Fig. 1
The KD alters overall gene expression to more closely resemble that seen in normal brain. Total cellular RNA was isolated from the tumor and the non-tumor containing contralateral side of the brain. Gene expression was analyzed using Affymetrix GeneChip® Mouse Genome 430 2.0 arrays (Affymetrix, Santa Clara, CA). A two-way ANOVA for interaction showed that the data from the tumor sample obtained from mice fed a SD are clearly separate from the data obtained from the other three conditions. This analysis implies that the KD is driving the overall gene expression in the tumor to be more normal, that is, to be more like gene expression seen in the non-tumor containing tissue. Reprinted from (Stafford et al. 2010)
5 Growth Factor Signaling
A number of growth factor signaling pathways are critical to the formation and progression of malignant brain tumors. Insulin-like growth factor 1 (IGF-I) is one such growth factor that supports the growth of the number of cancers including brain tumors (Arcaro 2013; Haisa 2013; Hummel et al. 2013; Negi et al. 2013; Singh et al. 2014; Weroha and Haluska 2012). We have shown that IGF1 expression is markedly reduced in tumors from mice fed a KD compared to those fed a standard diet (Fig. 2). Similar results have been found by others using caloric restriction in a variety of mouse models of malignant brain tumors (Marsh et al. 2008; Seyfried et al. 2003; Shelton et al. 2010). cDNA array analysis also showed a reduction in the expression of RAS p21 protein activator 1 and mitogen-activated protein kinase 8 (c-Jun N-terminal kinase, JNK) in tumors from animals fed a KD (Scheck et al. 2012). These proteins participate in the platelet-derived growth factor and epidermal growth factor receptor tyrosine kinase signaling pathways, suggesting that the KD may act as a “pan-growth factor inhibitor.”
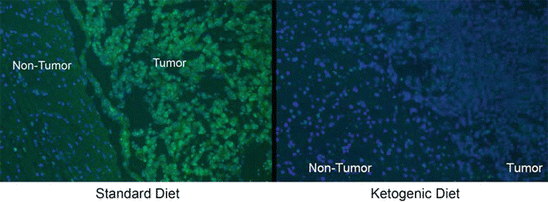
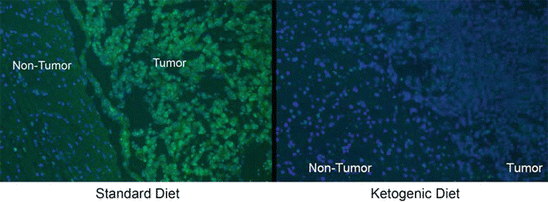
Fig. 2
Immunohistochemical analysis of Insulin Growth Factor 1 (IGF1). There is an increase in the expression of IGF1 in tumor tissue relative to non-tumor in animals fed a standard diet. In animals fed a ketogenic diet, the IGF1 expression in tumor tissue is reduced to the level of the adjacent non-tumor tissue. Reprinted from (Scheck et al. 2012)
Growth factor pathways also intersect with metabolism through the PI3K/Akt pathway which can be activated by a number of receptor tyrosine kinase growth factor pathways or activated Ras (Cantor and Sabatini 2012). The PI3K/Akt pathway is also closely linked to glucose metabolism and has been called the “Warburg kinase” (Robey and Hay 2009). Recent studies have shown that the action of Akt is fairly complex and may have different effects on tumor cell survival and growth depending on the genetic background of the cell (such as EGFR amplification, etc.), glucose and oxygen availability, therapy, and other environmental stimuli (Chautard et al. 2010; Elstrom et al. 2004; Eyler et al. 2008; Fan and Weiss 2010; Gallia et al. 2009; Li et al. 2009; Los et al. 2009; Marsh et al. 2008; Rao et al. 2005; Robey and Hay 2009; Vadlakonda et al. 2013). A complete discussion of the role of Akt, HIF-1, and other genes in tumor growth and metabolism is outside the scope of this chapter, but readers are referred to the cited review papers.
