Long-Term Survivorship: Late Effects
Noreen M. Aziz
Background and Significance
With continued advances in strategies to detect cancer early and treat it effectively along with the aging of the population, the number of individuals living years beyond a cancer diagnosis can be expected to continue to increase. Statistical trends show that, in the absence of other competing causes of death, 64% of adults diagnosed with cancer today can expect to be alive in 5 years (1, 2, 3, 4). Relative 5-year survival rates for those diagnosed as children (age <19 years) are even higher, with almost 79% of childhood cancer survivors estimated to be alive at 5 years and 75% at 10 years (5).
Survival from cancer has seen dramatic improvements over the past three decades, mainly as a result of advances in early detection, therapeutic strategies, and the widespread use of combined modality therapy (surgery, chemotherapy, and radiotherapy) (6, 7, 8, 9, 10). Medical and sociocultural factors such as psychosocial and behavioral interventions, active screening behaviors, and healthier lifestyles may also play an integral role in the length and quality of that survival (11).
Although beneficial and often lifesaving against the diagnosed malignancy, most therapeutic modalities for cancer are associated with a spectrum of late complications ranging from minor and treatable to serious or, occasionally, potentially lethal (2, 6, 12, 13, 14, 15). Although living for extended periods of time beyond their initial diagnosis, many cancer survivors often face various chronic and late physical and psychosocial sequelae of their disease or its treatment. Additionally, as the number of survivors and their length of survival expand, long-term health issues specific to cancer survival are also fast emerging as a public health concern. Questions of particular importance to cancer survivors include surveillance for the adverse sequelae, or late and long-term effects, of treatment; the development of new (second) cancers; and recurrence of their original cancer. One fourth of late deaths occurring among survivors of childhood cancer during the extended survivorship period, when the chances of primary disease recurrence are negligible, can be attributed to a treatment-related effect such as a second cancer or cardiac dysfunction (16). The most frequently observed medical sequelae among pediatric cancer survivors include endocrine complications, growth hormone deficiency, primary hypothyroidism, and primary ovarian failure. Also included within the rubric of late effects are second cancers arising as a result of genetic predisposition (e.g., familial cancer syndromes) or the mutagenic effects of therapy. These factors may act independently or synergistically. Synergistic effects of mutagenic agents such as cigarette smoke or toxins such as alcohol are largely unknown (2, 6, 12).
Therefore, there is today a greater recognition of symptoms that persist after the completion of treatment and which arise years after primary therapy. Both acute organ toxicities such as radiation pneumonitis and chronic toxicities such as congestive cardiac failure, neurocognitive deficits, infertility, and second malignancies are being described as the price of cure or prolonged survival (2, 6, 12). The study of late effects, originally within the realm of pediatric cancer, is now germane to cancer survivors at all ages because concerns may continue to surface throughout the life cycle (2, 6). These concerns underscore the need to follow-up and screen survivors of cancer for toxicities such as those mentioned and also to develop and provide effective interventions that carry the potential to prevent or ameliorate adverse outcomes.
The goal of survivorship research is to focus on the health and life of a person with a history of cancer beyond the acute diagnosis and treatment phase. Survivorship research seeks to examine the causes of, and to prevent and control the adverse effects associated with, cancer and its treatment, and to optimize the physiologic, psychosocial, and functional outcomes for cancer survivors and their families. A hallmark of survivorship research is its emphasis on understanding the integration/interaction of multidisciplinary domains.
This chapter presents definitional issues relevant to cancer survivorship; examines late effects of cancer treatment among survivors of pediatric and adult cancer; and articulates gaps in knowledge and emerging research priorities in cancer survivorship research relevant to late effects of cancer treatment. It draws heavily from pediatric cancer survivorship research because a paucity of data continues to exist for medical late effects of treatment for survivors of cancer diagnosed as adults. Research on late effects of cancer treatment began in the realm of pediatric cancer and continues to yield important insights for the impact of cancer therapies among adults.
Definitional Issues
Fitzhugh Mullan, a physician diagnosed with and treated for cancer himself, first described cancer survivorship as a concept (17). Definitional issues for cancer survivorship encompass three related aspects (2, 6).:
Who is a cancer survivor? Philosophically, anyone who has been diagnosed with cancer is a survivor, from the
time of diagnosis to the end of life.* Caregivers and family members are also included within this definition as secondary survivors.
What is cancer survivorship? Mullan described the survivorship experience as similar to the seasons of the year. Mullan recognized three seasons or phases of survival: acute (extending from diagnosis to the completion of initial treatment, encompassing issues dominated by treatment and its side effects); extended (beginning with the completion of initial treatment for the primary disease, remission of disease, or both, dominated by watchful waiting, regular follow-up examinations, and, perhaps, intermittent therapy); and permanent survival (not a single moment; evolves from extended disease-free survival when the likelihood of recurrence is sufficiently low). An understanding of these phases of survival is important for facilitating an optimal transition into and management of survivorship.
