Anemia is common among people living in low- and middle-income countries, and alleviation of the global burden of anemia is an essential global health target over the next decade. Estimates have attributed about half the cases of anemia worldwide to iron deficiency; a range of other causes probably make a similar overall contribution. Individuals living in low-income settings experience a simultaneous high burden of infection with inflammation and iron deficiency. At least in children, iron supplementation exacerbates the risk of infection in both malaria-endemic and nonendemic low-income countries, whereas iron deficiency is protective against clinical and severe malaria.
Key points
- •
Anemia remains a highly prevalent global health problem, affecting 43% of children younger than 5 years, 38% of pregnant women, and 29% of nonpregnant women worldwide.
- •
Current public health strategies to alleviate this burden include iron supplementation, home fortification with multiple micronutrient powders, and/or universal fortification of staple foods.
- •
Evidence that iron interventions benefit health outcomes is strongest in pregnant and nonpregnant women and weakest in young children.
- •
Emerging evidence indicates that iron interventions, especially in children, increase the risk of infection (especially from malaria and diarrhea) in areas of high transmission.
- •
Stratification of iron interventions toward children most in need, for example, using novel biomarkers, such as hepcidin, may facilitate more rational, safer, and effective interventions in the population for the future.
Epidemiology of anemia
There have been several recent estimates of the prevalence of anemia across the world. Overall, these studies indicate that the prevalence of anemia has marginally reduced. Although, with growth in the global population, the absolute number of anemic individuals continues to increase. Stevens and colleagues studied the trends in hemoglobin (Hb) concentrations for each country between 1995 and 2011 using an ongoing systematic review of 257 national surveys, peer-reviewed scientific reports of national and subnational surveys held on the World Health Organization (WHO) Vitamins and Mineral Nutrition Information System, and data from national and international agencies. Data were specifically considered for children younger than 5 years and pregnant and nonpregnant women, adjusted for altitude and smoking, and were reported in the original resources. The investigators estimated that in 2011, the global mean Hb concentration and anemia prevalence in children was 111 g/L and 43% respectively, in pregnant women 114 g/L and 38% respectively, and in nonpregnant women 126 g/L and 29% respectively.
These estimates reflect a probable improvement in Hb concentrations and anemia prevalence since 1995. However, enormous disparity persists, reflecting differences in economic development and infection burden. For example, the prevalence of anemia in Central and West African children exceeds 70%, and the prevalence also exceeds 50% in East Africa and in South Asia; the prevalence is only 11% in high-income countries. Similar patterns are seen among pregnant and nonpregnant women. Overall, Stevens and coworkers estimated that about 273 million children, 496 million nonpregnant women, and 32 million pregnant women were anemic in 2011. The investigators also estimated that, although most anemia was amenable to iron in settings where few other causes of anemia exist (eg, Latin America, high-income countries), this decreases to less than half in settings where malaria infection is endemic. In mid-2015, the WHO republished global estimates for the prevalence of anemia, confirming approximately 800 million women and children were anemic worldwide in 2011 ( Figs. 1–3 ).



A second estimate of the global prevalence of anemia, which subsequently estimated the disease burden of anemia, was published in 2014 by Kassebaum and colleagues. Using data from more than 400 references, the investigators modeled the global prevalence of anemia in 187 countries and across both sexes and 20 age groups and then modeled cause-specific attribution to anemia due to 17 key diseases. The investigators then estimated the total years lived with disability (YLDs) using global burden of disease methodology. The investigators reported that 32.6% of the world’s population were anemic in 2010 and that about 50% of cases of anemia overall were attributable to iron deficiency. In contrast to the Stevens analysis, the prevalence of anemia attributable to iron deficiency was smaller (not higher) in high-income countries where other causes (eg, hemoglobinopathies, renal impairment) were considered more important. Malaria was responsible for a quarter of cases of anemia in sub-Saharan Africa. The investigators identified a similar distribution of anemia among populations (ie, most prevalent in young children and pregnant women). The investigators estimated that anemia accounts for about 68 million YLDs, almost 9% of the total for all conditions, underlining the potential importance of anemia as a global health problem; even though the individual disability weights assigned to mild and moderate anemia are relatively small, the enormous prevalence of this condition makes it a global health priority. The results of this study should be considered with some reserve, however, as the investigators used thresholds for defining anemia in children younger than 5 years (Hb <120 g/L) inconsistent with clinical recommendations (Hb <110 g/L), likely overestimating the prevalence (and burden) of anemia in this group.
