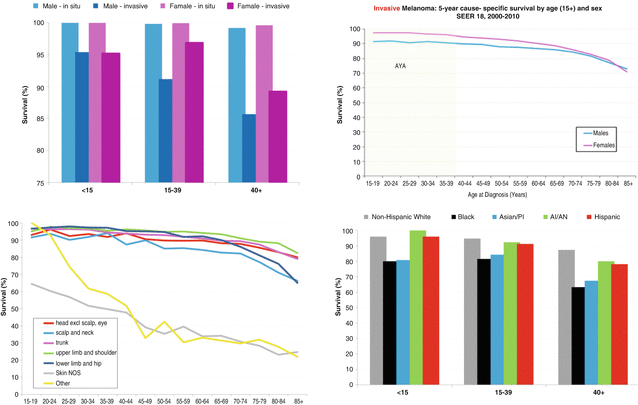
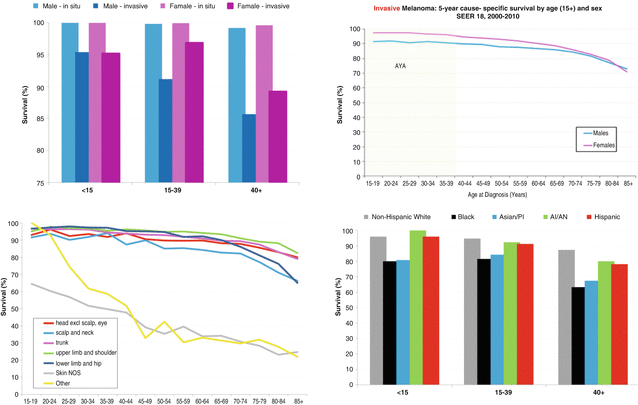
Fig. 10.4
5-Year Melanoma-Specific Survival (SEER 18, 2000-2010). 5-Year Melanoma-Specific Survival in AYA Groups (<15, 15-39, >40) (top left). Invasive Melanoma 5-Year Melanoma-Specific Survival by Age and Sex (top right). 5-Year Melanoma-Specific Survival By Primary Site (bottom left). 5-Year Melanoma-Specific Survival in AYA Groups (<15, 15-39, >40) by Race (bottom right)
10.3 Etiology and Risk Factors
10.3.1 Genetic Characterization
Early efforts to elucidate the genetic basis of cutaneous melanoma identified driver mutations involving several members of the MAPK pathway including BRAF (35–50 %) and NRAS (10–25 %) [3]. Next-generation high-throughput sequencing by The Cancer Genome Atlas (TCGA) has provided a comprehensive mutational landscape of cutaneous melanoma in adult melanoma and suggests that ~70 % of melanoma is associated with either BRAF or NRAS mutations (BRAF mutated 40–50 %; NRAS mutated 20–28 %) which directly result in activation of the MAPK pathway [4]. The vast majority of BRAF and NRAS mutations occur at sites of genomic instability (hot spots) and are not associated with the typical UV signature C→T transitions. Recent TCGA work suggests that NF1 mutations occur in 14 % of melanomas and these are mutually exclusive with BRAF/NRAS mutations [4, 5]. In contrast to BRAF and NRAS, NF1 and several other genes (ARID2, PPP6C, RAC1, SNX31, TACC1, STK19, and IDH1) and the known melanoma tumor suppressors (PTEN, p14ARF, p16INK4a) are commonly associated with C→T or G→A transitions – providing a causative link between UV exposure and melanoma oncogenesis. These data support a reclassification of cutaneous melanoma on the basis of mutational events into BRAF-mutated, RAS-mutated, NF1-mutated, and BRAF/RAS/NF1 wild-type (“triple wild-type”) cohorts – the latter comprising a group of tumors with a variety of mutations in tumor suppressors (PTEN, p14ARF, p16INK4a) and candidate oncogenes (ARID2, CKIT, PPP6C, RAC1, SNX31, TACC1, STK19, and IDH).