6 Reactive Oxygen Species
While the mechanisms underlying the anticancer effects of the KD are not completely understood, the literature regarding the KD in epilepsy has provided some insight. A number of these studies have involved the putative role of changes in the level of reactive oxygen species (ROS) and seizure control. ROS are involved in a variety of cellular processes including autophagic/apoptotic responses to genotoxic stress, pathways involved in the regulation of inflammation, response to hypoxia, and nutrient deprivation—to name a few. Rho and colleagues have shown that ROS production in the brain is reduced in animals fed a ketogenic diet (Kim and Rho 2008; Maalouf et al. 2007). Cancer cells often have increased levels of ROS resulting from a variety of intrinsic and external sources including mitochondrial alterations (Liang and Grootveld 2011), aberrant expression of components of cellular antioxidant systems, chronic inflammation, tobacco, viruses, and environmental pollutants to name just a few (Gupta et al. 2012; Weinberg and Chandel 2009; Fruehauf and Meyskens 2007). They regulate vascular endothelial growth factor (VEGF) and HIF-1 (Weinberg and Chandel 2009) and thus have been implicated in angiogenesis and tumor growth. We have demonstrated a reduction in ROS in tumors from mice fed a KD and changes in the expression of genes involved in oxidative stress pathways (Stafford et al. 2010). Amigo and Kowaltowski (2014) described a similar effect using CR. While the specific downstream effects of this have not yet been fully elucidated, alterations in tumor ROS levels are sure to have profound effects on tumor growth.
7 Anti-angiogenic Effects
One major hallmark of brain tumors is the rapid stimulation of blood vessels that supply the nutrients needed to sustain rapid cellular growth. This vessel growth is favored by the uncontrolled production of angiogenic stimulators and the absence of inhibitors. Vascular endothelial growth factor (VEGF) is considered a driving factor in angiogenesis and has thus become a prime target for anti-angiogenic therapy (El-Kenawi and El-Remessy 2013). To this end, the FDA approved bevacizumab, a monoclonal antibody targeting VEGF, for use in GBMs. While this drug may help to reduce edema, especially following radiation, it often results in adverse effects and it affords little if any improvement in overall survival (Field et al. 2014; Patel et al. 2012).
Studies as far back as 1914 have suggested that restricted food intake can target tumor blood supply and reduce tumor growth (Rous 1914). More recently, it has been suggested that caloric restriction (CR), which also reduces blood glucose and raises blood ketones, reduces growth and angiogenic biomarker expression in prostate cancer and breast cancer (De Lorenzo et al. 2011; Mukherjee et al. 1999; Phoenix et al. 2010; Thompson et al. 2004). Seyfried and colleagues recently showed that CR promoted vessel maturation by preventing vascular VEGF signaling in the CT-2A mouse astrocytoma model (Urits et al. 2012), and they have demonstrated reduced angiogenesis in a number of other mouse glioma models using caloric restriction (Mukherjee et al. 2002, 2004; Seyfried et al. 2011; Shelton et al. 2010; Zhou et al. 2007). Further, CR was shown to normalize a number of factors involved in tumor vessel instability and weakness (including VEGF) as well as reducing peritumoral edema in a mouse model using human U87 glioma cells (Jiang and Wang 2013). In the GL261 mouse glioma model, we found that when fed ad libitum the KD decreased tumor vasculature, reduced peritumoral edema, and altered the expression of genes involved in angiogenesis (Woolf et al. 2015), despite the fact that the expression of VEGF was unchanged (Scheck et al. 2012). Taken together, these results suggest that another effect of metabolic therapy may be to target angiogenesis activity, thus mimicking the beneficial effects of bevacizumab.
8 Anti-inflammatory Effects
The blood vessels formed by rapid angiogenesis in gliomas are often leaky, leading to peritumoral inflammation and edema. Inflammation can also be increased by treatment such as radiation therapy. Inflammation and edema can promote tumor growth, and reduce patient quality of life due to increased pressure-related symptoms and side effects of the high-dose steroids often used for treatment. We have shown that increasing blood ketones affects a number of tumor-related gene networks and alters the expression of genes involved in the cellular response to oxidative stress in tumor tissue, notably cyclooxygenase 2 (COX-2), an important mediator of inflammation (Stafford et al. 2010). A separate study using the KD in combination with radiation therapy in the same mouse model demonstrated reduced expression of both COX-2 and Nf-κB while reducing the production of ROS (Woolf et al. 2013). Similar results have demonstrated reduced expression of pro-inflammatory markers, cyclooxygenase-2 (COX-2), nuclear factor kappa B (NF-κB), and macrophage inflammatory protein (MIP-2) using caloric restriction in mouse models of astrocytoma (Mulrooney et al. 2011) and colon cancer (Harvey et al. 2013).
