Modality
Advantages
Limitations
Sensitivity (%)
CT
– Widespread availability
– poor detection and characterization of lesions <10 mm
70–85
– Relatively low cost
– reduced sensitivity in setting of chemotherapy induced liver injury (steatosis)
– Rapid image acquisition
– requires iodinated contrast
– Evaluation of EHD
– contraindicated in patients with renal dysfunction/failure
– Volume assessment of FLR
– radiation exposure
– Vascular mapping (CTA)
– Therapeutic monitoring
MRI
– improved detection/characterization of:
– limited availability
91–97
– small lesions (<10 mm)
– image acquisition time consuming requiring significant patient compliance
– lesions in the setting of chemotherapy induced liver injury (steatosis)
– expensive
– no radiation exposure
– contraindicated in setting of certain medical implants (PM, stents etc.)
FDG PET
– Evaluation of EHD
– limited availability
78–95
– surveillance for recurrence
– high false positive rates, leading to unnecessary work-up
– increased sensitivity/specificity when combined with CT
– expensive
– poor detection following chemotherapy
– poor detection of lesions <10 mm
Dynamic contrast enhanced computerized tomography refers to CT imaging of the liver in which images are obtained in three phases (triphasic); non-contrast, arterial, and portovenous. CRLM are typically hypoattenuating compared to the background liver parenchyma, and are best visualized during the portovenous phase (Fig. 1a). Sensitivity of modern thin slice, triphasic, contrast enhanced CT for the detection of CRLM ranges from 80 to 90 % and is significantly higher than standard contrast enhanced CT (sensitivity 60–75 %) [25]. These performance characteristics, in conjunction with widespread availability and relative cost-effectiveness, have led dynamic CT to be the most common radiology investigation for evaluation of CRLM [30]. However, CT is limited in its ability to identify and characterize smaller liver lesions, specifically those lesions <10 mm. Wiering et al. noted that despite CT being 97 % successful in detecting lesions >20 mm and 72 % successful for lesions 10–20 mm, CT performed poorly for lesions <10 mm, with a success rate of only 16 % [31]. Furthermore, previous treatment with cytotoxic chemotherapy may lead to steatosis of the underlying liver parenchyma and a subsequent decrease in the ability to discriminate between CRLM and normal liver. This phenomenon significantly decreases the sensitivity of CT to detect CRLM [32]. This has been observed in multiple studies. A recently published meta-analysis suggests pooled sensitivity estimates for CT following chemotherapy to be 69.9 % (65.6–73.9 %) compared to 80.5 % (67.0–89.4 %) in chemotherapy naïve patients [33]. Despite these limitations, CT remains the most common initial radiology investigation for evaluation of CRLM [30], with reservation of alternative investigations for “problem-solving” in the setting of lesions too small to characterize on CT or in chemotherapy treated patients.
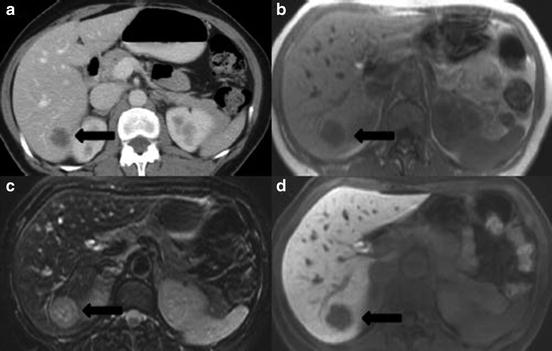
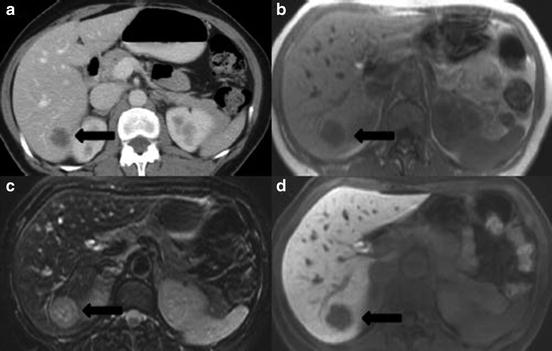
Fig. 1
Comparison of radiographic imaging modalities for colorectal cancer liver metastases with, (a) dynamic contrast enhanced CT-portovenous phase, (b) T1-weighted MRI, (c) T2-weighted MRI with fat suppression, (d) T1 weighted MRI post contrast with liver-specific contrast media (EOVIST™) on delayed hepatobiliary phase (
tumor)

Magnetic resonance imaging (MRI) provides exquisite soft tissue resolution when compared to other imaging modalities. As a consequence MRI offers several advantages in terms of detection and characterization of smaller lesions, and evaluation of the liver in the setting of previous chemotherapy or other underlying parenchymal abnormalities. CRLM appear hypointense to isointense on T1-weighted images, and isointense to hyperintense on T2-weighted images (Fig. 1b/c). Overall, the sensitivity of contrast enhanced MRI to detect CRLM is reported to range from 91 to 97 % [34] and the accuracy in stratifying too small to characterize lesions (<10 mm) as benign or malignant is over 90 %. Furthermore, specificity of liver MRI for the diagnosis of malignant lesions far exceeds that of CT (97.5 % versus 77.3 %) [35]. Use of chemotherapy can lead to steatosis and increasing amounts of fat within the liver which makes CRLM more difficult to detect. MRI is advantageous in this setting as images can be reformatted using “fat-suppression” techniques leading to improved detection of CRLM when compared to other imaging modalities. Meta-analyses of studies of patients with CRLM previously treated with chemotherapy suggest a sensitivity of liver MRI of 85.7 % (69.7–94.0 %), which is significantly improved over other imaging techniques [33]. Development of newer tissue-specific contrast agents such as gadozetate (Gd-EOB-DTPA; Eovist), that are taken up by hepatocytes can further improve the performance of MRI in identifying and characterizing CRLM (Fig. 1d) [36]. Additionally, diffusion weighted MRI (DWI), which relies on the differential proton diffusion characteristics between benign and malignant tissue, is another modification of standard MRI that may improve evaluation the liver for CRLM [37] The major limitations of MRI evaluation is the requirement for substantial patient compliance to minimize motion artifact, poor assessment of disease outside the liver, high cost, and limited availability, as such it is not the ideal initial investigation in the setting of CRLM. However, evidence suggests that available MRI techniques allow better characterization of small (<10 mm) CRLM and improve detection rates in the setting of previous chemotherapy. It is this subset of patients for whom use of MRI is likely warranted.
