Fig. 15.1
The blood capillary endothelium and the cellular-interstitial matrix are major barriers to efficient drug transport. When compared with the mesothelium, the interstitium and capillary barriers play greater roles in possessing insignificant barrier properties (Adapted from Intraperitoneal delivery of nanoparticles for cancer gene therapy (2013), Hallaj-Nezhadi S et al. [6])
Keeping high and long-lasting local drug concentration is necessary for successful and efficient IP therapy [7]. Drugs with a small molecular weight (<20 kDa) enter circulation through peritoneal capillary absorption. The drug is then quickly removed from circulation, and its residence time in the peritoneal cavity is not sufficient to get either high or long-lasting drug concentrations. In order to get satisfactory cytotoxicity, frequent or continuous dosing is required. However, this increased frequency can lead to catheter-related problems, increased risk of infection, and bowel complications in patients. Small molecular weight drugs do show systemic circulation. Pharmacokinetic studies in animals have shown that IP taxane (docetaxel or paclitaxel) was quickly cleared within 24 h from the peritoneal cavity. In addition, many free drugs are usually coupled with severe side effects. For example, Cremophor EL (Cr-EL) and dehydrated ethanol are usually used to increase paclitaxel solubility to get solvent-based PTX (Sb-PTX: Taxol®). Due to the large amount of Cr-EL added as well as the nonspecific drug biodistribution in other healthy organs, Sb-PTX has been reported to have moderated antitumor efficacy and severe side effects including hypersensitivity reactions, bone marrow suppression, and neurotoxicity.
With this in mind, nanoparticle albumin-bound paclitaxel (nab-paclitaxel, Abraxane®) [9] has been designed to address the aforementioned problems. Since it is an albumin-bound, 130-nm particle, neither ethanol nor Cr-EL is required. In animal models, Abraxane exhibited superior antitumor advantages and a more favorable safety profile when compared to free PTX. In the clinic, a randomized Phase II study investigated the overall response and the disease control rates for unresectable or recurrent gastric cancer patients treated with nab-paclitaxel. Results indicated responses of 27.8% and 59.3%, respectively [10]. Interestingly, one patient had a response rate of 100%. The median progression-free survival was 2.9 months, and overall survival time was 9.2 months. Recently, Kinoshita et al. [11] evaluated the therapeutic efficacy of nab-paclitaxel and free drug Sb-PTX on gastric cancer cell-bearing nude mice xenografts. Using this peritoneal metastatic xenograft model, nab-paclitaxel showed greater efficacy than Sb-PTX at equal doses when given as an IP injection. Compared with IP Sb-PTX, nab-paclitaxel treatment exhibited a better tumor suppression on both subcutaneous tumor size and ascites burden (p < 0.05).
Recently, thermosensitive hydrogel has attracted attention as a drug delivery method since it is a stimuli-responsive material. This is particularly true for local region administration [12]. At specified temperatures, thermosensitive hydrogel undergo a sol-gel transformation. Moreover, thermosensitive hydrogels are easy to load either with hydrophilic or hydrophobic drugs. This loading occurs with high loading efficiency, and the gel allows for controlled drug release behavior. In addition, thermosensitive hydrogels are easily acceptable to patients because they exist in one state when the temperature is lower than the sol-gel transition temperature [13] (Fig. 15.2).


Fig. 15.2
(a) Thermosensitive gel is liquid at 4 °C. (b) Gelation at 37 °C. (c) Thermosensitive gel is easily injectable through a 26-gauge needle. (d–e) Thermosensitive gel is flexible at 37 °C. Reproduced with permission from Ref. [13]
In order to treat peritoneal dissemination of gastric cancer, Bae et al. [14] prepared a thermoresponsive hydrogel based on poloxamer and linoleic acid-coupled Pluronic F127 (Plu-CLA). At room temperature, Plu-CLA exists in a liquid state, but is rapidly converted to a gelatin state at body temperature. Docetaxel was successfully encapsulated in the Plu-CLA and exhibited a controlled release profile. Intraperitoneal administration of docetaxel-Plu-CLA (Doc-Plu-CLA) showed better antitumor advantages than free drug administration, as evidenced through induction of apoptosis and a reduction in the number of peritoneal metastatic nodules. In addition, the Doc-Plu-CLA-treated peritoneal gastric cancer xenograft mice had the longest median survival time (Fig. 15.3). Taken together, these results show that IP Doc-Plu-CLA administration significantly inhibits peritoneal metastasis and prolongs survival in a xenograft mouse model of gastric cancer.