9 KD as an Adjuvant Therapy
Although evidence suggests that the KD provides antitumor benefits on its own, perhaps the most effective use of the KD is in combination with standard cancer therapies such as radiation and chemotherapy. The KD greatly enhanced survival in a mouse model of malignant glioma when combined with TMZ when compared to either treatment alone (Scheck et al. 2011). In addition, a separate study showed that 9 out of 11 animals maintained on the KD and treated with radiation had complete and sustained remission of their implanted tumors, even after being switched back to a standard rodent diet (Fig. 3) (Abdelwahab et al. 2012). Allen et al. reported similar results when the KD is combined with radiation and chemotherapy in a lung cancer xenograft model (Allen et al. 2013). That is, they found decreased tumor growth rate and increased survival. CR and short-term fasting have also been found to be synergistic with radiation and other anticancer therapeutics in both preclinical and clinical studies (Champ et al. 2013, 2014; Klement and Champ 2014; Lee et al. 2010, 2012; Poff et al. 2013; Raffaghello et al. 2008, 2010; Safdie et al. 2012; Saleh et al. 2013; Seyfried et al. 2012).
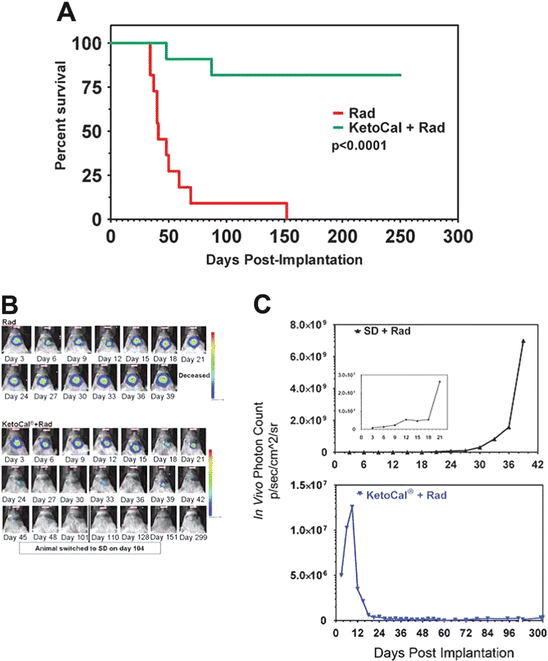
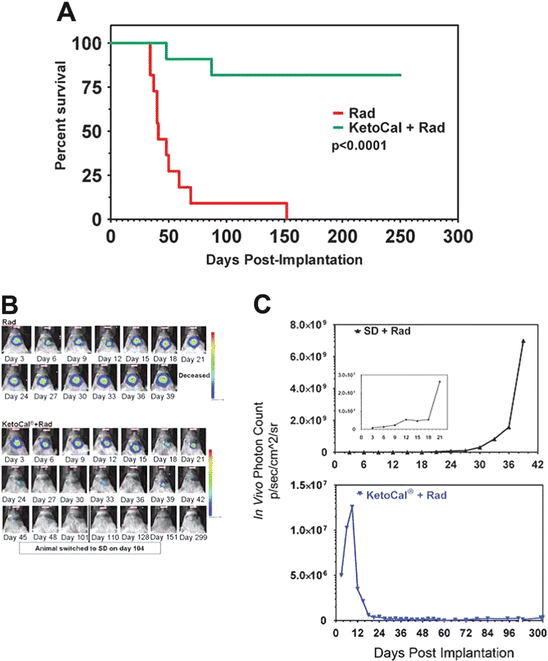
Fig. 3
Radiation in combination with the ketogenic diet causes tumor regression. On days 3 and 5 postimplantation animals received 4 Gy of radiation. The tumor completely regressed in 9 of the 11 animals fed a ketogenic diet. Animals were switched back to standard diet on day 101 and maintained for an additional 200 days and no tumor regrowth was detected. (a) Kaplan–Meier survival plot; (b) bioluminescence in representative animals treated with radiation and fed a standard diet vs. radiation plus the ketogenic diet; (c) bioluminescent signal plotted as in vivo photon count versus days postimplantation. Reprinted from (Abdelwahab et al. 2012)
The effectiveness of radiation therapy is due to a number of factors including relative damage done to tumor cells versus normal tissue and the ability of normal cells and tumor cells to repair the damage (Klement and Champ 2014). Radiation works, in part, by creating ROS through the radiolysis of water. The ROS damage the DNA and other macromolecules, causing sublethal damage that can become lethal if not repaired. The potentiation of radiation therapy by the KD or caloric restriction seems paradoxical in light of our data demonstrating a reduction in ROS in tumors from animals maintained on a KD (Stafford et al. 2010). However, radiation effects do not only occur through ROS, and radiation can directly damage DNA and other cellular macromolecules. Furthermore, in addition to reactive oxygen species, radiation causes the production of reactive nitrogen species (RNS), a potential source of macromolecular damage following radiation (Saenko et al. 2013). Whether the KD and/or caloric restriction reduces the formation of RNS is as yet unknown. In fact, the main effect of the KD or CR may not be in altering the amount of radiation-induced damage, but may in fact be in modulating the ability of tumor and normal cells to repair radiation-induced damage (Klement and Champ 2014; Santivasi and Xia 2014). Studies have shown that caloric restriction can enhance DNA repair in normal cells (Heydari et al. 2007); however, this may not be the case in tumor cells, and the differential response of tumor cells and normal cells to genotoxic stress may be mediated by reduced IGF1 and glucose in the tumor cells. In fact, a number of studies have shown that reduction of activation of the PI3K/Akt pathway, activation of the adenosine monophosphate-activated protein kinase (AMPK) signaling pathway, and reduction of receptor tyrosine kinase growth factor pathways can all reduce radioresistance in tumor cells (Choi et al. 2014; Danhier et al. 2013; Gil Del Alcazar et al. 2014; Li et al. 2014; Medova et al. 2013; Munshi and Ramesh 2013; Sanli et al. 2014; Wang et al. 2013; Zhang et al. 2014). These reports provide additional support for the use of the KD or caloric restriction as an adjuvant therapy for the treatment of gliomas.
