Fig. 13.1
The scheme shows the mechanisms which concur in the stimulation of maternal thyroid function during a normal pregnancy (Modified from Ref. [7])
13.1.3 Iodine Requirements
Although with some limitations, several studies have established the iodine requirement at different age and physiological conditions.
The WHO recommends 90 μg of iodine daily for infants and children up to 5 years, 120 μg for children 6–12 years, 150 μg daily for children ≥12 years and adults, and 250 μg daily during pregnancy and lactation [2] (Table 13.1). The US Institute of Medicine–recommended minimum daily intake of iodine is similar: 90 μg daily for children 1–8 years old, 120 μg for children 9–13 years, 150 μg daily for older adolescents and nonpregnant adults, 220 μg for pregnant women, and 290 μg for lactating women [8].
Age or population group | Iodine intake (μg/die) |
---|---|
Children 0–5 years | 90 |
Children 6–12 years | 120 |
Children ≥12 years/adults | 150 |
Pregnancy | 250 |
Lactation | 250 |
The iodine requirements are higher in pregnant women because of the above-listed changes in thyroid physiology.
13.1.4 Assessment of Iodine Intake
The methods for the assessment of iodine nutrition in the populations are goiter prevalence, urinary iodine concentration (UIC), serum TSH in newborns, and serum thyroglobulin (Tg). The urinary iodine concentration indicates current iodine nutrition, while thyroid size and the serum thyroglobulin concentration reflect iodine nutrition over a period of months or years.
Goiter
goiter can be measured by neck inspection and palpation or by thyroid ultrasonography. According to WHO/ICCIDD, grade 0 is a thyroid that is not palpable or visible, grade 1 is a goiter that is palpable but not visible when the neck is in the normal position, and grade 2 is a goiter that is clearly visible when the neck is in a normal position. Thyroid ultrasound is more sensitive and specific than palpation but requires valid references of thyroid volume data. Goiter surveys as indicators of iodine sufficiency are usually done in school-age children because they are easily recruitable and hopefully reflect the actual impact on humans of iodine deficiency, although enlarged thyroids in children who were iodine deficient during the first years of life may not regress completely after introduction of salt iodization [9]. The WHO has established total goiter rate in schoolchildren to define severity of iodine deficiency in populations: below 5.0 % indicates iodine sufficiency, 5.0–19.9 % mild deficiency, 20.0–29.9 % moderate deficiency, and above 30.0 % severe deficiency. In addition, a reduction of goiter rate by ultrasound indicates that iodine deficiency has disappeared, and therefore a frequency of goiter under 5 % in schoolchildren must be considered as a parameter of iodine sufficiency [10].
Urinary iodine concentration
because >90 % of dietary iodine eventually appears in the urine, the UIC is an excellent indicator of recent iodine intake. It is measured in spot urine specimens from a representative sample of a population and expressed as the median, in μg/L. The median urinary iodine concentration in a population has been used to develop a system for classifying iodine deficiency and sufficiency (Table 13.2) [2]. The most usual survey group is school-age children, but their nutrition must reflect that of the community in order for the data to be meaningful. Mild iodine deficiency is defined as a median urinary iodine concentration of 50–99 μg/L, moderate deficiency as 20–49 μg/L, and severe deficiency as <20 μg/L [11]. Pregnant women require special attention because their renal threshold for iodine is lower, the needs of the fetus are greater, and dietary salt (including iodized salt) is often restricted [12, 13]. In pregnant women, urinary iodine concentrations of 150–249 μg/L are considered adequate [14].