TCGA data were derived exclusively from adult specimens – and very little was known about the mutational spectrum of pediatric melanomas until recently. A group at St. Jude Children’s Research Hospital performed a detailed multiplatform characterization of three pediatric melanocytic lesions: “conventional” melanoma, spitzoid melanoma, and congenital nevus-derived melanoma [6]. In “conventional” melanoma, authors noted a high somatic mutation burden (14.36 mutations per megabase) and a high percentage (>80 %) of C→T single-base mutations similar to the observations in adult melanoma. Most “conventional” melanoma patients had mutations in BRAF and TERT promoter, while approximately half had non-mutually exclusive PTEN copy number changes or loss of function mutations. Conversely, congenital nevus-derived melanomas were associated with NRAS mutations and lacked TERT promoter mutations. About 40 % of spitzoid melanomas had kinase fusions involving the NTRK1, ROS, ALK, RET, and BRAF genes.
These data suggest that pediatric “conventional” melanoma and adult cutaneous melanoma are similar diseases. Similar to adult melanoma, pediatric “conventional” melanoma is associated with a high somatic mutation load and high frequencies of activating BRAF mutations and PTEN alterations with resulting activation of MAPK and PI3K/AKT cellular signaling pathways. Spitzoid melanoma and congenital nevus-derived melanoma emerge as distinct clinicopathologic entities with characteristic molecular phenotypes. The high somatic mutation burden and presence of mutated NRAS in congenital nevus-derived melanoma contrast distinctly with the absence of BRAF/NRAS mutations and low somatic mutation burden of spitzoid melanoma. Notably, both the analyses of Bastian and the St Jude’s group have identified novel kinase fusions in spitzoid melanoma that separates this group of lesions from other melanomas [7]. The kinase fusions observed are constitutively expressed and result in active oncogenes that drive malignant transformation. Further study is required to characterize the natural history of these unusual melanocytic lesions and confirm the above findings.
10.3.2 Inherited Susceptibility to Melanoma
10.3.2.1 High-Risk Loci: FAMMM, CDKN2A/CDK4 Mutations, and Xeroderma Pigmentosum
Early studies of genetic predisposition in melanoma did not distinguish clustered sporadic cases from inherited single-locus predisposition. Linkage analysis of Utah and Texas familial melanoma kindreds identified a melanoma susceptibility locus on chromosome 9p21 [8]. Concurrently, the phenotype of the familial syndrome was clarified [proband with variable number (10–100) of nevi, dysplastic features including junctional hyperplasia, and increased risk of melanoma and pancreatic malignancies], and the syndrome was termed familial atypical mole-malignant melanoma syndrome (FAMMM) or dysplastic nevus syndrome (DNS). Multiple affected first- or second-degree relatives suggested an autosomal dominant inheritance with high penetrance but variable expression.
Subsequently, investigators mapped the CDKN2A gene to this locus and established that CDKN2A encodes two proteins, p16 and p14ARF, that are transcribed in alternate reading frames through alternative first exons [9]. The p16 protein product binds to and inhibits CDK4 that, together with cyclin D, regulates G1/S phase transition during mitosis. The p14ARF protein inhibits mdm2 and promotes p53/p21 activation that inhibits CDK1/CDK2 complexes. Both p16 and p14ARF thus function as tumor suppressors, and their loss results in unrestricted cell cycle progression. CDKN2A mutations are associated with 20–57 % of hereditary melanoma and 1.3 % of sporadic melanoma, while CDK4 mutations are detected in 2–3 % of familial cohorts and only very rarely in sporadic melanoma [10–12]. Studies have reported diverse estimates of the lifetime risk of melanoma with CDKN2A mutations – ranging from 58 % in Europe to 91 % in Australia. A Genes, Environment, and Melanoma Study (GEMS) report evaluated 3,626 CDKN2A mutation carriers from Australia, Canada, the United States, and Italy and reported a lifetime risk of 14 % by age 50 years, 24 % by age 70 years, and 28 % by age 80 years [13].
Xeroderma pigmentosum (XP) is an autosomal recessive disease in which defective nucleotide excision repair (NER) results in an accumulation of UV-mediated DNA damage in epidermal cells. XP patients have an increased risk of all cutaneous malignancies including melanoma, basal cell carcinoma, and squamous cell carcinoma. Given the rarity (1 in 250,000) and severity (<50 % of patients survive past age 20), XP does not significantly impact melanoma incidence at the population level. Since CDKN2A/CDK4 mutations do not account for all hereditary cases of melanoma coupled with the rarity of XP, it is likely that other as yet unidentified genes are implicated in inherited predisposition to melanoma.