10 Neuroprotection
There is a resurgence of interest in the use of the KD for the treatment of medically refractory epilepsy and increasing interest in the use of this therapy for the treatment of malignant brain tumors. While the majority of the research in this field focuses on slowing tumor growth and enhancing the efficacy of current therapeutic modalities, the KD may have additional benefits for cancer patients. Evidence suggests that the ketogenic diet may also protect normal brain tissue from the genotoxic stress that is a typical “side effect” of radiation and chemotherapy. We have demonstrated that gene expression changes in the tumor tissue from animals fed the KD were not the same as those in the non-tumor containing contralateral side of the brain (Scheck et al. 2012; Stafford et al. 2010). This allows for the hypothesis that while the neuroprotective activity of the KD does not protect the tumor from the therapeutic benefits of radiation and chemotherapy, it may reduce the deleterious side effects of cranial radiation on normal brain. A recent publication showed that fasting, which elevates blood ketones, not only sensitizes many types of cancer cells to standard therapies but may promote the protection of normal tissue from the toxicity associated with radiation and chemotherapy (Lee et al. 2012). Additional studies are needed to support this hypothesis; however, the potential benefit of protecting the normal brain from decreased cognitive function due to radiation toxicity would be of great importance, particularly for the treatment of pediatric brain tumors.
11 KD in Other Cancers
Although much of the research regarding the anticancer benefits of the KD has focused on brain tumors, this type of metabolic therapy has also recently been explored in other cancer types. For example, Gluschnaider et al. used the MMTV-PyMT oncomouse model to demonstrate that a KD suppressed breast tumor growth (Gluschnaider et al. 2014). Likewise, Allen et al. showed that the KD enhanced radiation and chemotherapy responses in a mouse lung xenograft model by increasing oxidative stress in both NCI-H292 and A549 lung xenograft models (Allen et al. 2013). The use of a no carbohydrate ketogenic diet (NCKD) in prostate cancer models has also been examined. A recent study demonstrated that an NCKD significantly slowed tumor growth and prolonged survival in a prostate cancer xenograft model (Freedland et al. 2008). Studies in prostate cancer xenograft models demonstrated that the NCKD significantly reduces tumor volume (Kim et al. 2012) and alters pathways linked to apoptosis, inflammation, and insulin resistance (Mavropoulos et al. 2009). A KD supplemented with omega-3 fatty acids and medium chain triglycerides was shown to delay tumor growth in a mouse xenograft model of gastric cancer. The use of the KD in models of cancer-associated cachexia has also been studied. Shukla et al. showed that a KD reduced glycolytic flux and glutamine uptake in a number of pancreatic cell lines. They identified decreased pancreatic cancer cell growth as well as a dose-dependent induction of apoptosis in the presence of ketone bodies in vitro. Likewise, in the presence of ketones, pancreatic cancer cells had a reduced expression and activity of the oncogene c-Myc and reduced cachexic markers. They also showed a reduction of tumor growth and cachexia in an animal model of pancreatic cancer (Shukla et al. 2014).