Over the last two decades novel functional imaging techniques such as positron emission tomography using the radiolabelled 18-fluoro-deoxyglucose (FDG-PET) have proven useful in the detection of cancer. Images associated with FDG PET are dependent on the degree of uptake and accumulation of radiolabeled tracer. Cells that are highly active metabolically, such as cancer cells, tend to take up and hold onto greater amounts of radiotracer when compared to less active cells. Visualized foci of increased uptake are quantified using the standardized uptake value (SUV = activity per unit volume/injected activity per body weight). To date, FDG PET has been extensively evaluated in the setting of CRLM. Multiple meta-analysis and systematic reviews of trials regarding the diagnostic performance of FDG PET have been published [38–41] and suggest overall sensitivity of the test for the detection of CRLM to be significantly improved over MRI and CT (94.1 % versus 88.2 % and 83.6 %, respectively) [41]. However, much criticism exists regarding the implication of these findings and many suggest these studies are hampered by inappropriate comparison groups with poor quality imaging, thus FDG PET is typically reserved for problem-solving and/or evaluation for extrahepatic disease. Although FDG PET performs very well as a diagnostic test, it lacks spatial resolution of anatomic details, and evaluation of technical resectability is not feasible. Fusion of FDG PET with high quality CT (PET-CT) is an attempt to combat this limitation and is rapidly replacing stand alone PET scanning. Overall, the combined PET-CT allows for highly sensitive detection of CRLM and impressive tumor localization [30]. FDG PET is advantageous in that the entire body is imaged and its use as a component of the preoperative extent of disease work up for patients with CRLM and concern for EHD has been evaluated. Earlier studies evaluating PET-CT in this setting suggested improved evaluation of tumor burden and a subsequent reduction in futile laparotomy of up to 20 % [42] Based on this, it was concluded that routine use of preoperative staging PET-CT was essential to avoid unnecessary surgery. However, most recently, a randomized control trial of preoperative PET-CT versus no PET-CT in patients with initially resectable CRLM was conducted and the impact on surgical management was assessed [43]. In the 263 patients undergoing PET-CT in this trial, overall surgical plan was changed (canceled, more extensive liver resection, or more extensive extrahepatic resection) in only 23 (8.7 %) patients. More importantly, of these changes in management only 9 (2.7 %) patients avoided futile laparotomy, which is in stark contrast to the 20 % previously reported [42]. Major limitations to FDG-PET and PET-CT include limited accessibility, high cost, and poor diagnostic sensitivity for lesions <10 mm and in the setting of previous chemotherapy [33]. These limitations in conjunction with the best available evidence suggest that standardized use of PET-CT as a staging tool in patients with CRLM rarely results in clinically significant changes in management plans and is not cost-effective. PET-CT use should be reserved for cases in which diagnostic uncertainty regarding the presence or absence of EHD exists.
Radiographic imaging plays an integral role in the detection and characterization of CRLM as well as identification of EHD. To date, no gold standard imaging modality for evaluation of patients with CRLM has been defined. Based on currently available evidence, and cost-effectiveness, a reasonable algorithm for investigation of CRLM would include; initial dynamic CT (in most patients this will be adequate to determine technical resectability and extent of disease), if lesions are seen on CT that are too small to characterize (<10 mm) or if there is evidence of significant chemotherapy induced parenchyma injury, MRI should be performed. PET-CT is expensive and unnecessary in most patients. Its use should be reserved for those difficult cases in which delineation of potential EHD is necessary.
Evaluation of the Future Liver Remnant
FLR refers to the portion of liver that will remain in situ following hepatic resection and is one of the major determinants of technical resectability and postoperative morbidity. Evaluation of both quantity (volume) and quality (uptake/excretion/synthetic function) of the FLR is important in order to determine risk of postoperative liver insufficiency [19]. FLR volume is primarily determined using preoperative cross sectional imaging. Using available imaging and standard radiologic software, the proposed line of transection is drawn and the remnant volume of liver is subsequently calculated. Typically, FLR is measured as a proportion of total liver volume (TLV) and expressed as a percentage. It may also be evaluated and expressed as a ratio of FLR to body weight (BW) [44] Any cysts, tumors, and/or zones of previous ablation are outlined and excluded from the final measured volumes. Adequate FLR volume is essential to minimize the risk of postoperative liver insufficiency. However, the absolute volume of FLR required is dependent on the status of the underlying liver parenchyma. Multiple methods for evaluating hepatic reserve/function and predicting outcome exist. In North America clinicians tend to rely heavily on single laboratory values (total bilirubin) and or clinical scoring systems (Child-Pugh, MELD) [45] to estimate degree of liver dysfunction and potential risk. On the other hand, in the East, indocyanine green clearance tests measuring hepatic perfusion and excretory function are a common means of assessing liver function [46]. Accepted minimal FLR is contingent on both volume and function. Although data are limited, generally accepted minimal static FLR values for normal liver, chemotherapy treated liver, and cirrhotic liver are; >20 %, >30 % and >40 %, respectively [47]. Based on thorough evaluation FLR may be considered adequate, borderline, or insufficient for safe resection. In the foremost group surgical resection may be pursued without further intervention. In patients with borderline and insufficient FLR techniques have been developed to induce hypertrophy of the remnant liver such that resection may be achievable.
Strategies to Increase Future Liver Remnant Volume
Laboratory studies conducted as early as the 1920s indicated that interruption of the portal venous flow to the liver resulted in atrophy of the ipsilateral segment and compensatory hypertrophy of the contralateral side [48]. This translated into the clinical hypothesis that in patients requiring major hepatic resection, where FLR would be inadequate, diversion of portal blood flow from the portion of liver to be resected could result in hypertrophy of the FLR and subsequently allow safer resection of larger volumes of liver.