10.3.2.2 Moderate- to Low-Risk Loci
A variety of other genes have been associated with an increased risk of melanoma: MC1R, BRCA2, Rb, MITF (E318K) variant, and 1p22 locus. Melanocortin-1 receptor (MC1R), a G-protein-coupled receptor located on the surface of melanocytes, regulates relative levels of eumelanin and pheomelanin production by melanocytes. As eumelanin protects skin from UV radiation more than pheomelanin does, MC1R allelic variants have divergent risks of melanoma with odds ratios ranging from 1.42 (p.R163Q) to 2.45 (p.I155T) [14]. Patients with mutated BRCA2 and the MITF E318K variant have a twofold increased risk of developing melanoma [15–17]. The increased risk of melanoma in patients with Rb mutations is uncertain, and the melanoma susceptibility locus on chromosome 1p22 has yet to be identified [18, 19].
10.3.3 Risk Factors for Developing Melanoma
As discussed above, the risk of developing melanoma is an aggregate of genetic and environmental factors with UV exposure being the single greatest environmental risk factor. UV exposure is inversely proportional to latitude – being greatest at the equatorial belt (latitude 0°). The link between geography and melanoma incidence was first elucidated by Herbert Lancaster, who evaluated melanoma mortality in populations of European extraction residing at varying latitudes from the equator [20]. Lancaster reported greater mortality among individuals residing in tropical and subtropical Australian states compared to those living in temperate climes; a greater mortality was noted even within countries among subjects living at higher altitudes compared to those living at lower altitudes. These findings have since been replicated in other populations and provide a compelling implication of UV exposure (especially childhood sun UV exposure) in melanoma tumorigenesis [21–23]. Recreational UV exposure (outdoor and indoor tanning) further increases melanoma risk – especially after ten tanning sessions [24]. Recognizing these risks, the Society of Behavioral Medicine has issued a position statement banning indoor tanning for minors that has resulted in legislation to this effect in 11 states [25]. Beyond UV exposure, other risk factors include a personal history of prior melanoma, typical/atypical nevi, and systemic immunosuppression.
10.3.4 Risk Assessment and Screening Strategies
Some of these risk factors have variably been aggregated into several risk prediction models including Australia’s Victorian Melanoma Service (Emily’s Melanoma Risk Calculator) and National Cancer Institute (Melanoma Risk Assessment Tool) [26, 27]. These tools allow practitioners to individualize risk and consider specific interventions such as complete skin surveillance and/or participation in prevention trials in patients deemed high risk.
Survival of melanoma is strongly associated with thickness and level of invasion through the skin, and early detection appears to identify groups of patients for whom cure may be achieved with surgical excision alone. Despite compelling data regarding risk stratification and high lifetime risk of invasive disease in high-risk individuals, the US Preventive Services Task Force (USPSTF) and Cancer Council Australia have not adopted recommendations for population screening strategies to date, citing insufficient evidence that screening reduces mortality. Recent data, however, suggests otherwise. Investigators conducted an observational study at the Lawrence Livermore National Laboratory that incorporated awareness and targeted screening over a 26-year period (1969–1996). Albeit neither randomized nor controlled, targeted screening was associated with declining incidence of melanomas >0.75 mm, and no melanoma-specific deaths were noted during the screening period [28]. Germany has funded a screening study that was initially pursued in the State of Schleswig-Holstein, in which general practitioners and dermatologists were trained in an 8 h session to perform a standardized whole-body examination. Between July 2003 and June 2004, 360,288 men and women aged >20 were screened by general practitioners or dermatologists trained in this fashion. Age-standardized melanoma mortality declined by 47 % (men) and 49 % (women) by 2008/2009 compared to similar prescreening statistics [29]. These results have prompted similar trials in several large integrated hospital systems in the United States – reports from which are eagerly awaited.
Consensus society guidelines recommend genetic counseling for patients with a suggestive family pedigree to discuss the risks and benefits of genetic testing. CDKN2A testing can be considered in several instances:
Melanoma diagnoses among multiple first-degree family members. An Australian series of 131 probands with a family history of melanoma reported a 15.1 % incidence of CDKN2A mutations when in families with three or more cases of melanoma among first-degree relatives [30].