Table 13.2
Epidemiological criteria from WHO for assessment of iodine nutrition in school-aged children, pregnant and lactating women, and infants based on median or range of UI (Ref. [2])
UI (μg/L) | Iodine intake | Iodine nutrition |
---|---|---|
School-aged children | ||
<20 | Insufficient | Severe iodine deficiency |
20–49 | Insufficient | Moderate iodine deficiency |
50–99 | Insufficient | Mild iodine deficiency |
100–199 | Adequate | Optimum |
>200 | More than adequate | Risk of iodine induced hyperthyroidism |
>300 | Excessive | Risk of adverse health consequences |
Pregnant women | ||
<150 | Insufficient | |
150–249 | Adequate | |
250–499 | More than adequate | |
≥500 | Excessive | |
Lactating women | ||
<100 | Insufficient | |
≥100 | Adequate | |
Children less than 2 years | ||
<100 | Insufficient | |
≥100 | Adequate |
TSH
TSH concentration obtained during the neonatal screening of congenital hypothyroidism (CH) is useful to assess iodine nutrition, because the increase of fetal TSH is an adaptative mechanism to iodine deficiency. Compared with the adult, the newborn thyroid contains less iodine but has higher rates of iodine turnover. When iodine supply is low, maintaining high iodine turnover requires increased TSH stimulation. Therefore, iodine deficiency causes a shift toward higher TSH values in the neonatal screening of CH. A TSH value >5 mU/L in whole blood collected 3–4 days after birth that lasts for few weeks in more than 3 % of newborns indicates iodine deficiency in the population [2]. Studies suggest also that newborn TSH is a sensitive indicator of iodine nutrition during pregnancy along with determination of the median UIC [15].
Serum Tg
Tg is the most abundant intrathyroidal protein. Serum Tg is higher in iodine-deficient than in iodine-sufficient areas as a consequence of the TSH stimulation and the higher rate of goiter, and its concentrations fall quickly with the implementation of iodine prophylaxis. In iodine-deficient infants and children, serum Tg concentrations are high more often than are serum TSH concentrations. Although a nonspecific test, since any type of thyroid stimulation or injury can raise the serum Tg concentrations, the values correlate well with the severity of iodine deficiency. Tg has also been shown to be a sensitive measure of excess iodine intake in school-age children [16]. Moreover, in one study the serum Tg level was better than thyroid volume measurement by ultrasound as an indicator of iodine nutrition [17].
13.2 Consequences of Iodine Deficiency
The clinical consequences of inadequate iodine intake are collectively termed “the iodine deficiency disorders” (IDD) (Table 13.3) [18]. When severe iodine deficiency occurs during pregnancy, it is associated with cretinism and increased neonatal and infant mortality. Mild iodine deficiency is associated with thyroid enlargement and learning disabilities in children. All these consequences of iodine deficiency stem from the associated hypothyroidism.
Table 13.3
Iodine deficiency disorders (IDD) by age group
Age groups | Consequences of iodine deficiency |
---|---|
All ages | Goiter |
Fetus-neonate | Abortion |
Stillbirth | |
Congenital anomalies | |
Perinatal and infant mortality | |
Endemic cretinism | |
Overt or subclinical hypothyroidism | |
Infant-child/adolescent | Impaired mental function |
Delayed physical development | |
Adult | Toxic nodular goiter |
Iodine induced hyperthyroidism | |
Hypothyroidism | |
Endemic mental retardation | |
Decreased fertility rate |
Additional factors that can exacerbate the effects of iodine deficiency include coexistent deficiencies of iron, selenium, and vitamin A [19] and the ingestion of foods such as cassava or millet containing goitrogenic substances.
Goiter is the most frequent manifestation of iodine deficiency and can affect individuals of all ages. It represents a compensatory response to iodine deficiency. Low iodine intake leads to reduced T4 and T3 production, which results in increased TSH secretion in an attempt to restore iodothyronine production to normal. TSH also stimulates thyroid growth. The goiter is initially diffuse but eventually becomes nodular because the cells in some thyroid follicles proliferate more than others. Therefore, in regions of iodine deficiency, children and adolescents generally have diffuse goiters, while adults who lived in conditions of long-standing iodine deficiency have nodular goiter.
This chapter will focus on the consequences of iodine deficiency from fetal life to childhood.
13.2.1 Consequences of Iodine Deficiency During Pregnancy and Infancy
13.2.1.1 Neurological Development
Goiter is the most common clinical manifestation of iodine deficiency, but another important consequence is a defective development of central nervous system because brain development depends on thyroid hormones during fetal and early postnatal life.