What is cancer survivorship research? Cancer survivorship research seeks to identify, examine, prevent, and control adverse cancer diagnosis and treatment-related outcomes (such as late effects of treatment, second cancers, and quality of life); to provide a knowledge base regarding optimal follow-up care and surveillance of cancer survivors; and to optimize health after cancer treatment (2, 6).
Other important definitions include those for long-term cancer survivorship and late versus long-term effects of cancer treatment. Generally, long-term cancer survivors are defined as those individuals who are 5 or more years beyond the diagnosis of their primary disease and embody the concept of permanent survival described by Mullan. Late effects refer specifically to unrecognized toxicities that are absent or subclinical at the end of therapy and become manifest later with the unmasking of hitherto unseen injury caused by any of the following factors: developmental processes, the failure of compensatory mechanisms with the passage of time, or organ senescence. Long-term effects refer to any side effects or complications of treatment for which a patient with cancer must compensate; also known as persistent effects, they begin during treatment and continue beyond the end of treatment. Late effects, in contrast, appear months to years after the completion of treatment. Some researchers classify cognitive problems, fatigue, lymphedema, and peripheral neuropathy as long-term effects whereas others classify them as late effects (18, 19, 20, 21). Chemotherapeutic drugs for which late effects have been reported most frequently include adriamycin, bleomycin, vincristine, methotrexate, cytoxan, and many others (Table 71.1) (22, 23, 24, 25, 26, 27, 28, 29, 30, 31, 32, 33, 34, 35, 36, 37, 38, 39, 40, 41, 42, 43, 44, 45, 46).
Table 71.1 Possible Late Effects of Radiotherapy and Chemotherapy | ||||||||||||||||||||||||||||||||||||||||||||||||||||||||||||||||||||
---|---|---|---|---|---|---|---|---|---|---|---|---|---|---|---|---|---|---|---|---|---|---|---|---|---|---|---|---|---|---|---|---|---|---|---|---|---|---|---|---|---|---|---|---|---|---|---|---|---|---|---|---|---|---|---|---|---|---|---|---|---|---|---|---|---|---|---|---|
|
This chapter focuses largely on the physiologic or medical long-term and late effects of cancer treatment. Physiologic sequelae of cancer treatment can also be further classified as follows:
System specific (e.g., organ damage, failure, or premature aging, immunosuppression, or issues related to compromised immune systems, and endocrine damage)
Second malignant neoplasms (such as an increased risk of recurrent malignancy, increased risk of a certain cancer associated with the primary malignancy, and/or increased risk of secondary malignancies associated with cytotoxic or radiologic cancer therapies (this topic is not covered in detail in this chapter as it is reviewed comprehensively elsewhere in this book) and
Functional changes such as lymphedema, incontinence, pain syndromes, neuropathies, fatigue; cosmetic changes such as amputations, ostomies, and skin/hair alterations; and comorbidities such as osteoporosis, arthritis, and hypertension
Review of Late and Long-Term Effects by Organ System or Tissues Affected†
System-Specific Physiologic Sequelae‡
Cardiac Sequelae
The heart may be damaged by both therapeutic irradiation and chemotherapeutic agents commonly used in the treatment for cancer. Several types of damage have been reported, including pericardial, myocardial, and vascular. Cardiac damage is most pronounced after treatment with the anthracycline drugs doxorubicin and daunorubicin, used widely in the treatment of most childhood cancers and adjuvant chemotherapy for breast and many other adult cancers. An additive effect has also been reported when anthracyclines are used in conjunction with cyclophosphamide and radiation therapy. Anthracyclines cause myocardial cell death, leading to a diminished number of myocytes and compensatory hypertrophy of residual myocytes (47). Major clinical manifestations include reduced cardiac function, arrhythmia, and heart failure. Chronic cardiotoxicity usually manifests itself as cardiomyopathy, pericarditis, and congestive heart failure.
Cardiac injury that becomes clinically manifest during or shortly after completion of chemotherapy may progress, stabilize, or improve after the first year of treatment. This improvement may either be of a transient nature or last for a considerable length of time. There is also evidence of a continuum of injury that will manifest itself throughout the lives of these patients (48). From a risk factor perspective, patients who exhibit reduced cardiac function within 6 months of completing chemotherapy are at increased risk for the development of late cardiac failure (49). However, a significant incidence of late cardiac decompensation manifested by cardiac failure or lethal arrhythmia occurring 10–20 years after the administration of these drugs has also been reported (50).
