Fig. 5.1
Liquid biopsy in pancreatic cancer. Tumor-specific alterations can be detected in circulating tumor DNA (ctDNA) in blood. Detecting ctDNA in plasma or serum serves as a “liquid biopsy,” which would be useful for a variety of clinical applications for pancreatic cancer
5.2.2 Origin of cfDNA
It has been suggested that cancer patients have higher levels of cfDNA than healthy individuals [6]. Levels of cfDNA are also increased in a variety of other physiological and pathological conditions, including exercise, inflammation, smoking, sepsis, and trauma [7]. cfDNA is shed into the bloodstream via apoptosis, necrosis, direct release from viable cells, and lysis of circulating cells, but the major sources are now thought to be apoptotic and necrotic cells. In fact, the length of cfDNA fragments in the circulation often shows a characteristic laddering pattern with multiples of 170–180 base pairs, which is a well-known feature of apoptosis [6]. Apoptosis is programmed even for many normal cells on a daily basis. It has been suggested that a large fraction of cfDNA is derived from bone marrow and liver in healthy individuals [8]. But, in a tumor mass, hyperproliferation and rapid cellular turnover of cancer cells can lead to increased programmed cell death. Intratumoral microenvironments such as hypoxia may also lead to necrosis. Cellular debris of apoptotic or necrotic cells is normally phagocytozed by infiltrating macrophages and the cellular components are cleared. However, this clearance mechanism does not proceed effectively in a tumor mass, leading to the accumulation of cellular debris, including DNA, and its release into the circulation of patients.
5.2.3 cfDNA as a Biomarker for Cancer
Although cfDNA is generally increased in patients with cancer, its sensitivity and specificity for cancer detection are low, and the utility of total cfDNA level as a cancer biomarker is questionable. On the other hand, tumor DNA can be discriminated from normal cfDNA by detecting tumor-specific somatic mutations that exist only in the genomes of cancer cells or precancerous cells, but not in the genomes of matched normal cells. This assures the specificity of ctDNA as a cancer biomarker.
However, detection of ctDNA has been challenging, since the percentage of ctDNA is very low (<1.0% in many cases) in total cfDNA. Traditional sequencing methods such as Sanger sequencing or pyrosequencing can detect mutations of tumor-derived DNA fragment only in patients with a high tumor burden and a large amount of ctDNA. However, recent advances in sequencing technologies, including digital polymerase chain reaction and next-generation sequencing, have made it possible to detect ctDNA present at relatively low frequencies in blood, and there has recently been an explosive increase of studies to detect ctDNA in blood from patients with cancer and to investigate the clinical utility of ctDNA.
5.2.4 Methods for Detection of ctDNA
Digital polymerase chain reaction (dPCR) is now one of the major methods to sensitively detect genomic alterations in cfDNA. In 2003, a PCR-based digital approach, named BEAMing (Beads, Emulsion, Amplification, and Magnetics), was described [9]. Using emulsion PCR and flow cytometry, BEAMing can efficiently identify rare mutations with allele fractions as low as 0.01%.
Nowadays several dPCR systems, including droplet-based platforms, are commercially available. Generally, the sensitivity of a droplet dPCR system depends on the number of droplets. One of the most widely used droplet dPCR devices, QX200 Droplet Digital PCR System (Bio-Rad Laboratories) generates 20,000 nanoliter-sized droplets. The RainDrop Digital PCR System (RainDance Technologies) can perform “single-molecule” PCR in up to ten million picoliter-sized droplets, and therefore, possesses very high sensitivity. In addition, multiplex assays are possible in the RainDrop system by using combinations of two color probes at different concentrations (up to ten targets) [10].