THs have no influence on very early stages of neurological development but regulate its later processes, which include neurogenesis, myelination, dentrite proliferation, and synapse formation [20, 21]. In particular, three stages of thyroid hormone–dependent neurological development can be recognized. The first occurs before the onset of fetal thyroid hormone synthesis, which occurs at 16–20 weeks post conception in humans. During this period, TH exposure comes only from maternal hormones [1, 22] and influences proliferation and migration of neurones in the cerebral cortex, hippocampus, and medial ganglionic eminence [23, 24]. The second stage occurs during the remainder of pregnancy after the onset of fetal thyroid function when the developing brain derives its supply of TH from both the fetus and the mother [1, 22]. During this period, thyroid hormone regulates neurogenesis, neuron migration, axonal outgrowth, dendritic branching, and synaptogenesis, together with the initiation of glial cell differentiation and migration and the onset of myelination [25]. The third stage occurs in the neonatal and postnatal period when thyroid hormone supplies to the brain entirely derive from the child and are critical for continuing maturation. During this period, while continuing gliogenesis and myelination, THs regulate migration of granule cells in the hippocampus and cerebellum, pyramidal cells in the cortex, and Purkinje cells in the cerebellum [25].
The frequency and severity of the neurological impairment are proportional to the magnitude of iodine deficiency. In areas of severe chronic iodine deficiency, maternal and fetal hypothyroxinemia can occur from early gestation onward.
13.2.1.2 Endemic Cretinism
The clinical manifestations caused by chronic severe iodine deficiency are referred to as endemic cretinism. In its classical description, endemic cretinism includes a neurological and a myxedematous form [26].
The neurological cretinism presents with severe mental retardation with squint, deaf-mutism, motor spasticity, and goiter. The mental deficiency is characterized by a marked impairment of abstract thought, whereas autonomic and vegetative functions and memory are relatively well preserved, except in the most severe cases. The motor disorder is characterized by proximal rigidity of both lower and upper extremities and the trunk, whereas spastic involvement of the feet and hands is unusual, therefore most cretins can walk.
The myxedematous form has less severe mental retardation and more pronounced hypothyroid features, including severe growth retardation, incomplete maturation of the facial skeleton with naso-orbital configuration abnormalities and atrophy of mandibles, puffy features, dry and thickened skin, dry and rare hair, and delayed sexual maturation. In this form, goiter is usually absent, and the thyroid is usually atrophic.
In many instances, cretinism may present as a mixed form with features of both. Therefore, it can be often difficult to differentiate the two forms [27].
Studies suggest that selenium deficiency combined with severe iodine deficiency can more specifically induce forms of atrophic rather than goitrous hypothyroidism and therefore of myxedematous rather than neurological cretinism [28]. Selenium is normally present in high concentrations in the normal thyroid and is essential for the synthesis of selenoproteins such as glutathione peroxidase (GPX), which acts as antioxidant, and type I 5’-deiodinase, which metabolizes thyroid hormones. The mechanism would be the following: iodine deficiency causes thyroid hyperstimulation by TSH that leads to increased production of H2O2 within the thyroid follicular cells; selenium deficiency also results in accumulation of H2O2 due to GPX deficit. Excess of H2O2 can induce thyroid cell destruction and myxedematous cretinism. On the other hand, deficiency of the selenoenzyme 5′-deiodinase causes decreased catabolism of T4–T3 with increased availability of T4 for the fetal brain and prevention of neurological deficits; T4, in fact, crosses the brain–blood barrier more easily than T3.
Cases of overt myxedematous, neurological, or mixed endemic cretinism are reported in areas of severe iodine deficiency such as Africa and Asia.
The only way to prevent neurological cretinism is by administration of iodine to women early in gestation or even before they become pregnant. In a randomized trial and several population-based studies of women living in severely iodine-deficient regions, iodine supplementation to women prior to conception or during early pregnancy was associated with substantially better neurological and developmental outcomes in children [29–31].
13.2.1.3 Subclinical Neurological Defects
Severe iodine deficiency has been almost eradicated through extensive iodine prophylaxis programs worldwide. As a consequence, new cases of cretinism have disappeared. However, several regions of mild to moderate iodine deficiency still exist [32].