The most common method to achieve cessation of portal flow is percutaneous embolization. In the early 1980s proof of concept studies by Kinochita et al. [49] described perioperative portal vein embolization (PVE) in humans. Later PVE was used on a larger scale clinically with success in the setting of major resection for hilar cholangiocarcinoma and other primary hepatic malignancies [50]. In 2003, Belghiti et al. published a prospective trial of right hepatectomy with and without preoperative PVE. Subgroup analysis of patients with and without chronic liver disease (CLD) revealed that although hypertrophy of the FLR occurs in both groups, clinical benefit in terms of reduced overall morbidity and liver insufficiency was only observed in patients with CLD, no benefit of PVE was observed in the patients with normal underlying liver parenchyma [51]. To date no other prospective trial of PVE has been completed and present indications for preoperative PVE include any hepatic resection in which concern exists regarding the adequacy of the FLR. It is generally accepted that in order to minimize the risk of perioperative liver insufficiency PVE should be considered if the post-resectional liver volume is estimated to be ≤20 % [ 19, 52]. PVE is most commonly completed via percutaneous transhepatic route. Open techniques have also been described with access to the portal vein via iliocolic branches; although equally as effective in inducing hypertrophy, this requires mini-laparotomy and is rarely utilized [50]. Typically, 4 weeks following the procedure, adequacy of PVE is assessed with repeat cross section imaging and liver volumetry. Figure 2 illustrates CT images and volumetric rendering of the liver prior to and following PVE. In a recent meta-analysis typical absolute volume changes observed with PVE ranged from 8 to 27 % [52]. Traditionally, static measurements of the FLR (sFLR) volume have been used in the prediction of postoperative liver insufficiency. More recently, however, it has been suggested that it is not only the absolute sFLR volume (i.e., >20 % sFLR in normal liver) but also the degree of hypertrophy (DH) [53] and the kinetic growth rate (KGR) [54] of the FLR following PVE that are important in assessment of the adequacy of the FLR. Many believe these dynamic measures (DH, KGR) are the best in vivo markers of the functional capacity of the liver. Therefore, in conjunction with sFLR >20 %, DH > 5 % and a KGR of >2 %/week following PVE are considered minimal acceptable parameters for safe liver resection.
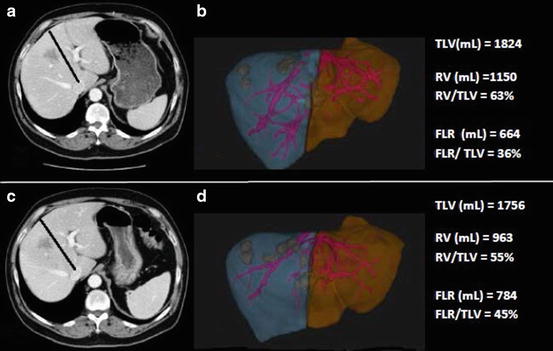
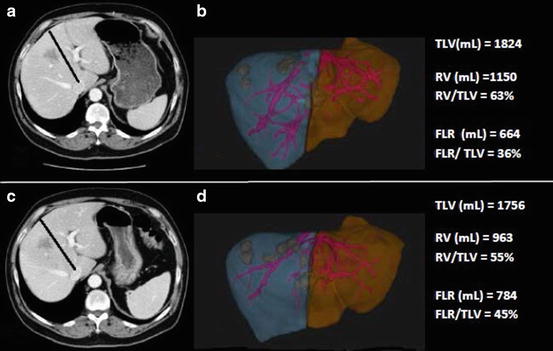
Fig. 2
CT imaging and volumetric rendering of the liver; (a) before portal vein embolization and, (b) after portal vein embolization (
line of proposed parenchymal transection, TLV = total liver volume, RV = resection volume, FLR = future liver remnant)

Portal vein ligation (PVL) is another technique in which hypertrophy of the FLR may be achieved. Results with this technique appear to be equivalent to those obtained with PVE [55]. However, PVL, is significantly more invasive requiring either mini-laparotomy or laparoscopy to complete and at present, outside of its use in two-stage resections, it is rarely used. Most recently, in attempts to induce more rapid and greater hypertrophy of the FLR a surgical procedure has been described in which partitioning of the liver is combined with portal vein ligation (Associating liver partition and portal vein ligation for staged hepatectomy-ALPPS) [56–58]. The concept behind this procedure is that combination of PVL with in situ splitting of the liver prevents the development of collateral circulation between segments and leads to more complete vascular isolation of the FLR, optimizing conditions for hypertrophy of the FLR. Although initial studies show significant and rapid increases in FLR (median increase 74 %, median time 9 days) the overall complication rate exceeds 60 %, with major complications occurring in over 40 % of patients, and in hospital death reported in 12 % [58]. Furthermore, the procedure requires two laparotomies within 7–10 days of each other which can be exceedingly arduous and taxing for patients. It appears that although novel in concept, the risks associated with ALPPS are significant and the benefits have yet to be seen, as such general application is not recommended.
Assessment of Prognosis
Over the past 20 years multiple different scoring systems and predictive models have been developed to help prognosticate patients with resectable CRLM (Table 2) [59–70]. Despite the heterogeneity in patient populations and era of study, the majority of these systems incorporate some variation of factors related to the pathology of the primary CRC, extent of disease (primary tumor and metastases), and timing of CRLM development relative to primary CRC. To date there is no uniformly accepted or “ideal” prognostic scoring system, however, the clinical risk score (CRS) as reported by Fong et al. in 1999 [60] is the most widely used [71, 72]. Based on multivariate analysis of preoperative clinicopathologic factors it is comprised of five criteria including, disease-free interval (DFI) < 12 months, size >5 cm, tumor number >1, CEA >200 ng/mL, and node positive primary, each of which is given a single point and a sum total score is generated. In the initial study by Fong et al. the total score was highly predictive of OS (p < 0.0001), with actuarial 5 year OS of 60 % and 14 %, for scores of 0 and 5, respectively. Since its inception the clinical utility of this scoring system has been evaluated extensively and has been validated in other study cohorts [67, 71, 73–75], however, its external validity has not been universally observed [67].