Multiple primary melanomas (MPM). Patients with two or more primaries are more likely to have CDKN2A mutations than patients with a single primary melanoma. GEMS Study Group report suggested a CDKN2A mutation rate of 3 % in patients with MPM, although other reports have suggested that this might be higher especially in patients with greater than two melanomas [13, 31].
Melanomas in association with other primary malignancies. Besides melanoma, CDKN2A mutations are associated with the development of other malignancies with lifetime relative risk estimates ranging from 40.4 (Wilms tumor), 7.4 (pancreatic cancer), 1.9 (colorectal cancer, brain cancer), to 1.4 (lung cancer) [32].
Patients with a defined mutation such as CDKN2A/CDK4 are recommended to undergo close surveillance and receive education regarding primary prevention with sunscreen and avoiding unnecessary UV exposure. Given the attendant risk of pancreatic cancer, pancreatic cancer surveillance is recommended in patients with CDKN2A mutations and/or family history of CDKN2A-associated melanoma/pancreatic cancer. In patients in whom no germline gene mutation is detected or in those who have a moderate- to low-risk locus, experts advocate education regarding risk reduction and recommend routine surveillance. Although the data from the Lawrence Livermore National Laboratory and Schleswig-Holstein studies are highly suggestive, there is a need for more rigorous or randomized data to allow the USPSTF to develop recommendations for universal screening of adults at this time, and the incidence of melanoma below 20–35 may make recommendations for the AYA group more difficult even then.
10.4 Clinical Presentation
Although certain predisposing factors, such as a familial history of melanoma, multiple dysplastic nevi [33], large congenital nevi [34], and an impaired immune system, increase one’s risk of developing melanoma earlier in life – for the majority of AYA with melanoma – no preexisting factor can be found. As in adults, those AYA with fair skin, blue or green eyes, red or blonde hair, and propensity to sunburn (Fitzpatrick skin phenotypes I–II) are at a higher risk for melanoma [35].
Because of a low index of suspicion, the diagnosis of melanoma may sometimes be delayed among younger patients [36]. The diagnosis of melanocytic tumors in AYA is further complicated because of the higher likelihood of lesions with spitzoid morphology to occur in this age group. Spitzoid lesions, which can be benign (e.g., Spitz nevus), borderline (atypical Spitz tumor), or frankly malignant (spitzoid melanoma), more commonly affect younger individuals and sometimes can create diagnostic uncertainty regarding their proper classification [37].
The clinical presentation of melanoma in AYA is similar to those in adults. The most common complaints in AYA with early lesions are a change in the size and color of a pigmented lesion. Other signs and symptoms may be bleeding, itching, pain, or ulceration that represent manifestations of later disease. Spitzoid melanoma presents as a papule, nodule, or less often a plaque that may often be amelanotic [38].
10.5 Staging
In cutaneous melanoma, four factors (primary tumor thickness, ulceration, mitotic rate, and lymph node tumor burden) independently predict the risk of locoregional relapse and mortality. These factors are delineated in the revised 2009 classification on the staging and prognosis of cutaneous melanoma copublished by the American Joint Committee on Cancer (AJCC) and the International Union Against Cancer (UICC) [39].
Primary tumor thickness (measured in mm) is the single most powerful factor predictive of 5- and 10-year survival rates, which decline commensurate with the thickness of the tumor. Tumor ulceration is an adverse factor associated with poorer survival and factored into the a/b substages of the “T” category; ulceration of any given “T” substage (designated “b”) has a risk of relapse and/or death that approximates the next higher “T” substage (T1, T2, T3, and T4).
Mitotic rate (number of mitoses per square millimeter observed) in the primary tumor is an adverse prognostic factor that has been recognized for some time, but was factored into the AJCC staging system only in the last edition. Mitotic activity subclassifies lesions into non-mitogenic potential (0 mitoses/mm2), low mitogenic potential (1–2 mitoses/mm2), and high mitogenic potential (≥3 mitoses/mm2). In the 2009 AJCC/UICC staging manual, high mitotic rate (as defined as ≥1 mitoses/mm2) was incorporated into the substaging of T1 lesions only – where this finding was used to assign patients to T1b, much as ulceration does. Recent evidence suggests that mitotic rate is a reliable adverse prognostic factor and may have a greater value than ulceration in early melanomas [40]. The grading of a lesion for mitotic rate and the role of mitotic activity in lesions over T1 micro-stage will be a factor to have clarified in the 8th edition of the AJCC/UICC staging manual, which is being developed over the next year.