Although the mechanisms behind the KD have not been completely identified and extend beyond the reduction in blood glucose and increase in blood ketones, recent studies in cancer research suggest that the KD may provide therapeutic benefits in a variety of cancer types. This demonstrates that more research is warranted to better understand the mechanisms behind the KD as well as the different physiological responses which occur based upon cancer type and location.
12 KD in Humans
Studies of glucose utilization in cancer go back prior to the 1980s, including studies of metabolism and cancer cachexia (Fearon et al. 1988; Tisdale et al. 1987). These and other studies suggested that the ketogenic diet consisting of a high percentage of medium chain triglycerides (MCT) along with various supplements resulted in weight gain and improved nitrogen balance in both animals and humans. In 1995, Nebeling and colleagues published a case report in which they used a similar ketogenic diet based on MCT oil to treat 2 female pediatric patients with advanced stage malignant brain tumors (Nebeling et al. 1995; Nebeling and Lerner 1995). They demonstrated that dietary-induced ketosis decreased the availability of glucose to the tumor without causing a decrease in patient weight for overall nutritional status. Furthermore, both children had long-term tumor management (Nebeling et al. 1995). The 2nd case report was published in 2010 by Zuccoli and coworkers (2010). This patient was a 65-year-old female with multicentric glioblastoma. She was put on a 4:1 (ratio of fats:carbohydrate plus protein) calorie restricted (600 kcal/day) ketogenic diet during radiation and chemotherapy. During this time her body weight dropped by 20 %, she had reduced blood glucose, increased urinary ketones, and, most importantly, no observable brain tumor by either fluorodeoxyglucose Positron Emission Tomography (FDG-PET) or magnetic resonance imaging (MRI). The tumor recurred 10 weeks after the patient resumed her normal eating habits and she succumbed to her disease less than 2 years after diagnosis. While this patient did not experience long-term tumor control after cessation of the diet, this report demonstrated that the diet could be tolerated, even when used in a calorie-restricted setting. Results of a phase 1 clinical trial were reported in 2011 by a German group. Tolerability of a restricted calorie ketogenic diet was tested in 16 patients with a variety of advanced (end-stage) cancers. There were no severe side effects and 5 of the 16 patients were able to complete the 3-month treatment. These 5 patients had stable disease while on the diet. Two of the 11 remaining patients died early following the beginning of the trial, one was unable to tolerate the diet and dropped out immediately, 2 patients dropped out for personal reasons, one was unable to continue the diet for more than a month, and 3 had disease progression within less than 2 months of starting the diet and one dropped out to resume chemotherapy. While this trail demonstrated tolerability and favorable side-effect profile, the antitumor efficacy could not be assessed due to the variety and severity of disease in the patients. More recently, a number of prospective clinical trials have been initiated. A study in Germany is evaluating the efficacy of a calorie-restricted ketogenic diet and transient fasting during re-irradiation for patients with recurrent GBM (ClinicalTrials.gov NCT01754350). Michigan State University is directing a similar trial evaluating a calorie-restricted KD for recurrent GBM (ClinicalTrials.gov NCT01535911). A third clinical trial is evaluating the KD as adjunctive treatment in refractory/end-stage GBM (ClinicalTrials.gov NCT01865162). The goals for all of these studies are to obtain data on the safety, efficacy, and tolerability of the KD as an adjunctive therapy for patients with GBM. The only study using the KD as an up-front, concurrent therapy has recently been approved and is now open for enrollment at St. Joseph’s Hospital and Medical Center and Barrow Neurological Institute in Phoenix, Arizona (ClinicalTrials.gov NCT02046187). This trial for patients with primary GBM will evaluate the classic 4:1 ketogenic diet therapy during radiation treatment and concurrent temozolomide followed by the modified Atkins Diet (1:1 fat:carbohydrate plus protein) during temozolomide treatment.