Epidemiology of anemia
There have been several recent estimates of the prevalence of anemia across the world. Overall, these studies indicate that the prevalence of anemia has marginally reduced. Although, with growth in the global population, the absolute number of anemic individuals continues to increase. Stevens and colleagues studied the trends in hemoglobin (Hb) concentrations for each country between 1995 and 2011 using an ongoing systematic review of 257 national surveys, peer-reviewed scientific reports of national and subnational surveys held on the World Health Organization (WHO) Vitamins and Mineral Nutrition Information System, and data from national and international agencies. Data were specifically considered for children younger than 5 years and pregnant and nonpregnant women, adjusted for altitude and smoking, and were reported in the original resources. The investigators estimated that in 2011, the global mean Hb concentration and anemia prevalence in children was 111 g/L and 43% respectively, in pregnant women 114 g/L and 38% respectively, and in nonpregnant women 126 g/L and 29% respectively.
These estimates reflect a probable improvement in Hb concentrations and anemia prevalence since 1995. However, enormous disparity persists, reflecting differences in economic development and infection burden. For example, the prevalence of anemia in Central and West African children exceeds 70%, and the prevalence also exceeds 50% in East Africa and in South Asia; the prevalence is only 11% in high-income countries. Similar patterns are seen among pregnant and nonpregnant women. Overall, Stevens and coworkers estimated that about 273 million children, 496 million nonpregnant women, and 32 million pregnant women were anemic in 2011. The investigators also estimated that, although most anemia was amenable to iron in settings where few other causes of anemia exist (eg, Latin America, high-income countries), this decreases to less than half in settings where malaria infection is endemic. In mid-2015, the WHO republished global estimates for the prevalence of anemia, confirming approximately 800 million women and children were anemic worldwide in 2011 ( Figs. 1–3 ).
A second estimate of the global prevalence of anemia, which subsequently estimated the disease burden of anemia, was published in 2014 by Kassebaum and colleagues. Using data from more than 400 references, the investigators modeled the global prevalence of anemia in 187 countries and across both sexes and 20 age groups and then modeled cause-specific attribution to anemia due to 17 key diseases. The investigators then estimated the total years lived with disability (YLDs) using global burden of disease methodology. The investigators reported that 32.6% of the world’s population were anemic in 2010 and that about 50% of cases of anemia overall were attributable to iron deficiency. In contrast to the Stevens analysis, the prevalence of anemia attributable to iron deficiency was smaller (not higher) in high-income countries where other causes (eg, hemoglobinopathies, renal impairment) were considered more important. Malaria was responsible for a quarter of cases of anemia in sub-Saharan Africa. The investigators identified a similar distribution of anemia among populations (ie, most prevalent in young children and pregnant women). The investigators estimated that anemia accounts for about 68 million YLDs, almost 9% of the total for all conditions, underlining the potential importance of anemia as a global health problem; even though the individual disability weights assigned to mild and moderate anemia are relatively small, the enormous prevalence of this condition makes it a global health priority. The results of this study should be considered with some reserve, however, as the investigators used thresholds for defining anemia in children younger than 5 years (Hb <120 g/L) inconsistent with clinical recommendations (Hb <110 g/L), likely overestimating the prevalence (and burden) of anemia in this group.
Framework for anemia control
Reduction of the global burden of anemia is generally considered within the scope of public health nutrition, and solutions have focused on micronutrient interventions incorporating iron. Public health approaches must be implementable on a large scale by health workers without specialized training and at low cost per individual recipient. Modern public health interventions must be able to be justified on risk-benefit and health economic analyses, whereby costs incorporate the price not just the unit costs of iron but also the broader implementation program. Several solutions are presently available for providing iron in the public health context. These solutions include iron supplementation, home fortification with multiple micronutrient powders, and central food fortification ( Table 1 ).