Fig. 15.3
18F-FDG PET image of mice with peritoneal metastases. (a) Tumoral 18F-FDG uptake (circle) based on microPET images. (b) Comparison of 18F-FDG SUV uptake in the control, docetaxel- and Doc-Plu-CLA-treated groups. *p < 0.05. Reproduced with permission from Ref. [14]
As a local treatment option, photodynamic therapy (PDT) consists of activating a photosensitizing agent using a specific laser wavelength [15]. Since photosensitizing agents allow for accumulation specifically at tumor sites, PDT showed fewer side effects and reduced damage to normal tissue. When compared with either radiation or chemotherapy, PDT also rarely induced drug resistance. Due to the above advantages, PDT has been widely used to treat gastric, breast, and lung cancers, among other diseases. Tsujimoto et al. [16] prepared indocyanine green (ICG) derivatives-loaded nanoparticles and ICG-loaded lactosomes (ICGm) in order to investigate their PDT theranostic value in the mice model of experimental peritoneal dissemination of gastric cancer. After photodynamic therapy, the median survival time in ICGm- and ICG-treated mice was 32 days and 17 days, respectively. Moreover, body weight loss in ICG-treated group was significantly greater than that in ICGm-treated mice (p < 0.05). This result was taken as an indication of the safety of ICGm treatment.
15.3 Intratumoral Delivery of Therapeutic Agents
Local intratumoral delivery of chemotherapeutic agents is likely to provide better drug localization within the targeted tumor, thereby reducing systemic exposure to healthy organs. This would lead to increased efficacy and lower toxicity than treatment with aqueous, free drug solutions. To this end, Al-Abd et al. [17] prepared an injectable, thermosensitive hydrogel to deliver the anticancer drug doxorubicin (DOX). During their experiment, 0.6% of DOX was loaded into a 10% reversible thermal poly(organophosphazene) (PPZ) hydrogel that was capable of body temperature-dependent transformation. An in vitro release study showed that a initial burst drug release in the first few hours after administration. However, DOX was released in vivo in a controlled and sustained manner over a 5-week period. The hydrogel mass was not completely degraded over 7 weeks (Fig. 15.4). It should be noted that an initial burst effect is beneficial for fast control over tumor growth, with the subsequent sustained release ensuring long-lasting tumor control. The PPZ hydrogel was then given intratumorally in a human gastric tumor xenograft mice model. In this case, the tumor T1/T2 for locally and systemically administered DOX was 2.6 days to 4.6 days, respectively, showing successful increase in local drug retention. Moreover, the data suggest that the hydrogel decreased systemic exposure and cardiac toxicity. The longer tumor DOX exposure levels obtained in the hydrogel delivery system mean better antitumor efficacy. After a single intratumoral administration, the DOX hydrogel formulation controlled gastric cancer size for up to 49 days without significant signs of toxicity.


Fig. 15.4
DOX-loaded hydrogel contacted the tumor as a mass with a well-defined margin. Reproduced with permission from Ref. [17]
Combination chemotherapy has become an important option for advanced gastric cancer treatment. Coadministration of DOX and PTX formulations using PPZ thermosensitive hydrogel has been assessed for the in vivo antitumor efficacy in local tumor management in human gastric cancer cell-xenografted mice [18]. Following intratumoral injection of PPZ into human SNU-601 gastric cancer cell-bearing mice, the combined DOX (15 mg/kg) and PTX (30 mg/kg) containing hydrogel resulted in the highest tumor inhibition in the tested experimental groups. The PTX-DOX hydrogel was injected intratumorally and gelated within the tumor site. PPZ hydrogel treatment exhibited no drug-related adverse effects and no mortality for 97 days. In comparison, the mortality rates in the PTX-DOX solution intratumoral and intravenous groups are 5/8 and 4/9, respectively. These results demonstrate that sustained release of a combined DOX and PTX treatment yielded a reduction in drug-induced toxicity.