The risk of lymph node involvement increases with tumor thickness by approximately 2–5 % for Breslow’s depth ≤1.00 mm and reaches 34 % for T4 lesions. Lymph node involvement is staged based on number (N1, one node; N2, two to three nodes; and N3, four or more nodes, the presence of tumor satellites surrounding the primary, or in-transit disease in the proximal lymphatics) and is subdivided according to the extent (a, micrometastases; b, macro-metastases). Unlike micrometastases, which are clinically non-palpable and diagnosed only after pathological evaluation of the wide excision and sentinel lymph node biopsy (with a cutoff of 0.1 mm to be considered positive), macro-metastases refer to clinically palpable regional lymph nodes. Sub-0.1 mm involvement (“submicrometastases”) after sentinel lymph node evaluation is considered by some to be negative as such patients in some series have had similar rates of relapse and mortality as patients with sentinel lymph node-negative disease [41]. However, the long interval that is required to reach maturity and definitive conclusions qualifies the assessment of these questions, and the AJCC Subcommittee for the 7th edition chose to delineate the presence of even a single tumor cell as positive.
In evaluating the systemic burden of disease, the site(s) of distant metastases has greater prognostic import than the number of metastatic sites. Patients with distant skin, subcutaneous, and/or lymph node metastases have what has been classified as M1a disease – with improved 1-year survival rates (62 %) compared to patients with pulmonary (M1b, 53 %) and extrapulmonary visceral metastases (M1c, 33 %). Patients with elevated serum lactate dehydrogenase (LDH) enzyme levels have poor outcomes that are similar to patients with non-lung visceral metastases and are therefore staged M1c.
10.6 Surgical Therapy
10.6.1 Resection
In the absence of guidelines regarding the surgical resection of melanomas in pediatric and AYA patients, management has defaulted to standards used to treat adults. Ideally, suspicious-appearing pigmented cutaneous lesions should be biopsied: either with a punch biopsy of the lesion including its deepest portion or with an excisional biopsy that includes a 1–2 mm rim of normal skin. Initial biopsies should be oriented parallel to long axis for extremity lesions or parallel to Langer’s lines of stress for truncal lesions thinking ahead to the definitive wide local excision (WLE) required should diagnosis of melanoma be confirmed. Such forethought facilitates subsequent surgical closure and minimizes skin grafting requirements. Deep punch or excisional biopsies are recommended over superficial shave biopsies for several reasons:
Shave biopsies tend to underestimate or incompletely assess Breslow’s thickness.
Shave biopsies tend to leave residual tumor at the radial and/or deep margins.
Post-biopsy scarring can obscure identification of residual melanoma in subsequent resection specimens.
Once pathological confirmation is obtained, definitive surgical management involves WLE with consideration of regional lymph node staging for a subset of deeper tumors. Definitive WLE involves an excision down to deep fascia with a width of peri-lesional normal tissue ranging from 1 cm (<2 mm T1–T2 melanomas) to 2 cm (>2.01 mm T3–T4 melanomas) [42]. Although some have argued for larger (3 cm) margins for patients with thick (T4) melanomas, no correlation between greater margin width and lower local/regional recurrence rates has been demonstrated [43, 44].
There is an absence of high-quality data from randomized trials to guide the management of melanoma in situ (MIS) lesions. A 5 mm margin was recommended by a National Institutes of Health (NIH) consensus statement in 1992 [45] and the WHO prior to that.
Mohs microsurgery (MMS) has gained popularity, especially in the management of lesions in locations where primary closure is hard to achieve (face, head and neck, distal upper extremity) and for large superficial lesions (lentigo maligna). Although retrospective data suggests that MMS has good outcomes, it has not been prospectively compared to WLE [46]. Further, although suitable for local control, MMS does not obviate the need for regional staging with sentinel lymph node (SLN) biopsy.