In a recent study of Hodgkin’s disease (HD) survivors, investigators reported finding cardiac abnormalities in most of the participants (51). This is an important finding especially because the sample consisted of individuals who did not manifest symptomatic heart disease at screening and described their health as “good.” Manifestations of cardiac abnormalities include the following:
Restrictive cardiomyopathy (suggested by reduced average left ventricular dimension and mass without increased left ventricular wall thickness)
Significant valvular defects
Conduction defects
Complete heart block
Autonomic dysfunction (suggested by a monotonous heart rate in 57%)
Persistent tachycardia and
Blunted hemodynamic responses to exercise
The peak oxygen uptake (VO2) during exercise, a predictor of mortality in heart failure, was significantly reduced
(<20 mL/kg/m2) in 30% of survivors and was correlated with increasing fatigue, increasing shortness of breath, and a decreasing physical component score on the SF-36. Given the presence of these clinically significant cardiovascular abnormalities, investigators recommend serial, comprehensive cardiac screening of HD survivors who fit the profile of having received mediastinal irradiation at a young age.
(<20 mL/kg/m2) in 30% of survivors and was correlated with increasing fatigue, increasing shortness of breath, and a decreasing physical component score on the SF-36. Given the presence of these clinically significant cardiovascular abnormalities, investigators recommend serial, comprehensive cardiac screening of HD survivors who fit the profile of having received mediastinal irradiation at a young age.
Congestive cardiomyopathy is directly related to the total dose of the agent administered; the higher the dose, the greater the chance of cardiotoxicity. Subclinical abnormalities have also been noted at lower doses. The anthracyclines doxorubicin and daunorubicin are well-known causes of cardiomyopathy that can occur many years after completion of therapy. The incidence of anthracycline-induced cardiomyopathy, which is dose dependent, may exceed 30% among patients receiving cumulative doses in excess of 600 mg per m2. A cumulative dose of anthracyclines >300 mg per m2 has been associated with an 11-fold-increased risk of clinical heart failure, compared with a cumulative dose of <300 mg per m2, the estimated risk of clinical heart failure increasing with time from exposure and approaching 5% after 15 years.
A reduced incidence and severity of cardiac abnormalities was reported in a study of 120 long-term survivors of acute lymphoblastic leukemia (ALL) who had been treated with lower anthracycline doses (90–270 mg per m2), compared with previous reports in which subjects had received moderate anthracycline doses (300–550 mg per m2) (52, 53). Twenty-three percent of the patients were found to have cardiac abnormalities, 21% had increased end-systolic stress, and only 2% had reduced contractility. The cumulative anthracycline dose within the 90–270 mg per m2 range did not relate to cardiac abnormalities. The authors concluded that there may be no safe anthracycline dose to completely avoid late cardiotoxicity. A recent review of 30 published studies in childhood cancer survivors found that the frequency of clinically detected anthracycline cardiac heart failure ranged from 0 to 16% (54). In an analysis of reported studies, the type of anthracycline (e.g., doxorubicin) and the maximum dose given in a 1-week period (e.g., >45 mg per m2) was found to explain a large portion of the variation in the reported frequency of anthracycline-induced heart failure.
Cyclophosphamide has been associated with the development of congestive cardiomyopathy, especially when administered at the high doses used in transplant regimens. Cardiac toxicity may occur at lower doses when mediastinal radiation is combined with the chemotherapeutic drugs mentioned above. Late onset of congestive heart failure has been reported during pregnancy, rapid growth, or after the initiation of vigorous exercise programs in adults previously treated for cancer during childhood or young adulthood as a result of
increased afterload and the impact of the additional stress of such events on marginal cardiac reserves. Initial improvement in cardiac function after completion of therapy appears to result, at least in part, from compensatory changes. Compensation may diminish in the presence of stressors such as those mentioned earlier and myocardial depressants such as alcohol.
increased afterload and the impact of the additional stress of such events on marginal cardiac reserves. Initial improvement in cardiac function after completion of therapy appears to result, at least in part, from compensatory changes. Compensation may diminish in the presence of stressors such as those mentioned earlier and myocardial depressants such as alcohol.