Several reports have described cases of neurological deficits or minor neuropsychological impairments also in children born to mothers exposed to mild to moderate iodine deficiency during pregnancy. These defects may be detected by appropriate neuropsychological tests [33]. In Tuscany, neuropsychological performance was tested in 107 children living in a village characterized by mild iodine deficiency (UIC = 64 μg/L) by a block design subtest of the Wechsler Intelligence Scale for Children and simple reaction times to visual stimuli. The results obtained in these children were compared with those obtained in children born and living in an iodine-sufficient area. The block design test was not different between the two groups of children, while reaction times were significantly delayed in children living in the iodine-deficient village. These data indicate that mild iodine deficiency may impair the rate of motor response to perceptive stimuli even in the absence of general cognitive defects. Mild to moderate iodine deficiency was also shown to be associated with minor neurological deficits in Sicily [34].
However, randomized trials of iodine supplementation to pregnant women with mild to moderate iodine deficiency have reported mixed results in terms of improvements of thyroid function parameters, which may be considered as surrogate markers of future infant development [35]. In some but not in all trials [36, 37], iodine supplementation resulted in smaller thyroid volumes and lower Tg concentrations in mothers and/or newborns compared with controls. Indeed, there was no effect on maternal or neonatal T4 concentrations in the majority of the trials. Moreover, no final conclusion can be drawn from these studies because child cognitive outcomes were not measured.
An increased auditory threshold may be another clinical manifestation of iodine deficiency. As an example, in a study of 150 school-age children in Spain, 38 % had a goiter [38]. In this subset, there was an inverse relationship between auditory threshold and urinary iodine excretion (i.e., the more iodine deficient, the higher the auditory threshold).
The potential adverse effects of mild to moderate iodine deficiency during pregnancy on cognitive and/or neurological function of the offsprings are still uncertain.
Two prospective case–control studies have reported even mild thyroid dysfunction during pregnancy may impair cognitive development of the offspring [39, 40]. Children exposed to maternal hypothyroxinemia presented reduced IQ scores, subtle deficits in cognition, memory, visuospatial ability [39], and delayed mental and motor function [40]. Animal models confirmed that maternal hypothyroxinemia induced in a critical period of active neurogenesis resulted in alteration in cell migration and cytoarchitecture of the cortex and hyppocampus in the 40-day-old progeny [23, 41]. The limitation of these previous studies is that they were conducted in iodine-sufficient areas.
In two following observational studies of women with mild to moderate iodine deficiency and mild hypothyroxinemia, neurodevelopmental outcomes were better in children whose mothers received iodine supplementation (200–300 μg potassium iodide daily) early in pregnancy (prior to the 10th week of gestation) compared with children whose mothers did not [42, 43]. The better outcomes noted in these studies may be related to improvement in maternal hypothyroxinemia. Both mild and severe maternal hypothyroxinemia have reportedly been associated with a higher risk of expressive language delay in newborns [44]. Severe maternal hypothyroxinemia also predicted a higher risk of nonverbal cognitive delay. It is possible that iodine supplementation in women with iodine deficiency severe enough to cause maternal hypothyroxinemia may improve neurodevelopmental outcomes, but this has not been assessed in randomized trials.
13.2.1.4 Birth Weight and Infant Growth
There is evidence that correction of iodine deficiency during pregnancy in severely iodine-deficient areas determines improvements of head circumference and birth weight of offspring.
In an area of western China, iodine repletion of pregnant women reduced the prevalence of microcephaly from 27 to 11 % [29]. In studies conducted in Algeria and Zaire, treatment of women with oral iodized oil just before conception or early in pregnancy resulted in respectively 6.25 % and 3.7 % higher birth weight compared with offspring of untreated mothers [45, 46]. (For the relationship between iodine deficiency and somatic growth, see Sect. 13.2.2.2.)