10.6.2 Lymph Node Staging
Regional lymph nodes are the most common (and often initial) site of melanoma metastases. For decades, surgical oncologists debated the rationale of removing clinically negative lymph nodes – essentially aiming to identify a group of patients with concurrent high risk of regional metastases but low risk of distant metastases. In 1979, Balch CM and colleagues reported on the 3-year risk of regional metastases with resected primary melanoma: 0 % (<0.76 mm), 25 % (0.6–1.50 mm), 51 % (1.50–3.99 mm), and 62 % (>4 mm) [47]. These results led many surgeons to advocate for elective lymph node dissections (LND) in all patients – with significant attendant morbidity as only 15–20 % of patients have regional lymph node involvement. The Intergroup Melanoma Surgical Trial was a randomized study of upfront elective LND versus observation in intermediate-thickness melanomas (1.0–4.0 mm). Although overall survival at 10 years was not significantly different in either group, patients with ulcerated and/or T1–T2 (1.0–2.0 mm) melanomas especially benefited from upfront LND – suggesting that these patients had a substantial risk of regional disease with no occult metastases [48]. In 1992, Donald Morton and Alistair Cochran demonstrated that sentinel lymph nodes (SLN) could be reliably mapped using a minimally invasive technique that utilized colloid dye and lymphoscintigraphy with intradermal injection of 99mTc-sulfur colloid or 99mTc-human serum albumin [49].
SLN assessment is reliable and of prognostic value to the patient and managing physicians. False-negative rates are low (<10 %), although greater tumor depth and non-extremity primary sites are associated with higher false-negative rates [50]. SLN status is highly prognostic – SLN negativity is associated with significant increases in disease-specific survival [51]. SLN biopsy was compared to observation in 2001 patients with intermediate (defined as 1.2–3.5 mm) and thick (defined as >3.5 mm) melanomas in the Multicenter Selective Lymphadenectomy Trial (MSLT-I). The initial report of this trial consisted of the 5-year outcomes in the intermediate cohort. Compared to observation, patients who underwent SLNB had improved time to relapse, but melanoma-specific survival was similar in both groups. Patients with positive SLN treated with immediate lymphadenectomy had an improved survival although this was a subset analysis and not the primary endpoint [52]. Recently, final results of the MSLT-I consisting of 10-year outcomes in the intermediate-thickness melanomas were reported [53]. Previously noted survival benefits in intermediate-thickness melanoma with positive SLN who underwent immediate LND compared to patients on observation treated with LND at time of clinical recurrence were upheld. Notably, thick melanomas that underwent LND guided by SLN biopsy had improved 10-year disease-free survival, but not melanoma-specific survival – suggesting that thick melanomas had a higher risk of occult distant metastases than intermediate melanomas.
The risk of identifying a positive sentinel node is directly correlated with the thickness of primary melanoma. Even thin melanomas (≤1.50 mm) carry a substantial risk of SLN involvement – ranging from 2.9 % (≤1.00 mm) to 7.1 % (1.01–1.50 mm) [54]. Bleicher et al. observed a higher incidence of SLN involvement in patients aged <44 years compared to older patients [54]. Ultimately, this underscores the need to consider SLN evaluation in all patients with Breslow’s depth >1.00 mm and in selected candidates with thinner melanomas who have other adverse prognostic factors or incomplete biopsies.
Unlike cutaneous melanomas and pediatric “conventional” melanomas, in which SLN involvement is commensurate to risk of distant metastases and eventual mortality, Spitzoid melanomas in the AYA have a high incidence of nodal involvement but a generally low risk of subsequent systemic spread. Lallas et al. conducted a systematic review of 24 trials that evaluated SLN biopsy in 541 patients with atypical Spitz tumors [55]. Two hundred and thirty-eight patients underwent wide excision alone, while 303 patients had SLN evaluation in addition to wide excision; SLN was negative in 184 (61 %) patients and positive in 119 (31 %) patients. Of the latter, 97 (97/119, 82 %) underwent completion LN dissection, and 18 (18/97, 19 %) had 1 or more positive LN. Five patients had regional recurrence, while six patients died from metastatic disease – of whom one fifth and one sixth, respectively, had a positive SLN but no subsequent completion LN dissection. Ninety nine percent of all patients were alive at a median follow-up of 59 months. Separately, other authors have reported that the age at diagnosis may affect the likelihood of SLN involvement – with a lower risk of SLN involvement in patients younger than 10 years of age [56]. These studies illustrate the unique biology of spitzoid melanomas, which have a high incidence of nodal disease but subsequent lack of dissemination beyond LN, and underscore the controversial nature of SLN evaluation in this disease.