Table 2
Comparison of prognostic scoring systems
Author (year, location) | Number of patients | Time frame | Prognostic factors | Risk groups (score) | Outcome (5-year) |
---|---|---|---|---|---|
Nordlinger et al., 1996; France [59] | 1568 | 1968–1990 | – Age ≥ 60 | Low (0–2) | 2 year—79 % |
– Serosal invasion of primary | Intermediate (3–4) | 60 % | |||
– Node + primary | High (5–7) | 43 % | |||
– DFI < 2 years | |||||
– Size ≥5 cm | |||||
– ≥ 4 tumors | |||||
– Margin ≤1 cm | |||||
Fong et al., 1999; USA [60] | 1001 | 1985–1998 | – Margin + | 0 (0) | 60 % |
– EHD | 1 (1) | 44 % | |||
– Node + primary | 2 (2) | 40 % | |||
– DFI ≤12 month | 3 (3) | 20 % | |||
– Size ≥5 cm | 4 (4) | 25 % | |||
– > 1 tumor | 5 (5) | 14 % | |||
– CEA ≥200 ng/mL | |||||
Iwatsuki et al., 1999; USA [61] | 305 | 1981–1996 | – ≥2 tumors | Grade 1 (0) | 48 % |
– Size ≥8 cm | Grade 2 (1) | 34 % | |||
– Bilobar distribution | Grade 3 (2) | 18 % | |||
– DFI ≤30 months | Grade 4 (3) | 6 % | |||
– Margin + | Grade 5 (4) | 1 % | |||
– EHD | Grade 6 (R1 or EHD) | 0 % | |||
Ueno et al., 2000; Japan [62] | 85 | 1985–1996 | – Primary tumor with marked budding and/or node + | Stage A (0–1) | 55 % |
– DFI < 1 year | Stage B (2) | 14 % | |||
– ≥ 3 tumors | Stage C (3) | 0 % | |||
Lise et al., 2001; Italy [63] | 132 | 1977–1997 | – > 30 % liver invasion | Group A (0–2) | 3 Year |
– Node + primary | Group B (3–5) | 80 % | |||
– > 1 tumor | Group C (≥6) | 55 % | |||
– GPT ≥55U/L | 10 % | ||||
– Non-anatomic resection | |||||
Nagashima et al., 2004; Japan [64] | 81 | 1981–1997 | – Serosal invasion of primary | Grade A (0–1) | 85 % |
– Node + primary | Grade B (2–3) | 56 % | |||
– Resectable EHD | Grade C (>3) | 0 % | |||
– >1 tumor | |||||
– Size > 5 cm | |||||
Schindl et al., 2005; UK [65] | 270 | 1988–2002 | – Duke stage C | Good (0–10) | Median OS |
– CEA level | Moderate (11–25) | 60 months | |||
– ALP | Poor (>25) | 32 months | |||
– Albumin | 22 months | ||||
– >3 tumors | |||||
Malik et al., 2007; UK [66] | 687 | 1993–2006 | – Inflammatory response to tumor | 0 (0) | 49 % |
– ≥ 8 tumors | 1 (1) | 34 % | |||
2 (2) | 0 % | ||||
Zakaria et al., 2007; USA [67] | 662 | 1960–1995 | – DFI ≤ 30 months | Group 1 (0) | 55 % |
– Size ≥ 8 cm | Group 2 (BT) | 39 % | |||
– Blood Transfusion | Group 3 (HDN) | 20 % | |||
– Hepatoduodenal lymph node + | |||||
Lee et al., 2008; Korea [68] | 135 | 1994–2005 | – Margin ≤5 mm | Low (0–1) | 46 % |
– CEA > 5 ng/mL | Intermediate (2) | 41 % | |||
– Node + primary | High (3–4) | 11 % | |||
– > 1 tumor | |||||
Rees et al., 2008; UK [69] | 929 | 1987–2005 | – > 1 tumor | 0 (0) | Pre/Post |
Evaluated preoperative and postoperative riska | – Node + primary | 1–5 (1–5) | 66 %/64 % | ||
– Poorly differentiate primary | 6–10 (6–10) | 51 %/49 % | |||
– EHD | 11–15 (11–15) | 35 %/34 % | |||
– Size ≥ 5 cm | >15 (>15) | 21 %/21 % | |||
– CEA > 60 ng/mL | 2 %/2 % | ||||
– Margin + | |||||
Konopke et al., 2009; Germany [70] | 201 | 1993–2006 | – ≥ 4 tumors | Low (0) | Median OS |
– Synchronous metastases | Intermediate (1) | 67 months | |||
– CEA ≥ 200 ng/mL | High (≥2) | 47 months | |||
38 months |
More recently, the value and relevance of these clinical scoring systems has been called into question [9, 72, 76, 77]. The primary issue surrounding these models is the fact that they were developed using retrospective analysis of historical cohorts, including patients from over 40 years ago. Within that time frame the management of CRLM has drastically changed and our ability to assess patient-specific tumor biology and evaluate molecular markers of prognosis has increased exponentially. To date multiple studies have attempted to identify potential radiologic, pathologic and molecular markers of prognosis. To this end, KRAS, a GTPase that when activated induces the mitogen-activated protein kinase (MAPK) cascade, has been the most extensively evaluated with respect to prognosis. CRLM with mutant KRAS have not only been associated with resistance to epidermal growth factor targeted antibodies, but in recent studies have been associated with significantly worse OS, DSS, and RFS [78, 79]. A variety of other molecular markers have also been assessed with respect to outcome in patients with CRLM and are outlined in Box 1.