Children | Women |
---|---|
|
|
Multiple micronutrient powders | Multiple micronutrient tablets |
Central staple-food fortification | |
Improve dietary diversity | |
Deworming | |
Delayed cord clamping | Birth spacing |
Prevent infection (especially malaria) |
Iron supplements (medicinal iron) are an established and effective approach for giving iron to women (including pregnant women) and are cheap to manufacture and distribute. However, this intervention has been difficult to implement in children because of the limited ability of children to swallow tablets; liquid supplements or dispersible tablets are required. An alternative approach for delivery of iron in children is home-based fortification with multiple micronutrient powders (MMPs, often also abbreviated as MNPs). These powders comprise lipid-encapsulated iron together with other micronutrients (at least vitamin A and zinc and often also several other micronutrients). These doses are provided in individual sachets that are then applied on the child’s food after serving. The two approaches seem to have comparable adherence and effects on Hb. The key benefit for MMPs may be in their ease of delivery. However, despite escalating implementation in programs in many countries, there are surprisingly few data directly comparing iron supplements with MMPs or confirming the benefits of MMPs on functional health outcomes beyond improvements in nutritional biomarkers.
Fortification of food with iron centrally (eg, at point of manufacture) is mandated in many countries. Fortification of staple foods, including wheat, maize, and rice, can improve iron intake and has been shown to restitute iron stores. Long-term population studies indicate these interventions can reduce the burden of iron deficiency and anemia in the short to medium term, although some studies question their efficacy in the longer term. Fortification can occur centrally, for example, at the point where flour is milled and packaged or more peripherally at the level of local distribution points. Key limitations of fortification as a solution to iron deficiency anemia in developing countries include the possibility that iron may not reach groups at highest risk of undernutrition unless special efforts are made to target programs, whereas groups at lower risk of iron deficiency (eg, men) may consume the equivalent amounts of the fortified food. Monitoring and evaluation of these programs should, therefore, incorporate not just assessment of the impact of the interventions on iron deficiency but also on the risk of iron overload in susceptible groups (for example, populations with high burdens of hemoglobinopathies where iron absorption may be enhanced).
Other measures to address iron deficiency anemia
Delayed Cord Clamping
Following birth, placental transfer of blood to the newborn continues through the cord post partum. Delayed cord clamping (1–3 minutes after delivery rather than immediately after delivery) enables improved placental transfusion and has been shown to improve infant iron stores at 6 months of age, and recent evidence indicates that this strategy improves long-term child fine-motor and social (but not overall cognitive) development. The WHO recommends delayed cord clamping for all uncomplicated deliveries. However, delayed cord clamping is also associated with an increased risk of neonatal hyperbilirubinemia, a condition that can result in long-term neurologic sequelae.
Malaria Control
An adverse effect from malaria on iron absorption now seems well established. Studies measuring iron absorption during and following convalescence from acute infection with malaria in women and children have shown that, during infection, there are elevations in hepcidin associated with a reduction in iron absorption, with improvement in iron absorption once the infection is treated. For example, Cercamondi and colleagues showed that, in Beninese women with asymptomatic Plasmodium falciparum infection, treatment increased the mean dietary iron absorption from 10% to 18%, associated with a marked decrease in serum hepcidin. Doherty and colleagues showed that toddlers infected with malaria have much poorer iron incorporation into red cells (8%) than those without malaria (28%). Epidemiologically, marked increases in the prevalence of iron deficiency, iron deficiency anemia, and anemia in Gambian children from the beginning to end of the malaria season (iron deficiency: 18% to 31%; iron deficiency anemia 14% to 25%; anemia 56% to 69%) have been observed, suggesting a seasonal effect on iron deficiency potentially mediated by infection.