Next-generation sequencing (NGS) is also widely used to analyze genomic alterations in cfDNA. Unlike dPCR, NGS techniques can analyze multiple, broad regions of interest. Even whole-genome sequencing or whole-exome sequencing of cfDNA from advanced cancer patients has been reported, and various alterations, including single nucleotide variants (SNV), copy number alterations (CNA) and structural alterations of DNA, were detected. However, only genomic alterations with high allele frequencies may be detectable with these platforms, since deep genome-wide analysis, especially whole-genome sequencing, is quite costly and not feasible in the routine clinical context. Therefore, these global genomic analyses can be applied for only cfDNA samples from advanced cancer patients with high tumor burden. On the other hand, targeted sequencing can be performed at relatively low cost. By focusing on clinically important genes, mutations can be detected with higher sensitivity compared to the genome-wide analyses.
Amplicon sequencing is one of the major techniques for analyzing mutations in specific genomic regions. Ion AmpliSeq Technology (Thermo Fisher Scientific) is a widely used targeted sequencing platform. Highly multiplex PCR followed by NGS, such as Ion PGM, allows deep sequencing of target regions from as little as 10 ng input DNA at low cost and with a short turnaround time. However, the Ion Ampliseq system has some issues such as relatively high error rate and false-negatives in detection of small insertions and deletions (indels).
Target enrichment techniques, target capture-based platforms, are widely used for analyzing gene alterations of cancer. In principle, fragmented genomic DNA is hybridized with DNA/RNA probes designed for capturing targeted regions, and the enriched DNA libraries are analyzed by NGS. The SureSelect Target Enrichment System (Agilent Technologies) is widely used for targeted sequencing in combination with the Illumina paired-end sequencing platform, which has quite a low error rate among high-throughput sequencing instruments. Although the manufacturer’s protocol for the SureSelect Target Enrichment System requires at least 200 ng of input DNA, the amount of input DNA can be reduced by using particular library preparation kits, such as KAPA Hyper Prep Kit (KAPA Biosystems) [11].
In addition to these commercially available technologies, various highly sensitive sequencing methods have been developed for detecting ctDNA. In an amplicon-based system, Safe-SeqS (Safe-Sequencing System), individual DNA molecules are tagged with a unique identifier, then amplified and sequenced. According to the original paper, the error rate could be lowered to 9 × 10−6 by taking into account the unique identifiers [12]. Forshew et al. reported a method termed Tam-Seq (Tagged-Amplicon deep Sequencing) in 2012. They detected somatic mutations in cfDNA at 2% allele frequencies [13]. In the case of non-small cell lung cancer, another method for profiling ctDNA, CAPP-Seq (Cancer Personalized Profiling by deep Sequencing), has been described [14]. In addition to methods for detecting SNVs, PARE (Personalized Analysis of Rearranged Ends) identifies cancer-specific genome rearrangements, and it has been shown that these alterations can be used as personalized cancer biomarkers [15].
5.3 Liquid Biopsy in Pancreatic Cancer Diagnosis
The clinical utility of ctDNA has been investigated in various types of cancer. For cancer diagnosis, the most commonly mutated genes are probably best suited for analysis as blood-based biomarkers. However, even within a single tumor type, the mutation profile generally varies from patient to patient. Even if a single gene is commonly mutated in a particular cancer type, the altered loci can vary, especially in tumor suppressor genes such as TP53. For this reason, among others, it is not easy to utilize tumor-derived DNA in plasma for diagnosis of many cancer types without information about mutations in the tumor tissues.
On the other hand, point mutations of KRAS are commonly observed in PDAC and 90% of all KRAS mutations occur in codon 12 or 13 in PDAC. A number of studies have, therefore, investigated KRAS mutations in cfDNA from PDAC patients, although detection methods are diverse. To date, many studies have confirmed that mutant KRAS can be detected in plasma or serum from patients with PDAC.
In the 2000s, several research groups investigated the potential use of KRAS mutation in cfDNA as a biomarker of pancreatic cancer. Some of them found that mutant KRAS is more frequently detected in blood of PDAC patients than in that of chronic pancreatitis patients [16, 17]. It has also been suggested that sensitivity and specificity for detection of PDAC can be improved by combining detection of KRAS mutation in blood with detection of increased CA19-9 level [16, 17]. Furthermore, it has been reported that the presence of mutant KRAS in the circulation is associated with poor prognosis of patients with pancreatic cancer [11, 18–21]. Thus, KRAS mutant cfDNA could be useful as a predictive biomarker in patients with pancreatic cancer.