10.7 Nonsurgical Therapy
10.7.1 Adjuvant Therapy in High-Risk Resected Disease
10.7.1.1 Adjuvant High-Dose IFN (HDI)
Early reports of the antitumor efficacy of cytokines (IFN-α, IL-2, IL-7, and IL-21) in a variety of tumor types resulted in a series of studies that evaluated IFN-α in advanced melanoma. Results were promising: occasional durable responses and complete responses, albeit of low frequency. Attempting to forestall the epidemic of advanced disease, investigators evaluated IFN-α in a plethora of adjuvant trials. These studies tested multiple subtypes (IFN-α2a, IFN-α2b, and IFN-α2c) at various dosages (low dose, ≤3 MU/dose; intermediate dose, 5–10 MU/dose; and high dose, ≥10 MU/dose) and schedules (induction, maintenance, and induction/maintenance) in an effort to define the most efficacious subtype, dose, and schedule and are summarized in Table 10.1 [57–75].
Table 10.1
Phase III studies of adjuvant IFN-α in high-risk resected melanoma
Study reference | Patients eligible for analysis | Stage | IFN type | Dose and schedule – treatment arm | Median follow-up at reporting (years) | DFS/RFS | OS | % node positive |
---|---|---|---|---|---|---|---|---|
High dose | ||||||||
NCCTG 83-7052 [57] | 262 | II–III (T2–4N0M0 or TanyN+M0) | IFN-α2a vs. observation | IM 20 MU/m2 3 days a week for 4 months | 6.1 | HR: 1.20 (HDI vs. observation) (NS) | HR: 1.11 (HDI vs. observation) (NS) | 61 |
ECOG E1684 [58] | 287 | II–III (T4N0M0 or TanyN+M0) | IFN-α2b vs. observation | Induction: IV 20 MU/m2 5 days a week for 4 weeks | 12.6 | HR: 1.38 (HDI vs. observation) (S) | HR: 1.22 (HDI vs. observation) (S at 6.9 years but NS at 12.6 years) | 89 |
Maintenance: SC 10 MU/m2 3 days a week for 48 weeks | ||||||||
ECOG E1690 [59] | 642 | IFN-α2b – high dose vs. low dose vs. observation | High dose: | 4.3 | HR: 1.28 (HDI vs. observation) (S) | HR: 1.0 (HDI vs. observation) (NS) | 74 | |
Induction: IV 20 MU/m2 5 days a week for 4 weeks | ||||||||
Maintenance: SC 10 MU/m2 3 days a week for 48 weeks | ||||||||
Low dose: SC 3 MU/m2 2 days a week for 2 years | ||||||||
1.19 (LDI vs. observation) (NS) | 1.04 (LDI vs. observation) (NS) | |||||||
RFS: 44 % (HDI) vs. 40 % (LDI) vs. 35 % (observation) | OS: 52 % (HDI) vs. 53 % (LDI) vs. 55 % (observation) | |||||||
ECOG E1694 [60] | 774 | IFN-α2b vs. GMK vaccine | Induction: IV 20 MU/m2 5 days a week for 4 weeks | 2.1 | HR: 1.49 (HDI vs. GMK) (S) | HR: 1.38 (HDI vs. GMK) (S) | 77 | |
Maintenance: SC 10 MU/m2 3 days a week for 48 weeks | ||||||||
RFS: 25 % (HDI) vs. 39 % (GMK) | OS: 78 % (HDI) vs. 73 % (GMK) | |||||||
ECOG E2696 [61] | 107 | II–IV | GMK vaccination with concurrent HDI (arm A) vs. GMK vaccination with HDI beginning D28 (arm B) vs. GMK vaccination alone (arm C) | Induction: IV 20 MU/m2 5 days a week for 4 weeks | 2.4 | HR: 1.75 (C vs. A) (S) | HR: not reported | Not reported |
Maintenance: SC 10 MU/m2 3 days a week for 48 weeks | ||||||||
GMK vaccination: GM2-KLH/QS-21 on D1, 8, 15, 22 then weeks 12, 24, 36 | ||||||||
OS: not reached (A, B, or C) | ||||||||
1.96 (C vs. B) (S) | ||||||||
RFS: not reached (A) vs. 30.72 months (B) vs. 14.85 months (C) | ||||||||