Liposomes have been reported to successfully delivery a wide range of drugs. A large amount of evidence has shown that drug-loaded liposomes are more advantageous than free drug in regard to cytotoxic and safety considerations. To this end, the antitumor effects of intratumoral docetaxel-loaded immuno-(trastuzumab)-liposomes (IDL) were evaluated in a local, clinical application of trastuzumab against NCI-N87 Her2/neu-overexpressing gastric cancer xenograft mouse model [19]. In this study, the liposome diameter was approximately 100 nm, as it has been reported that this size is more favorable for tumor uptake and retention time [20]. They also suggested that smaller liposomes may have greater surface-to-surface contact with the cell membrane. The NCI-N87 gastric cancer xenograft mice were treated with either IDL or docetaxel-loaded liposomes. When compared with docetaxel treatment alone, docetaxel-loaded liposomes, or the combined docetaxel/trastuzumab treatment, the intratumoral IDL-treated group exhibited higher drug concentration at the tumor site. Moreover, this treatment group also had far better antitumor efficacy in the N87 xenograft model. Intratumoral administration of either free trastuzumab or IDL significantly suppressed tumor cell growth without evidence of severe side effects. According to their study, intratumoral IDL administration resulted in a high docetaxel concentration in the tumor region and has great potential for use as a safe and effective local cancer therapy. It was also noted that the liposome delivery formations prolonged therapeutic retention time. Collectively, the docetaxel-loaded liposomes conjugated with trastuzumab exhibited several antitumor advantages, including [1] prolonged liposome-docetaxel retention time within tumor sites and [2] liposome promote trastuzumab to accumulation in tumors with no sign of decline. Furthermore nanoparticle formations could decrease the severe skin ulcerations resulting from docetaxel treatment. In this study, percutaneous injection of free docetaxel into the tumor sites resulted in severe skin ulceration in one-third (2/6) of mice. On the contrary, treatment with either DL or IDL did not result in any skin ulcerations. Thus, it is shown that docetaxel-loaded liposome formations may reduce the occurrence rate and severity of normal docetaxel side effects.
Nanoparticles have been explored to deliver their payloads at the local tumoral site and minimize systemic exposure. Previously, we prepared the paclitaxel (PTX) and berbamine (BA) co-deliver nanoparticles using methoxy poly (ethylene glycol)-polycaprolactone (mPEG-PCL) to [21]. This formulation allowed for both high encapsulation efficiency and controlled release at the tumor site. Intratumoral administration showed that when compared to free drug administration, PTX/BA-NP exhibited superior antitumor effects when delivered intratumorally in a human gastric cancer mouse model. This was evidenced by inhibition of tumor growth.
In addition to “passively” targeted nanocarriers, more and more “actively” targeted nanomedicines have been developed to improve therapeutic properties. Among them, stimulus-responsive drug delivery systems have significant benefits. These delivery systems are triggered upon exposure to a specific environmental condition, such as temperature, magnetic field, presence of tumor matrix metalloproteinases (MMPs), or low pH. Such stimulus-responsive nanomedicines accumulate within tumors via EPR effects, are transformed, and release their payloads under the influence of external impacts or conditions of the tumor microenvironment. Such a triggering mechanism might overcome transport barriers, decrease drug resistance, and allow for more controlled drug release. MMPs are highly expressed in various types of tumor tissues and play an important role in tumor invasion, metastasis, cancer stem cells, and drug resistance. The conjugation of polyethylene glycol (PEG) to nanoparticles, polymeric micelles, or liposomes can improve biocompatibility and prolong their time in blood circulation. However, it has been shown that PEGylation severely reduces their cellular uptake. To overcome this limitation, Park et al. developed a PEG-peptide-quantum dot (QD) that contained an MMP-2 cleavable peptide sequence. With this formulation, they showed that tumoral enzymatic dePEGylation effects improved intracellular drug delivery.
In a separate study, an antigen-binding fragment of an anti-MMP antibody was conjugated to doxorubicin-loaded liposomes via a PEG spacer. This approach showed enhanced tumor cell uptake and greater suppression of tumor growth in a cancer mouse model. In our previous studies, we have successfully synthesized PEG-PCL nanoparticles containing gelatinase-sensitive peptide. In the gelatinase (MMP2/9)-rich environment presented by gastric cancer tissue, nanoparticles have been shown to accumulate in both a targeted and effective manner. Moreover, nanoparticles provide a preferable platform for the co-delivery of different hydrophilic/hydrophobic agents including chemotherapeutics, nucleic acids, and small molecules of anti-gastric cancer activities, such as docetaxel [22], miR-200c [23], salinomycin, 5-aza-2′-deoxycytidine, and tetrandrine. Consequently, this kind of nanoparticles may also be used as a platform for local and regional delivery of therapeutic agents for the goal of tumor inhibition. We have also used this intelligent carrier to deliver a traditional medicine monomer evodiamine (EVO) [24]. These EVO-NPs were then intratumorally injected into tumor-bearing mice. Subsequent in vitro cellular uptake studies revealed gelatinase-stimuli nanoparticles could more easily enter the cytoplasm due to their hydrophobicity. Moreover, real-time in vivo nanoparticle biodistribution demonstrated that intelligent EVO-NPs could both efficiently accumulate and retain in the local tumor regions. Therefore, EVO-NPs showed higher tumor suppression and reduced side effects when compared to freely administered EVO (p < 0.01).