A second study has supported these findings from a different perspective: Frosch and colleagues studied the prevalence of iron deficiency in children younger than 5 years living in 2 Kenyan villages before and after a 12-month documented period of interruption of malaria transmission achieved by widespread indoor residual insecticide spraying and introduction of artemisinin combination therapy for malaria. The prevalence of iron deficiency decreased from 36% to 26% and anemia from 54% to 32%; regression analyses confirmed these changes were larger than would be expected for changes in age alone. Thus, malaria is likely to contribute to the burden of iron deficiency anemia in developing countries by elevating hepcidin and preventing iron absorption, and control of malaria may independently alleviate iron deficiency. Conclusive data on the effect of malaria control on the burden of iron deficiency and iron deficiency anemia are not yet available; however, malaria chemoprevention (for example, using intermittent preventative therapy) has similar effects on the prevalence of anemia as iron supplementation. Other infectious diseases producing a systemic inflammatory response (eg, diarrhea, pneumonia) may contribute to the burden of iron deficiency through a similar mechanism (raising hepcidin and preventing iron absorption); but this has not yet been measured.
Antihelminthic Therapy
Hookworm infection is an important cause of iron deficiency. Deworming is generally safe and cheap, reduces the burden of anemia, and should be included as part of anemia-control programs where hookworm is prevalent.
Iron Enrichment of Cooked Foods
Several approaches have been trialed to improve the iron content of cooked foods through leaching of iron from the vessel or an added component. Iron can enter the food when prepared in an acidic environment. For example, an Ethiopian study found that cooking food in iron pots improved iron content in the food and improved iron indices and Hb in children. More recently, efforts to fortify cooked foods using an iron fish added to food under preparation (together with acidification) have been attempted. Published reports from this approach indicate a short-term benefit on Hb and iron stores that does not persist beyond 3 months of use.
Benefits from Iron on Functional Health Outcomes
Data indicate that iron supplementation consistently improves Hb and iron stores and reduces the risk of anemia and iron deficiency in women and children. However, the functional benefits from iron on health outcomes have been less extensively reported in randomized controlled trials. In pregnancy, iron supplements increase birthweight and reduce the risk of low birth weight. Studies in Nepal and China have found that children of mothers randomized to iron interventions have reduced short- and long-term mortality. Two studies have reported on the effects of iron on long-term child development, with one trial identifying a beneficial effect from iron, whereas a second trial found no difference. The presence of an effect may depend on the underlying burden of iron deficiency in the population.
In nonpregnant women, meta-analyses indicate that iron supplementation improves both peak and submaximal exercise performance, particularly in women undergoing training and with iron deficiency. In women presenting with fatigue and ferritin levels less than 50 ug/L, oral iron treatment improves fatigue scores, although evidence of benefit on psychological symptoms and cognition in adults is ambiguous.
In children, although there are extensive observational data linking anemia and iron deficiency in young children (younger than 2 years) to impaired functional outcomes including poorer cognitive (mental and psychomotor) development, meta-analyses of available trials have not identified evidence of benefit from iron supplementation on this critical outcome ; 2 long-term follow-up studies (in Nepal and Thailand ) are now available, which similarly fail to show any effect from iron supplementation in young childhood on long-term cognitive outcomes. The overall quality of evidence for the effects of iron on cognitive development in preschool children is low, and further studies are urgently needed to identify whether a benefit exists, the magnitude of any effect, and whether baseline iron and anemia status predict a favorable response to iron. In older (school aged) children, iron supplementation improves child cognitive scores, including IQ in anemic children. It is important to note that, although impaired growth has been postulated as an effect from iron deficiency, interventional studies of iron supplements have identified evidence that iron supplements actually seem to reduce linear growth and weight gain, particularly in iron-replete children.