Box 1: Association of Molecular Markers and Survival in CRLM
Biomarker | Prevalence | Impact on survival |
---|---|---|
40 % | Independent predictor of worse OS, DSS, and RFS | |
BRAF mutation [80] | 5–10 % | Independent predictor of worse OS |
20–80 % (overexpression) | Overexpression is associated with worse OS and RFS | |
– N/A | Independent predictor of worse OS; correlation with survival better than CRS | |
– N/A | Independent predictor of worse OS; correlation with survival better than CRS | |
Hypoxia inducible factor-1α [85] | 30 % (overexpression) | Overexpression is an independent risk factor for disease recurrence |
Modern chemotherapeutics are increasingly being used in the neoadjuvant setting, and have generated significant interest in the evaluation of the relationship between response to therapy and prognosis. Degree of tumor response to chemotherapy can be assessed radiographically, most commonly using size-based measures such as response evaluation criteria in solid tumors (RECIST). Using these criteria multiple studies have revealed that patients with CRLM who demonstrate radiographic progression while on treatment have worse OS, DSS, and RFS [86–88]. More recent reports, however, suggest that even with disease progression on chemotherapy, patients who remain resectable may have OS similar to those in whom complete or partial responses are observed [89]. Response to treatment may also be evaluated pathologically. The extent of pathologic response, measured as a percentage of remaining viable tumor cells, has repeatedly been shown to positively correlate with survival and recurrence outcomes [90–92]. More recent investigations suggest that the type of pathologic response, assessed by pathologic tumor regression (fibrosis overgrowing on tumor cells, decreased necrosis, and tumor glands at the periphery), is a better indicator of tumor response to chemotherapy and is an independent predictor of DFS [93]. Molecular risk scores (MRS), generated from gene expression profiling have recently been reported [94]. The MRS appears to have prognostic value in terms of DSS as well as liver recurrence-free survival (LRFS) alone as well as in conjunction with the CRS. Parallel innovations in many different realms; radiology, pathology, molecular biology, and genetics, has led to an insurgence of data regarding CRLM biomarkers. At present, no comprehensive prognostic scoring system incorporating these new potential biomarkers with clinical criteria has been developed. However, it is certain that as cancer care becomes increasingly personalized, integration of clinical and biologic factors will be essential in determining optimal therapeutic interventions.
Resectable Colorectal Liver Metastases
General Approach
Historically, of the over 50 % of patients with CRC who develop CRLM, only 15–20 % will have disease that is amenable to surgical resection [6]. However, as advancements in all areas of treatment of CRLM continue and the boundaries of surgical resection expand, this number is certain to increase. In general, resection for CRLM may be undertaken only when patient operability has been established, resectability confirmed, and FLR adequacy verified. Once the decision to proceed with resection is made, an operative plan, with the aim of removing all tumor(s) with a clear margin while simultaneously preserving as much functional liver parenchyma as feasible, is formulated. The importance of an R0 resection on disease recurrence has been well established; however, what constitutes R0 or negative margins remains a point of some debate. Previously, surgical margins with evidence of microscopic tumor present within 1 cm of the transected parenchyma (R1) were associated with significantly worse clinical outcomes [95, 96]. In attempts to avoid margin positivity, large volume anatomic resections (hemi-hepatectomies and trisegmentectomy) were commonly employed. This approach was bolstered by early studies suggesting that non-anatomic wedge resections (WR) were associated with increased rates of margin positivity when compared to anatomic lobar and segmental resection (AR) and subsequent worse clinical outcome [97, 98]. More recently, multiple large studies call to question the need for a 1 cm negative resection margin and suggest that although a negative margin (R0 resection) is critical, the width of that margin in millimeters is not [99–102]. As the definition of adequate surgical margins changed, surgical practice shifted towards greater use of parenchymal preservation techniques (WR). With this paradigm shift away from large volume resections, concerns arose regarding oncologic outcomes. In recent studies, however, no differences in terms of margin positivity or clinical outcome have been observed between parenchymal preservation WR and AR [18, 103, 104].
Open liver resection is typically performed under low central venous pressure (LCVP) anesthesia (CVP < 5 mmHg), through an upper midline or a subcostal hockey stick (right subcostal with midline vertical extension) incision. LCVP anesthesia consists of a prehepatic resection phase where crystalloid administration is limited. CVP is kept at or below 5 mmHg in an attempt to minimize bleeding from hepatic veins during parenchymal transection and facilitate control of venous injury should it occur. Following hepatic transection, a resuscitation phase begins, where patients are returned to euvolemic state. Use of this technique is associated with reduced intraoperative blood loss, decreased perioperative blood transfusion, and has contributed significantly to lower surgical morbidity in patients undergoing liver resection [105]. Upon entering the abdominal cavity a full laparotomy is performed and a search for evidence of EHD is completed and any concerning findings are biopsied and sent for frozen section analysis. The liver is then mobilized, examined by palpation and intraoperative ultrasound (IOUS) is performed to confirm the location of all known tumors, to identify any new lesions (or residual tumor in the setting of neoadjuvant chemotherapy), and to assess tumor location relative to critical structures. Currently some controversy exists as to the overall utility of routine IOUS in the setting of CRLM. Initial studies indicated that IOUS changed operative management plan in 44–67 % of cases [106, 107] and clearly supported its value. However, some argument exists, that in the current era of high-resolution preoperative imaging routine use of IOUS is low yield and should be reserved for use only in cases in which preoperative imaging elicits concerns regarding involvement of critical structures, or in the setting of disappearing CRLM following neoadjuvant chemotherapy [108]. Despite this, IOUS adds little time to the procedure and continues to be performed routinely in most centers.
After a thorough evaluation, if no unexpected findings are encountered, preparation for liver transection begins. In all liver resections control of hepatic inflow structures at the hilum should be obtain such that, if required, a Pringle maneuver may be applied. For tumors in precarious locations, isolation and encirclement of hepatic veins should be considered, as rapid control may be obtained should it be required. For major resections, typically inflow is taken followed by outflow. The line of transection is marked on the liver and parenchymal transection initiated. Many different techniques and instruments have been described for division of hepatic tissue including clamp-crush, ultrasound or harmonic vibration, water jet, and ablative devices none of which is superior to another and use is typically dictated by surgeon preference. Regardless of instrument the goal of parenchymal transection is to safely and efficiently come through the liver tissue with minimal damage to the parenchyma that will remain. Transection should be cautious and all visible vessels and bile ducts formally secured. Blind coagulation of these structures increases the risk of postoperative bleeding and bile leak. At the completion of transection the specimen is checked to ensure grossly negative margins. The raw edge of the liver is assessed for hemostasis and biliostasis, the patient is resuscitated, and the abdomen closed. The evolution of these operative techniques combined with meticulous LCVP anesthesia and fastidious perioperative care, have significantly decreased the morbidity and mortality of liver resection, such that even large volume resection may safely be performed in experienced centers.