One of the major potential applications of ctDNA is disease monitoring. Tjensvoll et al. reported that changes in mutant KRAS levels in the circulation were correlated with radiological imaging data and CA19-9 levels during the course of chemotherapy [20]. They suggested that detection of KRAS mutation in plasma could be useful for monitoring treatment efficacy and tumor progression in pancreatic cancer patients.
It is noteworthy that various other cancer-related genes are mutated at relatively low frequencies in PDAC. Importantly, it has been indicated that 20% of patients with pancreatic cancer have somatic alterations in genes that are potential targets of therapies approved by the U.S. Food and Drug Administration (FDA) for oncologic indications or therapies in published prospective clinical studies [22]. This suggests that genomic profiling in pancreatic cancer could be useful to design precision treatment strategies. Due to improvements of sequencing technologies, global or highly multiplexed genomic analysis of ctDNA is becoming feasible using NGS. Analyzing ctDNA has also been proposed as an alternative method to tissue biopsy in the setting of precision medicine. Investigating mutations of actionable genes in cancer cells is essential for precision medicine. Although tumor tissue biopsies are generally used for molecular screening of cancer, it may be difficult in some patients to obtain sufficient amounts of tissues with a high tumor fraction. Indeed, obtaining adequate biopsy tissues for molecular diagnosis is often difficult in pancreatic cancer patients. Most importantly, tissue biopsies are invasive and are therefore not without clinical complications. Zill et al. analyzed 54 genes in tumor tissues and cfDNA samples using a commercially available gene panel and demonstrated that a large proportion of mutations detected in tumor biopsies can also be detected by sequencing of cfDNA in pancreatic and biliary cancer [23]. Although 35% of patients had an insufficient quantity or quality of tissue biopsy sample for sequencing analysis in their cohort, sequencing of cfDNA identified somatic mutations in many of these patients. We have also reported targeted deep sequencing analysis of cfDNA using a modified SureSelect-Illumina platform and an original gene panel for pancreatic cancer [11]. Our gene panel consisted of 60 genes, including 17 potentially actionable genes. As prescreening for sequencing analysis, dPCR assay was firstly performed to determine the mutational status of KRAS in plasma cfDNA of 259 patients with PDAC. We then carried out targeted deep sequencing in 48 patients including 43 cases that were considered to have ≥1% tumor DNA in total cfDNA based on dPCR KRAS assay and 5 cases with obvious distant organ metastasis, even though they were negative for KRAS mutation in plasma on dPCR assay. We found somatic mutations in potentially targetable genes in 14 of 48 patients (29.2%). In addition, we analyzed somatic copy number alterations using targeted sequencing data of cfDNA, and potentially targetable gene amplifications, such as CCND1 and ERBB2, were also detected. Thus, previous studies indicate that liquid biopsy has great potential for diagnosis and treatment design in pancreatic cancer in diverse clinical settings.