In malaria-endemic settings, a rationale for iron supplementation has been to reduce the risk of severe anemia, a life-threatening complication of infection, especially with malaria; however, current evidence indicates that severe anemia is not predominantly due to iron deficiency but rather inflammation and red cell destruction. Trials administering iron to children in malaria-endemic settings have never identified a reduction in hospitalization due to severe anemia, and reductions in severe anemia due to iron are 50% smaller than reductions in severe anemia achievable by malaria chemoprophylaxis; furthermore, coadministration of iron together with antimalarials does not reduce severe anemia beyond just giving antimalarials alone. Thus, there is presently inadequate evidence to establish the functional benefits of iron supplementation in young children, including in children in malaria-endemic settings.
Risks of Iron Supplementation
Infection
The potential risk of iron supplementation on infection has been long postulated, and human trials and experimental studies have consistently reported an increase in infection when organisms are provided excess iron. For example, historic trials in Somali Nomads identified a 5-fold increase in infection risk when treated with iron compared with a control, with infections resulting from a range of bacterial and parasitic microorganisms.
Microorganisms require iron, and the discovery of the hepcidin-ferroportin axis during the previous 15 years provides confirmatory evidence of the likely protective role, the iron-withholding response. Systemic iron homeostasis is orchestrated by hepcidin, a predominantly hepatic-derived hormone, which is expressed in response to iron (in order to suppress iron absorption and recycling by preventing iron egress into the serum from the duodenum and macrophage respectively). Importantly, hepcidin also comprises a component of and is elevated directly by the innate immune response (especially interleukin [IL]-6 and IL-22 ); it is, therefore, elevated in a range of infections, including malaria; bacterial infections, including typhoid and tuberculosis; and viral infections, including influenza A virus and human immunodeficiency virus. These findings demonstrates a clear physiologic drive to reduce serum iron (induce hypoferremia) during infection, which can be potentially overwhelmed by high doses of administered iron.
Data from more recent randomized controlled trials seem to indicate an increased risk from iron and iron-containing interventions on 3 key infections (malaria, diarrhea, and acute respiratory infection), which are highly prevalent among children in low-income countries. Mechanistic data support a direct interaction between iron interventions and malarial and bacterial infection.
Clinical trials data
In 2006, the Lancet published the results of the 2 largest randomized controlled trials of iron supplementation in young children ever in Pemba, Tanzania (a sub-Saharan African malaria-endemic setting) and Nepal (a South Asian non–malaria-endemic setting). These trials together randomized almost 34,000 children aged 1 to 35 months to iron and folate (12.5 mg unless younger than 12 months of age, in which case 6.25 mg), with or without zinc, versus about 16,600 whom were assigned to placebo and were powered to detect an effect (a hypothesized benefit) from iron on mortality and serious morbidity. The two trials were both stopped early by the Data Safety Monitoring Board (DSMB) for different reasons. The Nepal study was ceased because the DSMB identified no evidence of a difference in morbidity between the groups and determined the power to identify an effect on mortality would be inadequate. The study found no effect from iron on overall or cause-specific mortality and no significant effect from iron on the incidence of diarrhea or pneumonia.
Conversely, the Pemba study was stopped for a different reason: Here, the DSMB was concerned about a significant increase in serious events among children randomized to iron, necessitating early cessation of the trial. The investigators reported that children in the iron folate group experienced a 12% increased risk of serious adverse events (defined as mortality or hospitalization) and were 11% more likely to be admitted to hospital, with a trend toward increased mortality. Children receiving iron had a 16% increased risk of serious adverse events due to clinical malaria and a 22% increased risk of cerebral malaria. There was also a 28% increase in hospitalization and 61% increase in death from nonmalarial infections. A substudy suggested that the increased risk was observed in children who were randomized to iron but were baseline iron replete, whereas a protective effect from iron on infection was seen in children with baseline iron deficiency anemia. However, these definitions were based on zinc protoporphyrin (ZPP) measurements, which are inaccurate in sub-Saharan African settings and which can represent not just iron deficiency but also inflammation; thus, the underlying iron status predicting these responses is uncertain. Key concerns with the applicability and implications of this trial have been that malaria control measures were not implemented as part of the study and coverage of prevention measures was considered fairly poor (eg, insecticide-treated net coverage was less than 40% in the main trial and 50% in the substudy). However, this is likely to reflect the situation in many sub-Saharan African settings, where bed net coverage exceeds 50% in only 4 of 23 countries evaluated.