Synchronous Colorectal Liver Metastases
CRLM present at the time of primary diagnosis and/or those diagnosed within the following 12 months, are arbitrarily defined as synchronous. Patients presenting in this fashion may have less favorable disease biology and worse clinical outcomes [59, 60]. Simultaneous diagnosis of primary CRC and CRLM represents a unique, often challenging, clinical scenario requiring special consideration and multidisciplinary management [109]. In the setting of resectable synchronous CRLM three different surgical approaches have been described. The “classic” approach is most commonly employed and includes upfront resection of the primary CRC followed by subsequent liver resection at a later date. However, as morbidity associated with hepatectomy continues to decrease, there is increased enthusiasm for the use of a “combined” resection strategy, in which both the primary tumor and CRLM are removed simultaneously. Most recently, a third approach, referred to as the “reverse” or, liver first, approach has been described, in which hepatectomy precedes resection of the primary lesion. With the addition of chemotherapy, many permutations of these general approaches exist [110]. Each approach is associated with specific surgical and oncologic risks and benefits (Table 3). To date, no good clinical evidence exists to suggest superiority of one approach over the other [111].
Table 3
Comparison of surgical approaches for management of synchronous colorectal liver metastases
Approach | Advantages | Pitfalls |
---|---|---|
Classic (primary first) | – delivery of chemotherapy prior to liver resection is more reliable | – chemotherapy induced liver injury |
– test of time to assess tumor biology | – disappearing CRLM | |
– in vivo assessment of tumor responsiveness facilitating postoperative therapy | – progression of disease | |
– removal of the “source” of subsequent metastases | ||
Reverse (liver first) | – progression of disease unlikely | – primary CRC related complication (rare) |
– removal of “lethal” disease first | – decreased ability to deliver chemotherapy postoperatively | |
Simultaneous (combined liver and primary) | – single surgery | – relatively contraindication with major hepatic resection or low rectal resection (LAR/APR) |
– +/− reduced morbidity | – prolonged recovery may delay adjuvant chemotherapy |
Staged Resection Strategies
The “classic” or primary first approach is the most commonly employed. Typically patients undergo resection of their primary disease followed by some duration of adjuvant chemotherapy followed by hepatectomy. Proponents of this strategy suggest that it offers significant advantages including: (1) delivery of chemotherapy prior to liver resection ensuring all patients receive some treatment, (2) disease progression on chemotherapy identifies aggressive tumor biology and prevents unnecessary and morbid hepatic resection, (3) assessment of tumor responsiveness to chemotherapy prior to resection allows better selection of postoperative chemotherapy, and (4) resection of the primary removes the “source” of subsequent metastases. However, these assumptions for the most part are theoretical in nature and have not been substantiated. For instance, no prospective trial of neoadjuvant or perioperative chemotherapy in the setting of resectable CRLM has shown benefit in terms of overall survival; therefore, whether or not a patient receives, or does not receive, therapy may in fact be a moot point. Furthermore, in randomized trials of modern short course perioperative chemotherapy for resectable CRLM, disease progression is rare (7–8 %) [88, 112], as such identification of biologically aggressive disease is unlikely. Additionally, administration of chemotherapeutic agents such as oxaliplatin and irinotecan prior to liver resection may cause injury to the non-tumoral liver, and potentially increase the risk of subsequently hepatic resection [24]. This staged approach has been extensively evaluated and compared to the alternatives, and in some studies is has been associated with increased combined morbidity [113] while in others it has not [114]. Most importantly, to date, no differences have been observed in terms of long-term clinical outcomes [111]. At present no consensus exists regarding when and in whom this approach for synchronous CRLM is indicated.
In the past it was thought that resection of the primary tumor was essential to prevent complication (bleeding, perforation, obstruction), however, in the era of current combination chemotherapy regimens, these events are rare [115] and leaving the primary tumor in situ is acceptable and safe. Consequently, an alternative staged approach to synchronous CRLM has recently been described. This “reverse” or liver first approach entails resection of the CRLM followed by interval resection of the primary. The initial rationale of liver resection prior to primary resection stems from the belief that it is the burden of disease within the liver that dictates the development of subsequent metastases. Although commonly stated by proponents of a liver first approach, this popular theory lacks scientific evidence. With this strategy chemotherapy is typically given prior to liver resection. Consequently, it has been suggested as an approach well suited to rectal primaries, where evidence exists to support the use of preoperative chemoradiotherapy [116], and in the setting of more advanced CRLM [117] where reducing hepatic tumor burden may improve resectability of the CRLM. Recent systematic reviews evaluating the reverse approach in synchronous CRLM suggests that it is feasible and safe [118] and not inferior to other suggested management strategies [111].
Simultaneous Resection Strategies
Recent advances in perioperative care and surgical technique have reduced morbidity and mortality of both colorectal and liver surgeries, leading many to consider combined surgery in the setting of patients with resectable synchronous CRLM. Numerous retrospective studies published over the last two decades, support this shift in paradigm, and suggest that simultaneous colon and liver resection is not associated with increase morbidity when compared to either resection performed alone [114, 119]. In fact, given the need for a single operation, some suggest a reduction in cumulative morbidity when compared to staged interventions [113]. Simultaneous resection offers the benefits of a single operation without increased morbidity and is a viable option in the majority of patients with synchronous CRLM. There is however debate as to whether simultaneous resection should be undertaken in cases where major hepatectomy would be required to extirpate all disease. Investigations to date are all retrospective and the patients undergoing simultaneous procedures are highly selected with characteristically low volume, unilobar, CRLM [120]. Given this heavy selection bias towards minimal disease and minor resections the safety of simultaneous resection should not arbitrarily be extrapolated to all liver resections, but rather reviewed on a case by case basis. This approach is not appropriate for patients requiring emergency colorectal surgery for complications related to the primary, or in those with underlying liver dysfunction, poor functional status, or where concern regarding surgical margin exist. With the exception of performing major hepatectomy in combination with major pelvic surgery for low rectal cancer (i.e., abdominal perineal resection), in high volume centers, combined resection of synchronous CRLM is increasingly being employed and outcomes appear to be acceptable. Currently, outcomes in highly selected patients, undergoing simultaneous resection are similar to those observed with staged resections and in this setting appear safe and oncologically sound [111]. In general, we favor the combined approach for the great majority of cases. Cases requiring an extensive hepatectomy, especially when combined with complex pelvic resection, require special consideration and appropriateness is determined on a case by case basis after multidisciplinary review.