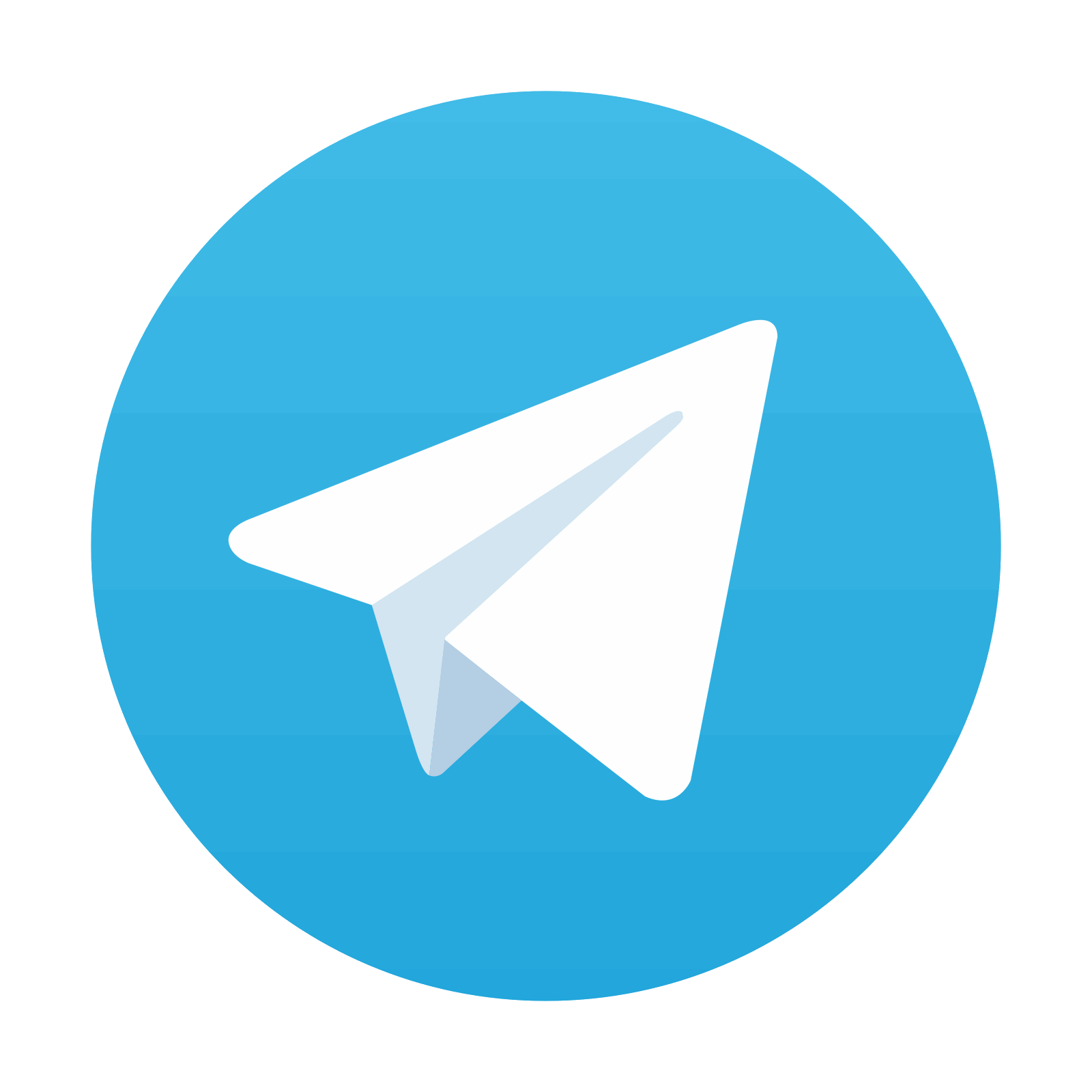
Stay updated, free articles. Join our Telegram channel

Full access? Get Clinical Tree
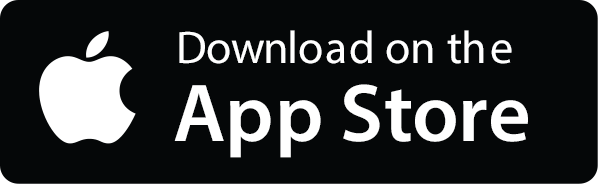
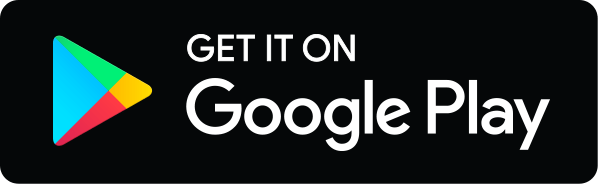