Three further subsequent clinical trials have been undertaken to try to further assess the risk of infection from iron interventions in low-income settings. Each of these was considerably smaller than the initial two studies. These interventions did not use iron supplementation per se but instead deployed home fortification using iron-containing MMPs. In Ghana, Zlotkin and colleagues cluster randomized almost 2000 children to iron-containing or noncontaining MMPs, with coadministration of insecticide-treated bed nets as well as malaria treatment when needed. The trial was powered for superiority of placebo to be able to detect a 15% increase in malaria rate with iron. The investigators found a lower unadjusted rate of malaria in children randomized to iron-containing MMPs, which was no longer observed once analyses were adjusted for baseline anemia and malarial incidence. However, the investigators observed a significant 23% increase in hospitalization rate among children receiving iron, although cause-specific morbidity was not different between groups.
Two nonblinded trials of MMPs containing iron were undertaken in Pakistan. Soofi and colleagues cluster randomized 2746 children to no intervention or MMPs containing iron with or without zinc for 12 months from 6 to 18 months of age. The investigators observed an increase in rate of bloody diarrhea and severe diarrhea (reported by parents) as well as the proportion of days with diarrhea. The investigators also observed an increase in respiratory infection (evidenced by reported rapid breathing or chest indrawing) in children receiving one of the MMPs. A second smaller trial in Pakistan, unblinded and providing iron-containing MMPs to young children, again reported an increase in diarrhea incidence among children randomized to intervention. Critically, lack of blinding of these studies makes data based on parental reporting of symptoms difficult to interpret.
Mechanistic evidence for increased risk from iron interventions on malaria
In Malawian preschool children, an observational cohort study found that those with baseline iron deficiency had a lower incidence of malaria parasitemia (hazard ratio [HR] 0.55) and clinical malaria (HR 0.49) over 12 months. A similar cohort study in Tanzania found that children younger than 3 years with iron deficiency had a 23% reduction in subsequent clinical malaria, 38% reduction in severe malaria, 60% reduction in all-cause mortality, and 66% reduction in malaria-specific mortality. These data indicate that iron deficiency seems to protect against malaria; they conflict with the more generally prevailing view that to optimize health, iron deficiency should be averted.
Effects of iron depletion on malaria parasite growth in vitro have been long observed. For example, in vitro studies using a range of iron chelators and human trials of the iron chelator desferrioxamine reduce plasmodial infection. Recent experimental studies have shown that Plasmodium falciparum infects iron-deficient erythroblasts less efficiently and that reticulocytes generated during erythropoietic recovery from iron deficiency anemia are at increased susceptibility to merozoite invasion. These data support the hypothesis that restitution of erythropoiesis during treatment of iron deficiency exacerbates the risk of malaria.
Other potential pathways have been hypothesized to mediate the effect of iron on malaria risk. For example, large doses of iron may exceed plasma iron-binding capacity, resulting in a spike in non–transferrin-bound iron (NTBI), which may increase reactive oxygen species and cell damage, potentially enhancing endothelial damage and promoting parasitic adhesion as well as perhaps supporting bacterial infection. However, the role of NTBI infection in exacerbating malaria infection has not been confirmed experimentally or in humans. Importantly, because of the facilitative role of iron deficiency on iron absorption, NTBI is increased when iron is administered to an iron-deficient, compared with replete, individual.
Together, these mechanisms suggest a diabolical paradox: iron administration may in fact be at greatest risk of promoting infection among iron-deficient (and especially iron-deficient anemic) individuals, the very same group whom might benefit most from receiving iron.