Multiple and/or Bilobar Colorectal Liver Metastases
The landscape of surgery for CRLM is rapidly changing and advancements in all areas of patient care and disease management continue to allow liberalization of the definition of surgically “resectable” CRLM. In the past, presence of four or more metastases, and/or bilobar disease distribution, were contraindications to resection. However, newer techniques and surgical adjuncts have resulted in a proportion of patients with multiple and/or bilobar disease distribution to undergo successful resection with 5 year disease-free survival (DFS) of up to 20 % and OS rates ranging from 33 to 50 % [18, 121]. Two basic approaches for surgical management in this group have been described, staged and parenchymal sparing. Staged resection typically consists of three phases; (1) clearing of the FLR of all disease, (2) PVE/PVL to induce hypertrophy of the FLR, and (3) interval hemi or extended major hepatectomy to remove all remaining disease. Depending on the center, chemotherapy may be given before, between, and/or after resections. The major limitation of this strategy is the need for multiple surgeries to achieve complete resection. It has been shown that between 20 and 50 % of patients fail to complete the second stage of resection and survival outcomes of this cohort are no different from those patients receiving palliative chemotherapy alone [122, 123]. These findings raise concern as to the regular use of this strategy as many patients are exposed to the potential harms of surgical intervention with no obvious benefit. However, failure to complete the second stage of two staged resection is reportedly lower (12–30 %) in modern series from specialized centers [124–126] and without this attempt there is no chance of complete resection. Conversely, parenchymal sparing surgery entails a single curative intent operation with treatment of all sites of disease using multiple segmental or sub-segmental resections WR with or without ablative techniques. Some studies suggest increase risk of liver recurrence with this approach as compared to more extensive resections. This may be due, in part, to the increased use of ablative therapies to achieve an RO resection, which has been associated with increased local recurrence when compared to resection [121]. However, this increase recurrence appears to be size dependent. A recent review of over 300 CRLM treated with ablation suggest that tumor size <1 cm is associated with 92 % local control rate at 2 years and is highly effective treatment for these lesions [127] Furthermore, it is of note that patients treated with parenchymal sparing techniques are inherently different, and clinical outcomes are not directly comparable to those in whom extensive resection achieves complete tumor removal. In general, if complete extirpation of disease can be achieved 5 year OS of 40–50 % have been observed [18, 121]. Regardless of approach, morbidity associated with both of these strategies is high but acceptable, and similar to other liver surgeries, morbidity remains low. Despite some limitations, in this cohort of patients with advanced CRLM, surgery is feasible however controversy exists as to the routine implementation and multidisciplinary planning is essential to optimize patient outcomes.
Chemotherapy for Resectable Colorectal Liver Metastases
Adjuvant Systemic Chemotherapy
Depending on selection criteria, approximately 70 % of patients undergoing complete resection of CRLM will experience recurrence. Half of these patients will have the liver as the only site of disease recurrence [128]. As such there is a strong rationale behind the desire to provide adjuvant systemic therapy in this setting with the goal of improving long-term outcomes. To date, several retrospective studies have suggested benefit of adjuvant systemic therapy. In a review of 792 patients by Parks et al. [129] adjuvant chemotherapy was associated with significant improvement in OS (p = 0.007) and was an independent predictor of outcomes for all categories of clinical risk. This finding was mirrored in a retrospective review by Figueras et al. [130], where patients receiving adjuvant chemotherapy had an associated longer OS (RR 0.3, p < 0.001). Despite impressive retrospective findings, similar outcomes have not been observed in randomized trials. To date, four trials of adjuvant systemic therapy alone in patients with resectable CRLM have been completed [131–134], all of which failed to show a significant benefit in terms of OS. In 2008, Mitry et al. published a pooled analysis of 278 patients with resectable CRLM from two randomized trials of 5FU based adjuvant systemic chemotherapy versus surgery alone and found no differences in terms of PFS or OS between the groups [135] Furthermore, recent randomized evidence published by Ychou et al., revealed no difference in DFS between patients randomized to receive adjuvant FOLFIRI versus those receiving 5FU/LV [134]. Direct evidence to support the use of adjuvant systemic chemotherapy for resectable CRLM is lacking and its usefulness in this setting is debated. Proponents of adjuvant therapy note several limitations to the interpretation of the aforementioned trial findings including, variability in the actual delivery of chemotherapy leading some trials to be underpowered, heterogeneity of baseline disease characteristics, and use of chemotherapy considered inadequate by current standards [128]. These limitations call to question the clinical applicability of these trial results in the modern era and use of adjuvant systemic therapy in patients with resectable CRLM is commonly employed.
Neoadjuvant Systemic Chemotherapy
Chemotherapy delivered prior to liver resection in patients with upfront resectable CRLM is controversial. Proponents of this approach suggest that neoadjuvant chemotherapy with modern drug combinations (5FU + Oxaliplatin/Irinotecan with or without targeted antibodies) has significant advantages, including; (1) facilitation of hepatic resection by reducing tumor size, (2) eradication of micrometastatic occult disease, (3) avoidance of unnecessary surgery for those who progress, (4) identification of tumor chemosensitivity prior to resection [128]. However, opponents suggest that administration of toxic chemotherapy in the setting of CRLM that are already resectable introduces the unnecessary risk of chemotherapy induced liver injury and increased perioperative morbidity [136]. Additionally, neoadjuvant therapy may result in “disappearance” of some tumors. This poses a significant challenge for surgeons, because although these tumors are not evident clinically, viable cancer cells are likely still present, and failure to resect these sites is associated with high rates of recurrence [137]. Furthermore, the argument that neoadjuvant treatment allows assessment of tumor biology is likely overstated. In a recent systematic review evaluating 23 different studies of neoadjuvant therapy in the setting of resectable CRLM median rate of disease progression while on treatment was 15 % (range 0–37 %) [138] whilst randomized trials of modern chemotherapy regimens found rates to be even lower (7–8 %) [88, 112] suggesting that the ability to select out “bad biology” with preoperative chemotherapy is uncommon. Neoadjuvant chemotherapy may also result in liver parenchymal injury that appears to portend higher postoperative morbidity [136, 139] Moreover, although tumor progression while on chemotherapy is associated with increased recurrence; there are conflicting data on the impact on OS and many series show no differences in long term outcome if completely resected [89, 140].