The incidence of subclinical anthracycline myocardial damage has been the subject of considerable interest. Steinherz et al. found 23% of 201 patients who had received a median cumulative dose of doxorubicin of 450 mg per m2 had echocardiographic abnormalities at a median of 7 years after therapy (55). In a group of survivors of childhood cancer received a median doxorubicin dose of 334 mg per m2, it was found that progressive elevation of afterload or depression of left ventricular contractility was present in approximately 75% of patients (47). A recent review of the literature on subclinical cardiotoxicity among children treated with an anthracycline found that the reported frequency of subclinical cardiotoxicity varied considerably across the 25 studies reviewed (frequency ranging from 0 to 57%) (56). Because of marked differences in the definition of outcomes for subclinical cardiotoxicity and the heterogeneity of the patient populations investigated, it is difficult to accurately evaluate the potential long-term outcomes within anthracycline-exposed patient populations or the potential impact of the subclinical findings.
Effects of radiation on the heart may be profound, and include valvular damage, pericardial thickening, and ischemic heart disease. Patients with radiation-related cardiac damage have a markedly increased relative risk (RR) of both angina and myocardial infarction [RR, 2.56] years after mediastinal radiation for HD in adult patients, whereas the risk of cardiac death is 3.1 (57). This risk was greatest among patients receiving >30 Gy of mantle irradiation and those treated before 20–21 years of age. Blocking the heart reduced the risk of cardiac death due to causes other than myocardial infarction (58).
In general, among anthracycline-exposed patients, the risk of cardiotoxicity can be increased by mediastinal radiation (59), uncontrolled hypertension (60, 61, underlying cardiac abnormalities (62), exposure to nonanthracycline chemotherapeutic agents (especially cyclophosphamide, dactinomycin, mitomycin C, dacarbazine, vincristine, bleomycin, and methotrexate) (63, 64, female gender (65), younger age (66), and electrolyte imbalances such as hypokalaemia and hypomagnesaemia (67). Previous reports have suggested that doxorubicin-induced cardiotoxicity can be prevented by continuous infusion of the drug (68). However, Lipshultz et al. compared cardiac outcomes in children receiving either bolus or continuous infusion of doxorubicin, and reported that continuous doxorubicin infusion over 48 hours for childhood leukemia did not offer a cardioprotective advantage over bolus infusion (69). Both regimens were associated with progressive subclinical cardiotoxicity, therefore suggesting that there is no benefit from continuous infusion of anthracyclines.
Chronic cardiotoxicity associated with radiation alone most commonly involves pericardial effusions or constrictive pericarditis, sometimes in association with pancarditis. Although a dose of 40 Gy of total heart irradiation appears to be the usual threshold, pericarditis has been reported after as little as 15 Gy, even in the absence of radiomimetic chemotherapy (70, 71). Symptomatic pericarditis, which usually develops 10–30 years after irradiation, is found in 2–10% of patients (72). Subclinical pericardial and myocardial damage, as well as valvular thickening, may be common in this population (73, 74). Coronary artery disease has been reported after radiation to the mediastinum, although mortality rates have not been significantly higher in patients who receive mediastinal radiation than in the general population (58).
Given the known acute and long-term cardiac complications of therapy, prevention of cardiotoxicity is a focus of active investigation. Several attempts have been made to minimize the cardiotoxicity of anthracyclines, such as the use of liposomal-formulated anthracyclines, less-cardiotoxic analogs, and the additional administration of cardioprotective agents. The advantages of these approaches are still controversial, but there are ongoing clinical trials to evaluate the long-term effects. Certain analogs of doxorubicin and daunorubicin, with decreased cardiotoxicity but equivalent antitumor activity, are being explored. Agents such as dexrazoxane, which are able to remove iron from anthracyclines, have been investigated as cardioprotectants. Clinical trials of dexrazoxane have been conducted in children, with encouraging evidence of short-term cardioprotection (75); however, the long-term avoidance of cardiotoxicity with the use of this agent has yet to be sufficiently determined. The most recent study by Lipshultz et al. reported that dexrazoxane prevents or reduces cardiac injury, as reflected by elevations in troponin T, which is associated with the use of doxorubicin for childhood ALL without compromising the antileukemic efficacy of doxorubicin. Longer follow-up will be necessary to determine the influence of dexrazoxane on echocardiographic findings at 4 years and on event-free survival (76).