Experimental evidence for increased risk from iron interventions on diarrhea
There has been considerable recent interest on the effects of iron interventions on the intestinal microbiome. Two studies undertaken in sub-Saharan African children where sanitation was poor and potentially harmful bacteria already comprised a sizable proportion of the intestinal microflora have found evidence that food fortification with iron (both at low doses and higher doses using MMPs) causes a shift toward pathogenic bacteria. In Côte d’Ivoire, in 6- to 14-year old children, iron-fortified biscuits containing a relatively low concentration of poorly bioavailable iron increased enterobacteria, reduced lactobacilli, and increased intestinal inflammation as measured by fecal calprotectin. Subsequently, using 16s pyrosequencing, Jaeggi and colleagues characterized the intestinal microbiomes of Kenyan infants participating in 2 randomized controlled trials of low-dose iron (2.5 mg) in micronutrient powders versus control or high-dose iron in MMPs (12.5 mg) versus control. In both trials, although most of the intestinal bacteria at baseline were nonpathogenic, a high proportion of potentially pathogenic bacteria were also observed. Among children receiving iron, there was a significant increase in potentially pathogenic enterobacteria, including Escherichia and Shigella and Clostridium . In the high dose iron trial, there was a trend toward an increase in clinical diarrhea among children receiving iron. Importantly, iron-containing MMPs increased fecal calprotectin, a biomarker of intestinal inflammation. Notably, however, in a trial among older children in South Africa, where there was a lower prevalence of pathogenic bacteria at baseline, iron provision of high-dose iron in the form of 50-mg tablets did not increase the risk of diarrhea, increase intestinal inflammation, or alter enteric flora. Thus, the clinical observations of increased risk of diarrhea from iron may be attributable to increases in intestinal burden of pathogenic bacteria, which may be particularly frequent among children where carriage of such bacteria is already endemic.
Other risks of iron supplementation
In adults, iron supplementation with ferrous sulfate is associated with gastrointestinal side effects, including abdominal discomfort, nausea, and constipation (as well as stool discoloration). These side effects can be ameliorated by reducing the dose or frequency of doses or taking the iron with food rather than in between meals. The incidence of these adverse effects in young children is less well defined, as reporting of side effects is less feasible. However, a systematic review identified an increase in the risk of vomiting among children receiving iron.
In children, there is evidence that iron supplementation can reduce growth rate. The mechanism for this is unclear; potential explanations include increased risk of infection, reduced appetite due to gastrointestinal adverse effects, or impairment in dietary zinc (which is important for linear growth) absorption or utilization due to interference by iron. Indeed, meta-analysis has confirmed that coadministration of iron and zinc is less effective at improving zinc concentrations compared with zinc supplementation alone.
Ferrous salt preparations can be lethal in overdose, especially in children: the dose that is lethal to 50% of experimental animals for ferrous sulfate is 255 to 350 mg/kg. In the United States, 30.2% of deaths due to accidental overdose in children between 1983 and 1991 were caused by iron preparations. Iron supplementation programs (for example, to pregnant or nonpregnant women) could potentially distribute hundreds of thousands of courses of iron nationally, with potentially limited time for education at the level of interaction between health worker and consumer regarding safe storage and potential risks. Furthermore, packaging may not be secure; in many settings, supplements are not provided in individual blisters but are in bottles or even paper envelopes, from which they could be accessed and consumed by children. Data on risk of unintentional poisoning from iron in low-income settings are unavailable, but the risk is unlikely to be remote. Several alternative iron formulations are considered nontoxic in overdose, such as carbonyl iron and iron polymaltose complex.