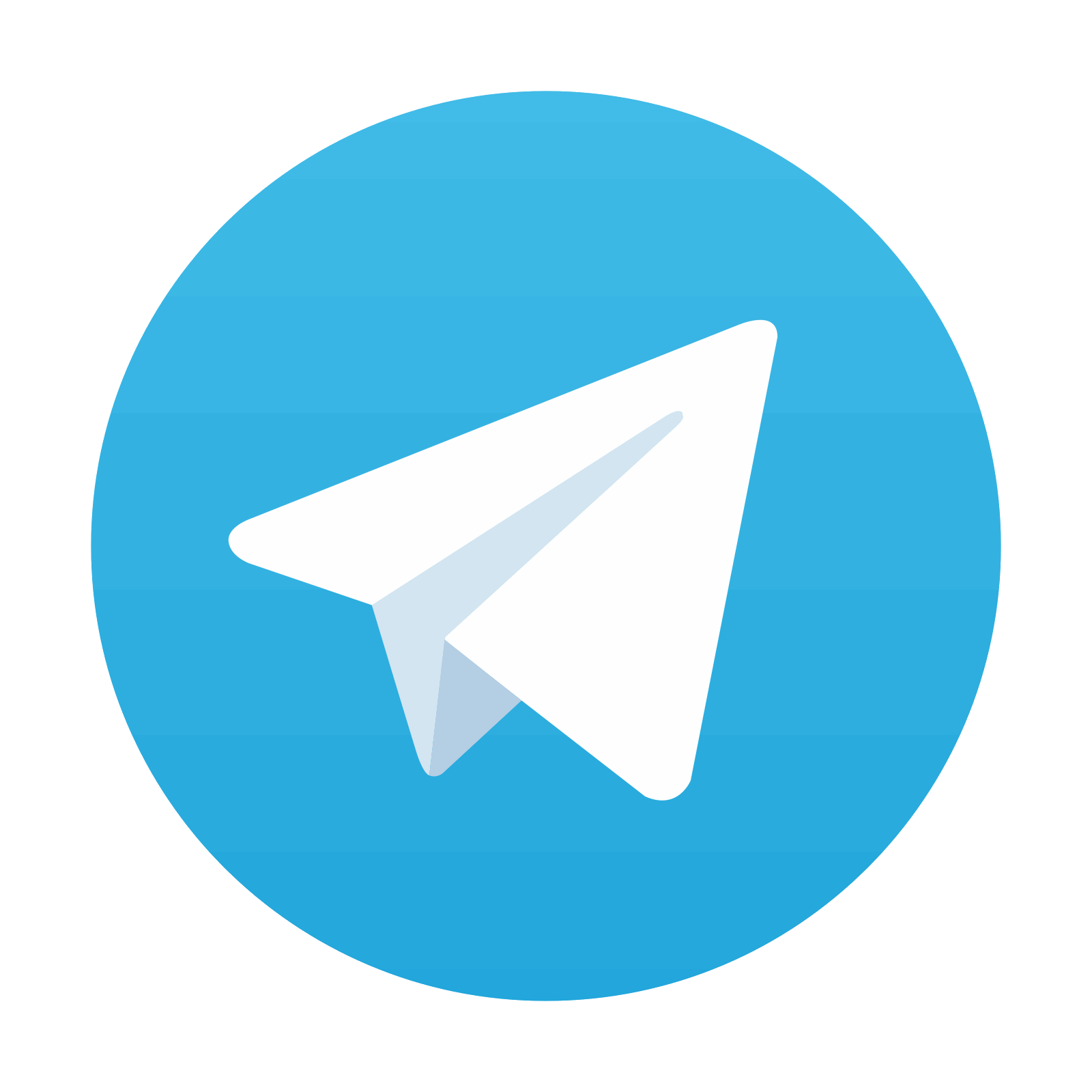
Stay updated, free articles. Join our Telegram channel

Full access? Get Clinical Tree
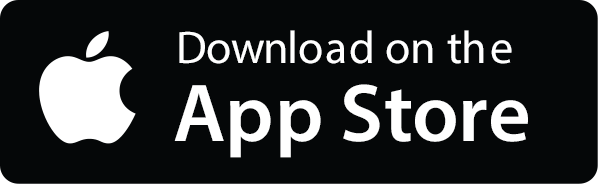
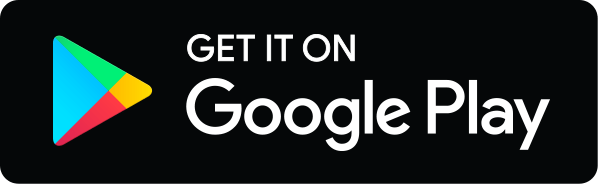