Another key emerging issue is the interaction of taxanes with doxorubicin. Epirubicin-taxane combinations are active in treating metastatic breast cancer, and ongoing research is focusing on combining anthracyclines with taxanes in an effort to continue to improve outcomes following adjuvant therapy (77). Clinically significant drug interactions have been reported to occur when paclitaxel is administered with doxorubicin, cisplatin, or anticonvulsants (phenytoin, carbamazepine, and phenobarbital), and pharmacodynamic interactions have been reported to occur with these agents that are sequence- or schedule dependent (78). Because the taxanes undergo hepatic oxidation through the cytochrome P-450 system, pharmacokinetic interactions from enzyme induction or inhibition can also occur. A higher than expected myelotoxicity has been reported. However, there is no enhanced doxorubicinol formation in human myocardium, a finding consistent with the cardiac safety of the regimen (79). Investigators have suggested that doxorubicin and epirubicin should be administered 24 hours before paclitaxel and the cumulative anthracycline dose be limited to 360 mg per m2, thereby preventing the enhanced toxicities caused by sequence- and schedule-dependent interactions between anthracyclines and paclitaxel (78). Conversely, they also suggest that paclitaxel should be administered at least 24 hours before cisplatin to avoid a decrease in clearance and increase in myelosuppression. With concurrent anticonvulsant therapy, cytochrome P-450 enzyme induction results in decreased paclitaxel plasma steady-state concentrations, possibly requiring an increased dose of paclitaxel. A number of other drug interactions have been reported in preliminary studies for which clinical significance has yet to be established (78).
The human epidermal growth factor receptor (HER) 2 is overexpressed in approximately 20–25% of human breast cancers and is an independent adverse prognostic factor. Targeted therapy directed against this receptor has been developed in the form of a humanized monoclonal antibody, trastuzumab. Unexpectedly, cardiac toxicity has developed in some patients treated with trastuzumab, and this has a higher incidence in those treated in combination with an anthracycline (80, 81). Both clinical and in vitro data suggest that cardiomyocyte HER2/erbB2 is uniquely susceptible to trastuzumab (82). Trastuzumab has shown activity as a single agent in metastatic breast cancer both before chemotherapy and in heavily pretreated patients, and its use in combination with an anthracycline or paclitaxel results in a significant improvement in survival, time to progression, and
response (80). The HER2 status of a tumor is a critical determinant of response to trastuzumab-based treatment; those expressing HER2 at the highest level on immunohistochemistry, 3+, derive more benefit from treatment with trastuzumab than those with overexpression at the 2+ level. Interactions between the estrogen receptor and HER2 pathway have stimulated interest in using trastuzumab in combination with endocrine therapy.
response (80). The HER2 status of a tumor is a critical determinant of response to trastuzumab-based treatment; those expressing HER2 at the highest level on immunohistochemistry, 3+, derive more benefit from treatment with trastuzumab than those with overexpression at the 2+ level. Interactions between the estrogen receptor and HER2 pathway have stimulated interest in using trastuzumab in combination with endocrine therapy.
Neurocognitive Sequelae
Long-term survivors of cancer may be at risk of neurocognitive and neuropsychological sequelae. Among survivors of childhood leukemia, neurocognitive late effects represent one of the more intensively studied topics. Adverse outcomes are generally associated with whole-brain radiation and/or therapy with high-dose systemic or intrathecal methotrexate or cytarabine (83, 84, 85). High-risk characteristics, including higher dose of central nervous system (CNS) radiation, younger age at treatment, and female sex, have been well documented. Results from studies of neurocognitive outcomes are directly responsible for the marked reduction (particularly in younger children) in the use of cranial radiation, which is currently reserved for treatment of very high-risk subgroups or patients with CNS involvement (86).
A spectrum of clinical syndromes may occur, including radionecrosis, necrotizing leukoencephalopathy, mineralizing microangiopathy, and dystrophic calcification, cerebellar sclerosis, and spinal cord dysfunction (87). Leukoencephalopathy has been primarily associated with methotrexate-induced injury of white matter. However, cranial radiation may play an additive role through the disruption of the blood–brain barrier, therefore allowing greater exposure of the brain to systemic therapy.
Although abnormalities have been detected by diagnostic imaging studies, the abnormalities observed have not been well demonstrated to correlate with clinical findings and neurocognitive status (88, 89). Chemotherapy- or radiation-induced destruction in normal white matter partially explains intellectual and academic achievement deficits (90). Evidence suggests that direct effects of chemotherapy and radiation on intracranial endothelial cells and brain white matter as well as immunologic mechanisms could be involved in the pathogenesis of CNS damage.