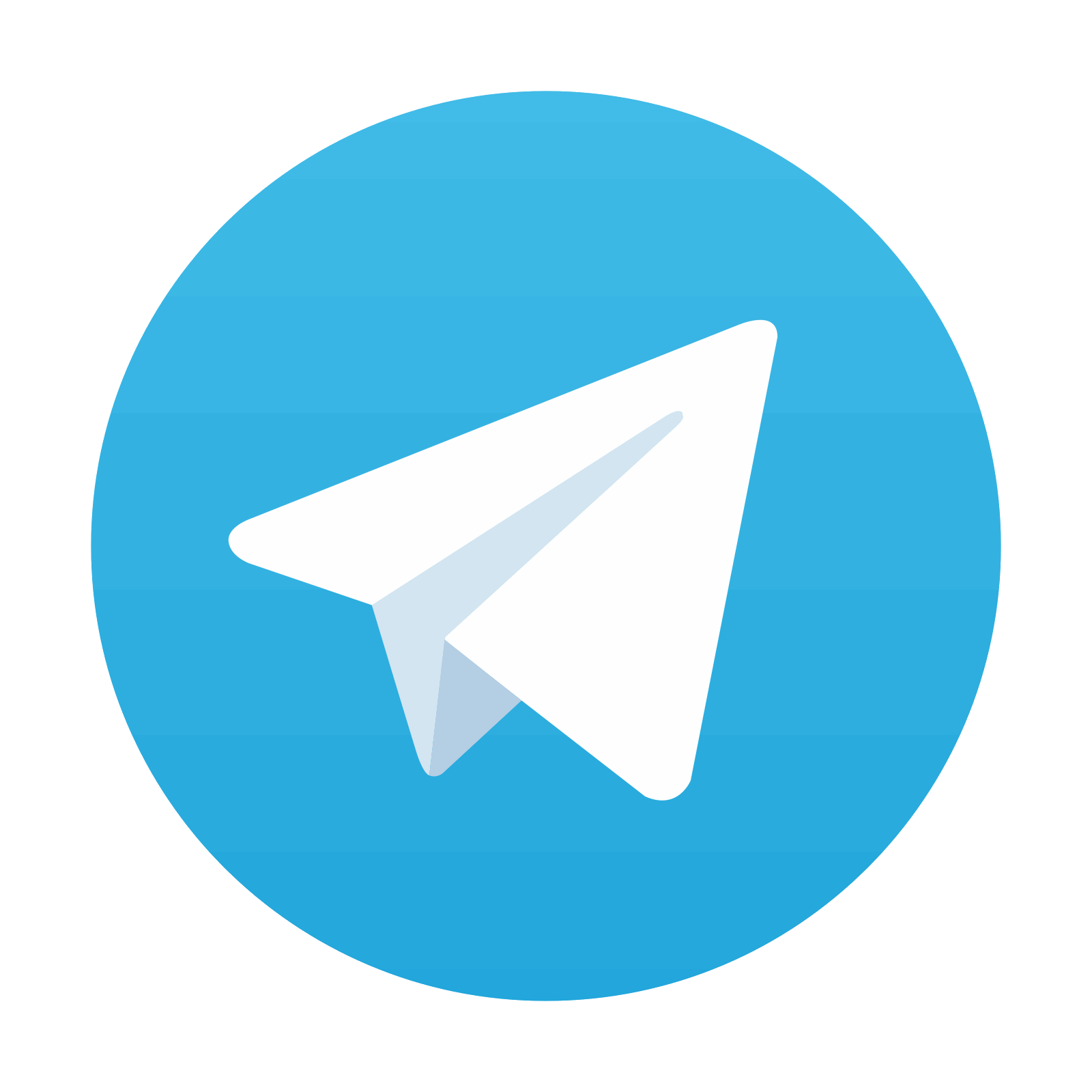
Stay updated, free articles. Join our Telegram channel

Full access? Get Clinical Tree
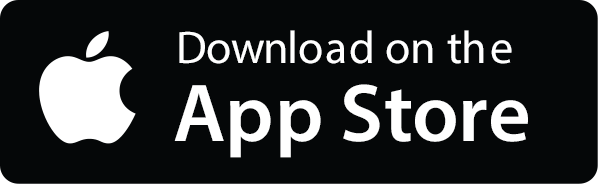
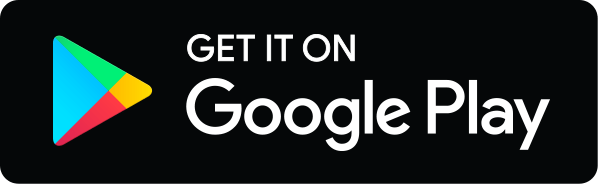