To date no randomized trial addressing neoadjuvant systemic chemotherapy alone for resectable CRLM has been completed. Its use has primarily been extrapolated from the initial findings of a single randomized trial of perioperative systemic chemotherapy (chemotherapy before and after resection). In this trial [112], Nordlinger et al. randomized 364 patients with resectable CRLM to surgery alone versus surgery + perioperative chemotherapy (six cycles preoperative 5FU-LV/oxaliplatin FOLFOX and six cycles FOLFOX postoperatively) with a primary end point of progression-free survival (PFS). Intent to treat analysis revealed a borderline absolute improvement in PFS of 7.3 % at 3 years in the treatment arm. Given these findings, use of chemotherapy perioperatively for resectable CRLM was considered standard practice. More recently, however, the long-term results of this trial were published [139]. No difference in median OS was observed between study arms at 8.5 years of follow-up (61.3 months (95 % CI 51.0–83.4) perioperative chemotherapy group versus 54.3 months (41.9–79.4) in the surgery-only group, p = 0.34). The authors suggest that despite the lack of OS benefit, the improvement in PFS with perioperative FOLFOX is evidence enough to warrant perioperative treatment in patients with CRLM.
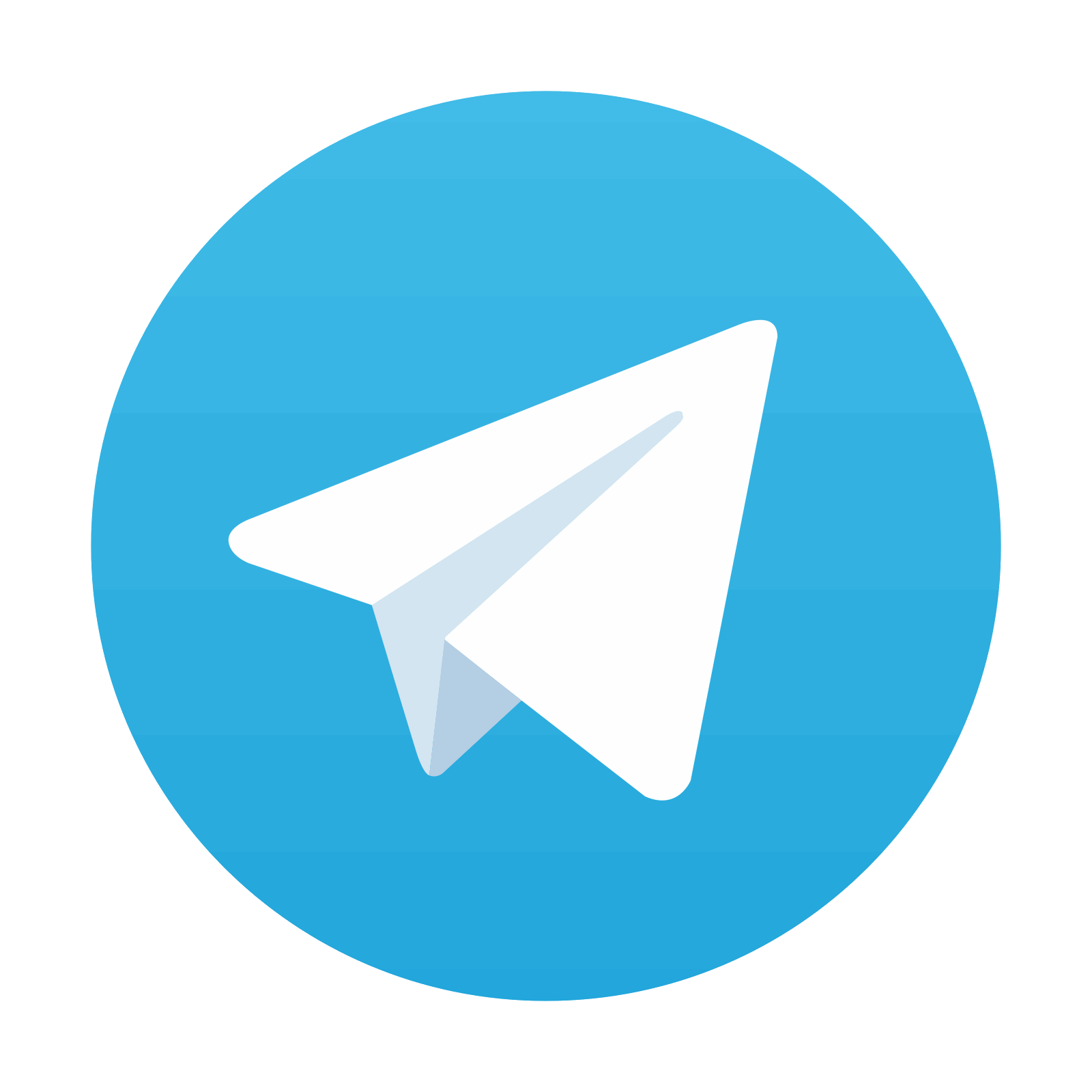
Stay updated, free articles. Join our Telegram channel

Full access? Get Clinical Tree
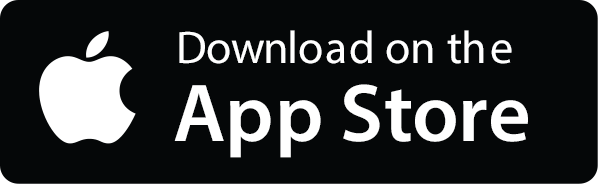
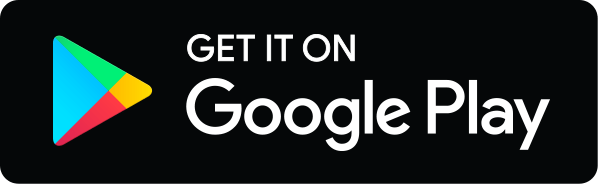