Neurocognitive deficits, as a general rule, usually become evident within several years of CNS radiation and tend to be progressive in nature. Survivors of leukemia treated at a younger age (e.g., <6 years of age) may experience significant declines in intelligence quotient (IQ) scores (91). However, reductions in IQ scores are typically not global, but rather reflect specific areas of impairment, such as attention and other nonverbal cognitive processing skills (92). Affected children may experience information-processing deficits, resulting in academic difficulties. These children are particularly prone to problems with receptive and expressive language, attention span, and visual and perceptual motor skills, most often manifested in academic difficulties in the areas of reading, language, and mathematics. Accordingly, children treated with CNS radiation or systemic or intrathecal therapy with the potential to cause neurocognitive deficits should receive close monitoring of academic performance. Referral for neuropsychological evaluation with appropriate intervention strategies, such as modifications in curriculum, speech and language therapy, or social skills training, implemented in a program tailored for the individual needs and deficits of the survivor should be taken into consideration (93). Assessment of educational needs and subsequent educational attainment have found that survivors of childhood leukemia are significantly more likely to require special educational assistance, but have a high likelihood of successfully completing high school (37, 94). However, when compared with siblings, survivors of leukemia and non-Hodgkin’s lymphoma (NHL) are at greater risk of not completing high school. As would be anticipated from the results of neurocognitive studies, it has been shown that survivors, particularly those under 6 years of age at treatment, who received cranial radiation and/or intrathecal chemotherapy were significantly more likely to require special education services and least likely to complete a formal education (86, 95, 96).
Progressive dementia and dysfunction have been reported in some long-term cancer survivors as a result of whole-brain radiation with or without chemotherapy, and occur most often in patients with brain tumor and patients with small cell lung cancer who have received prophylactic therapy. Neuropsychological abnormalities have also been reported after CNS prophylaxis utilizing whole-brain radiation for leukemia in childhood survivors. In fact, cognitive changes in children began to be recognized as treatments for childhood cancer, especially ALL, became increasingly effective. These observations have resulted in changes in treatment protocols for childhood ALL (97, 98).
Several recent studies have reported cognitive dysfunction in women treated with adjuvant therapy for breast cancer (99, 100). In one study (101), investigators compared the neuropsychological performance of long-term survivors of breast cancer and lymphoma treated with standard-dose chemotherapy who carried the epsilon 4 allele of the apolipoprotein E (APOE) gene to those who carry other APOE alleles. Survivors with at least one epsilon 4 allele scored significantly lower in the visual memory (p <.03) and the spatial ability (p <.05) domains and tended to score lower in the psychomotor functioning (p <.08) domain as compared with survivors who did not carry an epsilon 4 allele. No group differences were found on depression, anxiety, or fatigue. The results of this study provide preliminary support for the hypothesis that the epsilon 4 allele of APOE may be a potential genetic marker for increased vulnerability to chemotherapy-induced cognitive decline.
Although cranial irradiation is the most frequently identified causal factor in both adults and children, current work in adults indicates that cognitive problems may also occur with surgery, chemotherapy, and biologic response modifiers (102, 103, 104). These findings need to be validated in prospective studies along with the interaction among treatment with chemotherapeutic agents, menopausal status, and hormonal treatments. Emotional distress has also been related to cognitive issues in studies of patients beginning cancer treatment.
Patients have attributed problems in cognition to fatigue, and others have reported problems with concentration, short-term memory, problem-solving, and concerns about “chemobrain” or “mental pause” (105). Comparisons across studies are difficult because of different batteries of neuropsychological tests used, and differences among patient samples by diagnosis, age, gender, or type of treatment received, and, finally, inconsistency in the timing of measures in relation to treatment landmarks. Despite these methodological issues, studies have shown impairments in verbal information processing, complex information processing, concentration, and visual memory (106, 107, 108, 109).
Current studies indicate that cognitive deficits are often subtle but a observed consistently in a proportion of patients, may be durable, and can be disabling (110). Deficits have been observed in a range of cognitive functions. Although underlying mechanisms are unknown, preliminary studies suggest a genetic predisposition. Cognitive impairment may be accompanied by changes in the brain, detectable by neuroimaging. Priorities for future research include the following:
Large-scale clinical studies that use both a longitudinal design and concurrent evaluation of patients with cancer who do not receive chemotherapy. Such studies should address the probability and magnitude of cognitive deficits, factors that predict them, and underlying mechanisms
Exploration of discrepancies between subjective reports of cognitive dysfunction and the objective results of cognitive testing
Studies of cognitive function in patients receiving treatment for diseases other than breast cancer, and in both men and women, to address the hypothesis that underlying mechanisms relate to changes in serum levels of sex hormones and/or to chemotherapy-induced menopause
Development of interventions to alleviate these problems and
Development of animal models and the use of imaging techniques to address mechanisms that might cause cognitive impairment
Endocrinologic Sequelae
Thyroid
Radiation exposure to the head and neck is a known risk factor for subsequent abnormalities of the thyroid. Among survivors of HD and, to a lesser extent, survivors of leukemia, abnormalities of the thyroid gland, including hypothyroidism, hyperthyroidism, and thyroid neoplasms, have been reported to occur at rates significantly higher than those found in the general population (111, 112, 113, 114). Hypothyroidism is the most common nonmalignant late effect involving the thyroid gland. Following radiation doses above 15 Gy, laboratory evidence of primary hypothyroidism is evident in 40–90% of patients with HD, NHL, or head and neck malignancies (113, 115, 116). In a recent analysis of 1791 5-year survivors of pediatric HD (median age at follow-up, 30 years), Sklar et al. reported the occurrence of at least one thyroid abnormality in 34% of subjects (114). The risk of hypothyroidism was increased 17-fold compared with sibling control subjects, with increasing dose of radiation, older age at diagnosis of HD, and female sex as significant independent predictors of an increased risk. The actuarial risk of hypothyroidism for subjects treated with 45 Gy or more was 50% at 20 years following diagnosis of their HD. Hyperthyroidism was reported to occur in only 5%. Finally, it is important to note that the risk of hypothyroidism in adult patients treated with mantle irradiation for HD is significant. Most of the adult cases occur in the first 5 years but the risk is lifelong. These issues are of key importance for survivors of adult cancer with a history of RT for head and neck cancer.