Hellenic He13A/98 [62] | 364 | IIB–C/III (T3/4N0M0 or TanyN+M0) | Modified IFN-α2b induction only (arm A) vs. modified IFN-α2b induction and maintenance (arm B) | Modified induction: IV 15 MU/m2 5 days a week for 4 weeks | 5.3 | Median RFS: 24.1 months (arm A) vs. 27.9 (arm B) (NS, primary non-inferiority endpoint met) | Median OS: 64.4 months (arm A) vs. 65.3 (arm B) (NS) | 58 |
Non-inferiority design | ||||||||
Modified maintenance: SC 10 MU 3 days a week for 48 weeks | ||||||||
Italian Melanoma Intergroup [63] | 330 | III (TanyN1–3 M0) | Intensified IFN-α2b (IHDI) every other month vs. IFN-α2b for 1 year | IHDI: IV 20 MU/m2 5 days a week for 4 weeks every other month for 4 cycles | 5.0 | Median RFS: 47.9 months (IHDI) vs. 35.6 months (HDI) (NS) 5 year RFS: 45.8 % (IHDI) vs. 44.3 % (HDI) | Median OS: 88.7 months 5 year OS: 60.1 % (IHDI) vs. 82.6 % (HDI) (NS) | 100 |
Standard HDI: IV 20 MU/m2 5 days a week for 4 weeks → then → SC 10 MU/m2 3 days a week for 48 weeks | ||||||||
E1697 [64] | 1,150 (1,420 planned enrollment) | IIB–C/III (T2–4N0M0 or TanyN1a/2aM0) | Induction HDI vs. observation | Induction only: IV 20 MU/m2 5 days a week for 4 weeks | Not reported | Median RFS: 6.8 years (HDI induction) vs. 7.3 years (observation) (NS) | 5 year OS: 82 % (HDI induction) vs. 85 % (observation) (NS) | 19 |
Intermediate dose | ||||||||
EORTC 18952 [65] | 1,388 | II–III (T4N0M0 or TanyN+M0) | IFN-α2b for 1 year vs. 2 years vs. observation | Induction: IV 10 MU 5 days a week for 4 weeks | 4.7 | DMFI: | DMFS: | 74 |
HR: 0.93 (13 month vs. observation) (NS) 0.83 (25 month vs. observation) (S) | HR: 0.95 (13 month vs. observation) (NS) 0.85 (25 month vs. observation) (NS) | |||||||
Maintenance: SC 10 MU 3 days a week for 1 year or SC 5 MU 3 days a week for 2 years | ||||||||
EORTC 18991 [66] | 1,256 | III (TanyN+M0) | Peg-IFN vs. observation | Induction: SC 6 μg/kg/week for 8 weeks | 7.6 | 34.8 months (IFN) vs. 25.6 months (observation); S | Not reported | 100 |
Maintenance: SC 3 μg/kg/week for 5 years | ||||||||
Nordic IFN [67] | 855 | IIB-IIIB (T4N0M0 or TanyN1–2 M0) | IFN-α2b for 1 year vs. 2 years vs. observation | SC 10 MU 5 days a week for 4 weeks then SC 10 MU 3 days a week for 1 year (B) versus | 6.0 | 23.2 months (A) vs. 37.8 months (B) vs. 28.6 months (C) IFN vs. observation and IFN 1 year vs. observation (S); IFN 2 year vs. observation (NS) | 56.1 months (A) vs. 72.1 months (B) vs. 64.3 months (C) (NS) | 81 |
SC 10 MU 5 days a week for 4 weeks then SC 10 MU 3 days a week for 2 years (C) versus observation (A) | ||||||||
Low dose | ||||||||
Austrian Melanoma Cooperative Group (AMCG) [68] | 311 | II (T2-4N0M0) | IFN-α2a vs. observation | SC 3 MU 7 days a week for 3 weeks, then SC 3 MU 3 days a week for 1 year | 3.4 | RFS/DMFS not reported | Not reported | 0 |
Rate of relapse: (24.0 % LDI vs. 36.3 % observation) | ||||||||
French Melanoma Cooperative Group (FCGM) [69] | 499 | II (T2–4N0M0) | IFN-α2a vs. observation | SC 3 MU 3 days a week for 18 months
![]() Stay updated, free articles. Join our Telegram channel![]() Full access? Get Clinical Tree![]() ![]() ![]() |