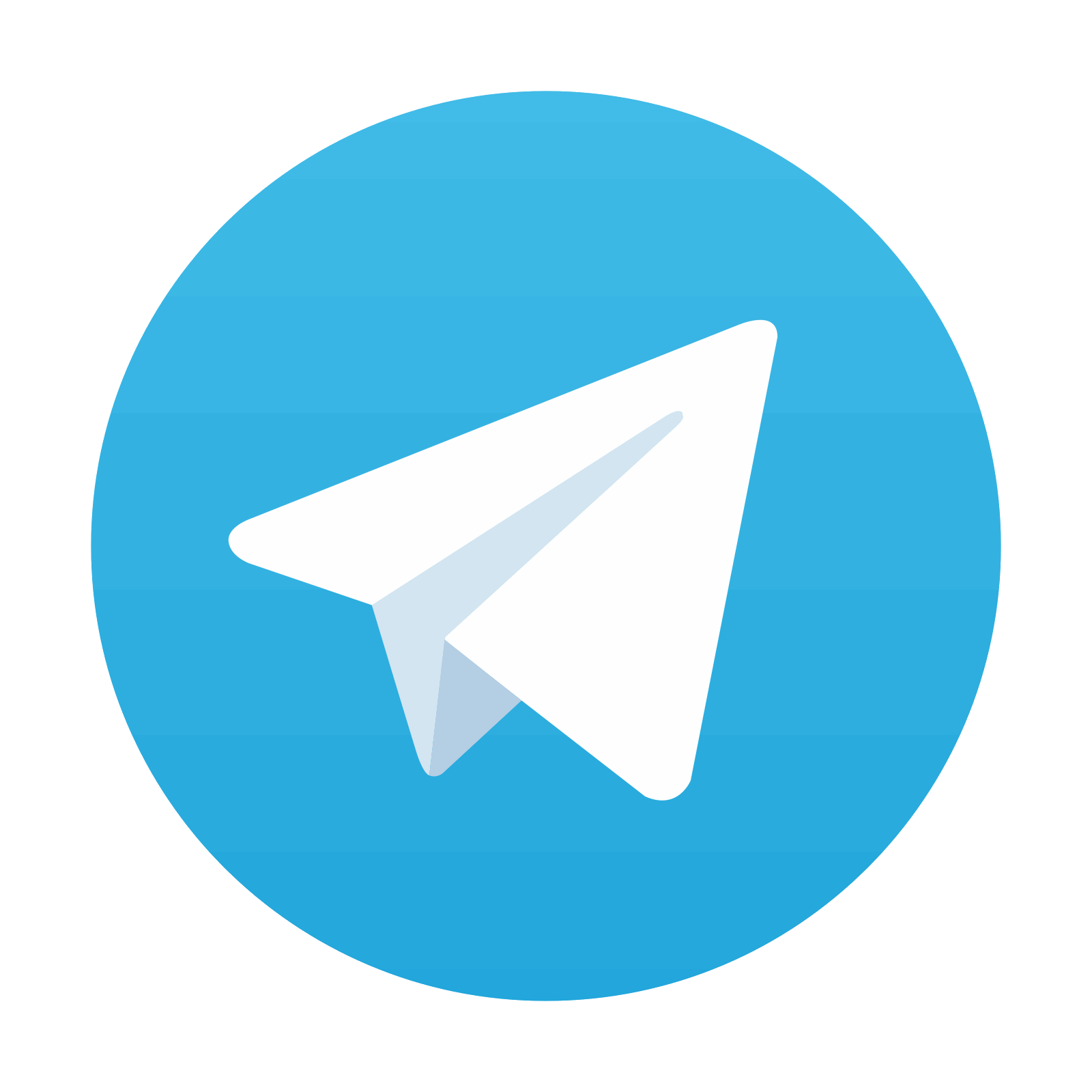
Stay updated, free articles. Join our Telegram channel

Full access? Get Clinical Tree
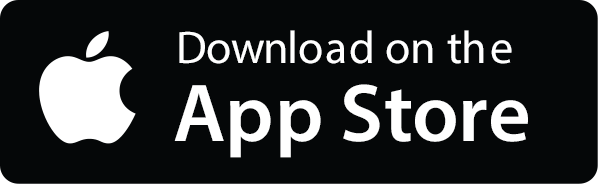
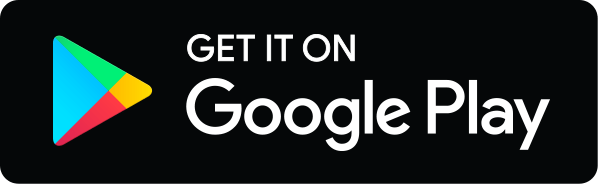