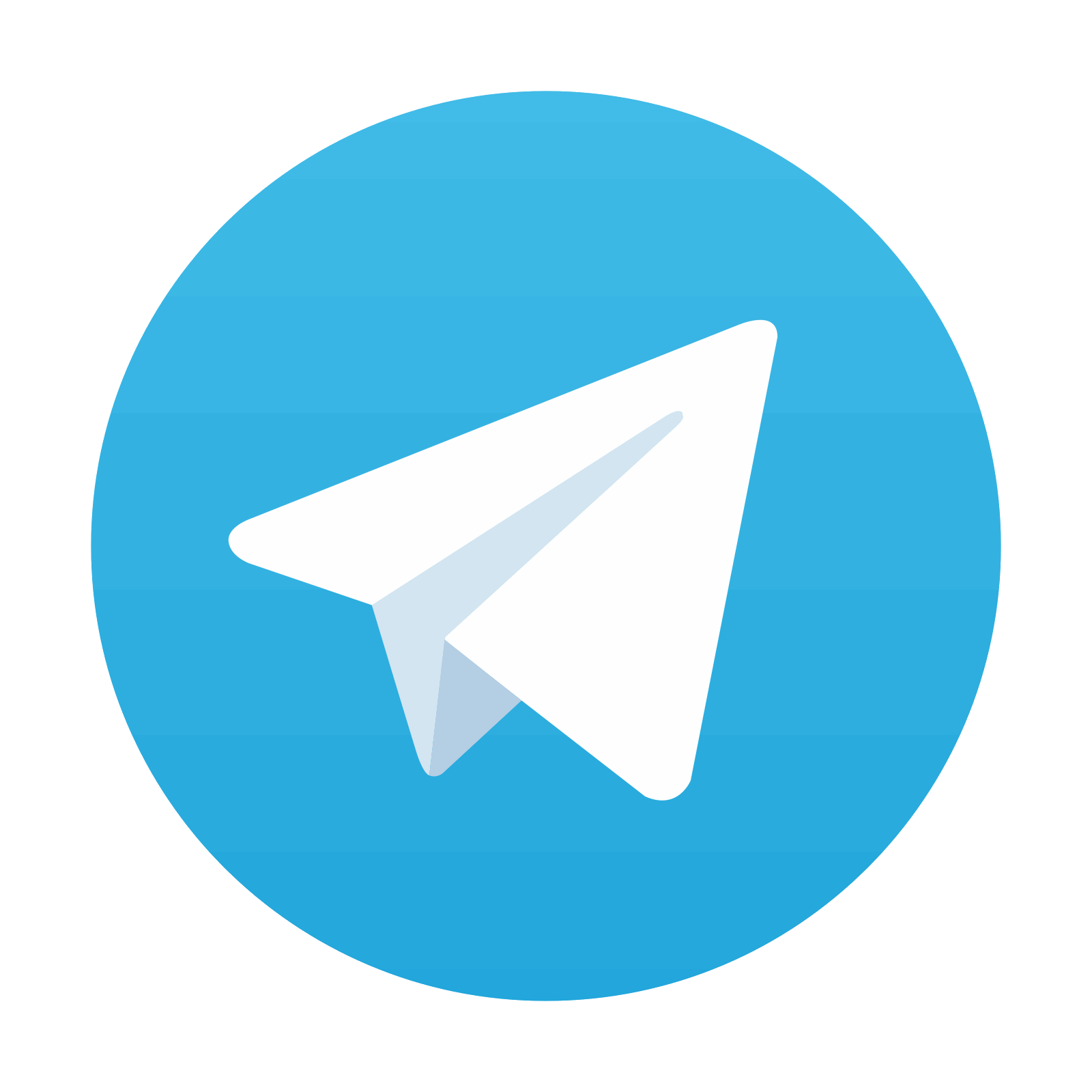
Stay updated, free articles. Join our Telegram channel

Full access? Get Clinical Tree
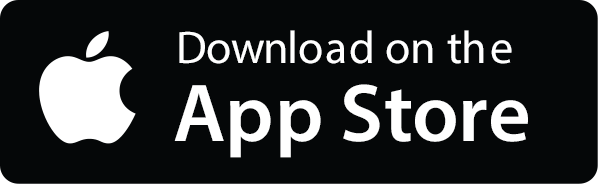
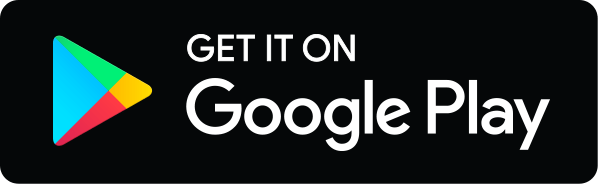