Hormones affecting growth
Poor linear growth and short adult stature are common complications after successful treatment of childhood cancers (117). The adverse effect of CNS radiation on final height as an adult among patients with childhood leukemia has been well documented, with final heights below the fifth percentile occurring in 10–15% of survivors (43, 118, 119). The effects of cranial radiation appear to be related to age and gender, with children younger than 5 years at the time of therapy and female patients being more susceptible. The precise mechanisms by which cranial radiation induces short stature are not clear. Disturbances in growth hormone production have not been found to correlate well with observed growth patterns in these patients (31, 120). The phenomenon of early onset of puberty in girls receiving cranial radiation may also play some role in the reduction of final height (33, 121). In survivors of childhood leukemia, not treated with cranial radiation, there are conflicting results regarding the impact of chemotherapy on final height (122).
Hormonal rationale for obesity
An increased prevalence of obesity has been reported among survivors of childhood ALL (123, 124, 125). Craig et al. investigated the relationship between cranial irradiation received during treatment for childhood leukemia and obesity (126). Two hundred thirteen (86 boys and 127 girls) irradiated patients and 85 (37 boys and 48 girls) nonirradiated patients were enrolled. For cranially irradiated patients, an increase in the body mass index (BMI) Z score at the final height was associated with female sex and lower radiation dose but not with age at diagnosis. Severe obesity, defined as a BMI Z score >3 at final height, was present only in girls, who received 18–20 Gy irradiation, at a prevalence rate of 8%. Both male and female nonirradiated patients had raised BMI Z scores at latest follow-up, and there was no association with age at diagnosis. The authors concluded that these data demonstrated a sexually dimorphic and dose-dependent effect of cranial irradiation on BMI. In a recent analysis from the Childhood Cancer Survivor Study, Oeffinger et al. compared the distribution of BMI of 1765 adult survivors of childhood ALL with that of 2565 adult siblings of survivors of childhood cancer (127). Survivors were significantly more likely to be overweight (BMI, 25–30) or obese (BMI, 30 or more). Risk factors for obesity were cranial radiation, female gender, and age from 0 to 4 years at diagnosis of leukemia. Girls diagnosed under the age of 4 who received a cranial radiation dose >20 Gy were found to have a 3.8-fold-increased risk of obesity.
Gonadal dysfunction
Treatment-related gonadal dysfunction has been well documented in both men and women following childhood malignancies (128). However, survivors of leukemia and T-cell NHL treated with modern conventional therapy are at a relatively low risk of infertility and delayed or impaired puberty. Treatment-related gonadal failure or dysfunction, expressed as amenorrhea or azoospermia, can lead to infertility in both male and female survivors of cancer, and may have its onset during therapy (129). Infertility can be transient, especially in men, and may recover over time after therapy. Reversibility is dependent on the dose of gonadal radiation or alkylating agents. Ovarian function is unlikely to recover long after the immediate treatment period because long-term amenorrhea commonly results from loss of ova. Cryopreservation of sperm before treatment is an option for men (130), but limited means are available to preserve ova or protect against treatment-related ovarian failure for women (131, 132, 133). A successful live birth after orthotopic autotransplantation of cryopreserved ovarian tissue has been recently reported (134, 135, 136, 137). A reasonable body of research on topics relating to the long-term gonadal effects of radiation and chemotherapy exists (138, 139, 140, 141, 142, 143, 144, 145, 146, 147, 148, 149, 150, 151, 152, 153, 154, 155, 156, 157, 158, 159, 160, 161). and provides a basis for counseling patients and parents of the anticipated outcomes on pubertal development and fertility. A detailed review of this topic is beyond the scope of this chapter.
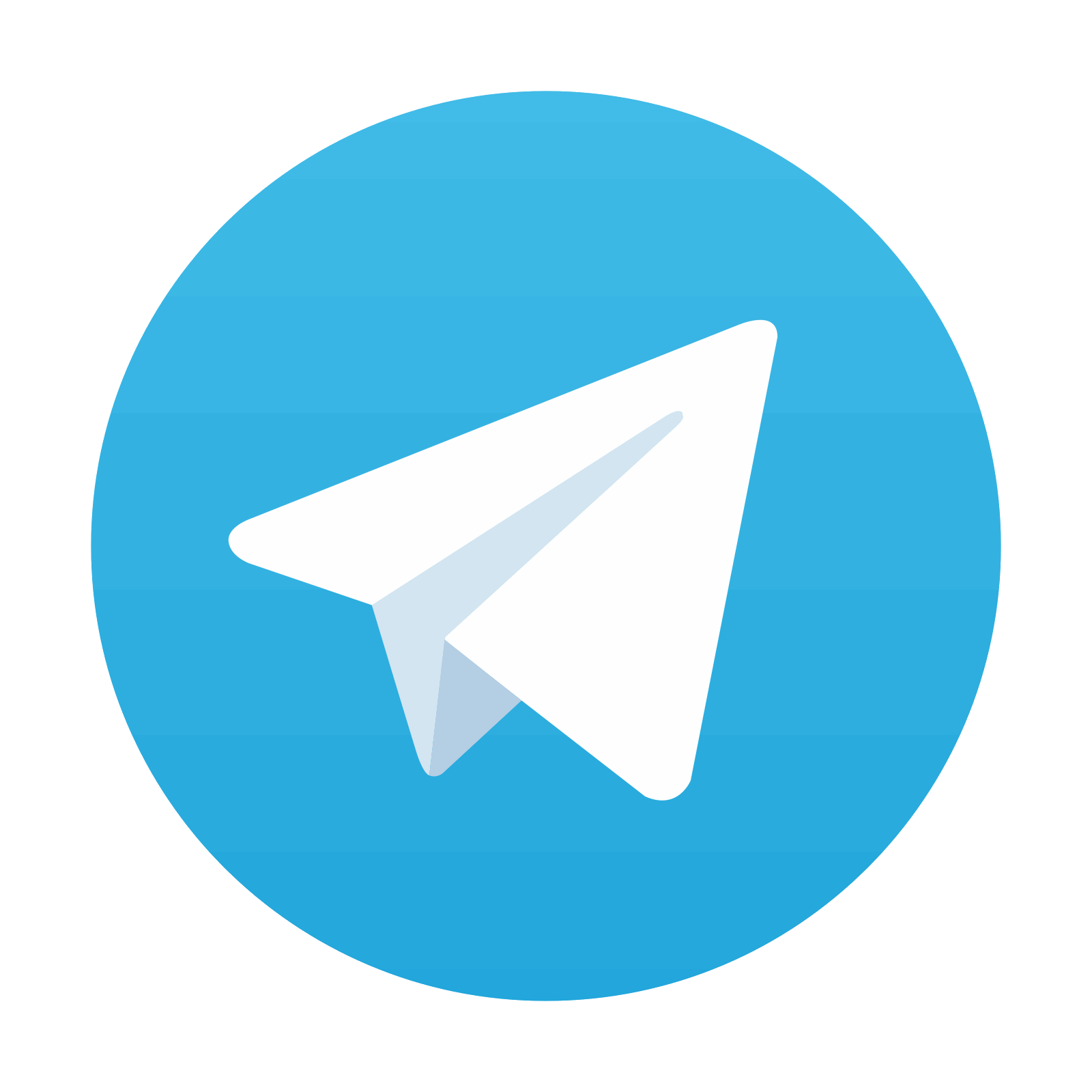
Stay updated, free articles. Join our Telegram channel

Full access? Get Clinical Tree
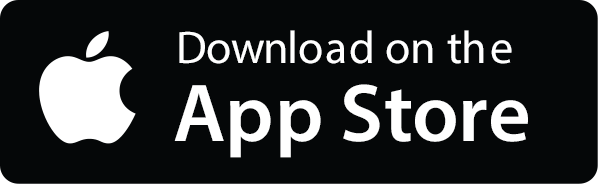
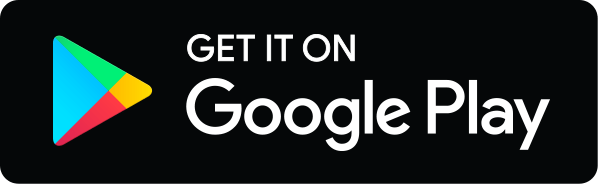
