Introduction: Process, Equipment, and Personnel
Faiz M. Khan
Every patient with cancer must have access to the best possible care regardless of constraints such as geographic separation from adequate facilities and professional competence, economic restrictions, cultural barriers, or methods of health care delivery. Suboptimal care is likely to result in an unfavorable outcome for the patient, at greater expense for the patient and for society.
— BLUE BOOK
Radiotherapy procedure in itself does not guarantee any favorable outcome. It is through meticulous planning and careful implementation of the needed treatment that the potential benefits of radiotherapy can be realized. The ideas presented in this book pertain to the clinical, physical, and technical procedures in radiotherapy treatment planning. Optimal planning and attention to details will make it possible to fulfill the goal of the Blue Book (1), namely, to provide the best possible care for every patient with cancer.
Treatment Planning Process
Treatment planning is a process that involves the determination of treatment parameters considered optimal in the management of a patient’s disease. In radiotherapy, these parameters include target volume, dose-limiting structures, treatment volume, dose prescription, dose fractionation, dose distribution, positioning of the patient, treatment machine settings, and adjuvant therapies. The final part of this activity is a blueprint for the treatment, to be followed meticulously and precisely over several weeks.
Target Volume Assessment
Treatment planning starts right after the therapy decision is made and radiotherapy is chosen as the treatment modality. The first step is to determine the tumor location and its extent. The target volume, as it is called, consists of a volume that includes the tumor (demonstrated through imaging or other means) and its occult spread to the surrounding tissues or lymphatics. The determination of this volume and its precise location is of paramount importance. Considering that radiotherapy is basically an agent for local or regional tumor control, it is logical to believe that errors in target volume assessment or its localization will cause radiotherapy failures.
Modern imaging modalities such as computed tomography (CT), magnetic resonance imaging (MRI), ultrasound, single photon emission computed tomography (SPECT), and positron emission tomography (PET) assist the radiation oncologist in the localization of target volume. However, what is discernible in an image may not be the entire extent of the tumor. Sufficient margins must be added to the demonstrable tumor to allow for the uncertainty in the imaging as well as the microscopic spread, depending upon the invasive characteristics of the tumor.
Next in importance to localization of the target volume is the localization of critical structures. Again, modern imaging is greatly helpful in providing detailed anatomic information. Although such information is available from standard anatomy atlases, its extrapolation to a given patient is fraught with errors that are unacceptable in precision radiotherapy.
Assessment of the target volume for radiotherapy is not as easy as it may sound. The first and foremost difficulty is the fact that no imaging modality at the present time is capable of revealing the entire extent of the tumor with its microscopic spread. The visible tumor, usually seen through imaging, represents only a part of the tumor, called the gross tumor volume (GTV). The volume that includes the entire tumor, namely, GTV and the invisible microscopic disease can be estimated only clinically and is therefore called the clinical target volume (CTV).
The estimate of CTV is usually made by giving a suitable margin around the GTV to include the occult disease. This process of assessing CTV is not precise because it is subjective and depends entirely on one’s clinical judgment. Because it is an educated guess at best, one should not be overly tight in assigning these margins around the GTV. The assigned margins must be wide enough to ensure that the CTV thus designed includes the entire tumor, including both the gross and the microscopic disease. If in doubt, it is better to be more generous than too tight because missing a part of the disease, however tiny, would certainly result in treatment failure.
Added to the inherent uncertainty of CTV are the uncertainties of target volume localization in space and time. An image-based GTV, or the inferred CTV, does not
have static boundaries or shape. Its extent and location can change as a function of time because of variations in patient setup, physiologic motion of internal organs, patient breathing, and positioning instability. A planning target volume (PTV) is therefore required, which should include the CTV plus suitable margins to account for the above uncertainties. PTV, therefore, is the ultimate target volume—the primary focus of the treatment planning and delivery. Adequate dose delivered to PTV at each treatment session, presumably, assures adequate treatment of the entire disease-bearing volume, the CTV.
have static boundaries or shape. Its extent and location can change as a function of time because of variations in patient setup, physiologic motion of internal organs, patient breathing, and positioning instability. A planning target volume (PTV) is therefore required, which should include the CTV plus suitable margins to account for the above uncertainties. PTV, therefore, is the ultimate target volume—the primary focus of the treatment planning and delivery. Adequate dose delivered to PTV at each treatment session, presumably, assures adequate treatment of the entire disease-bearing volume, the CTV.
Because of the importance of accurate determination of PTV and its localization, the International Commission on Radiation Units and Measurements (ICRU) has come up with a systematic approach to the whole process, as illustrated in Figures 1.1 and 1.2. The reader is referred to ICRU Reports 50, 62, and 71 for the underlying concepts and details of the system (2,3,4).
Although sophisticated treatment techniques such as intensity-modulated radiation therapy (IMRT) and image-guided radiation therapy (IGRT) are now available, which account for organ motion and positional uncertainties as a function of time, the basic problem still remains: How accurate is the CTV? Unless the CTV can be relied upon with a high degree of certainty, various protocols to design PTV from it and the technical advances to localize it precisely in space and time would seem rather arbitrary, illusory, or even make-believe. Therefore, the need for technological sophistication (with its added cost and complexity) must be balanced with the inherent uncertainty of CTV for a given disease.
The above seemingly pessimistic view of the process, however, should not discourage the development or the use of these technologies. It should rather be taken as a cautionary note for those who may pursue such technologies with a blind eye to their limitations. Technological advances must ultimately be evaluated in the context of biological advances. “Smart bombs” are not smart if they miss the target or, worse yet, produce unacceptable collateral damage.
Treating the right target volume conformally with the right dose distribution and fractionation is the primary goal of radiotherapy. It does not matter if this objective is achieved with open beams or uniform-intensity wedged beams, compensators, IMRT, or IGRT. As will be discussed in the following chapters, various technologies and methodologies are currently available, which should be selected on the basis of their ability to achieve the above radiotherapy goal for the given disease to be treated. In some cases, simple arrangements such as a single beam, parallel-opposed beams, or multiple beams, with or without wedges, are adequate, while in others IMRT or IGRT is the treatment of choice.
Treatment Volume
Armed with knowledge from the clinical evaluation, biological characteristics of the disease, imaging studies, and laboratory tests, the radiation oncologist is in a position to design the PTV, as discussed earlier. The treatment volume must be larger than the PTV to allow for limitation of the treatment technique. The treatment volume is defined by an isodose surface that adequately covers the PTV, and its value represents the minimum required target dose. The margin between the treatment volume and the PTV is dictated by the particular treatment technique and equipment. Strictly speaking, the treatment volume cannot be optimally designed without an accurate knowledge of PTV and isodose distribution, both in three dimensions.
Traditionally, the first attempt at designing the treatment volume is made in the simulator room. By this time, all the diagnostic information about the disease must be available. Although diagnostic CT or MRI is usually obtained with the patient positioning differing from that to be used for radiotherapy treatment, the information provided by these images can still be used to design a rough outline of the treatment volume. Treatment fields, possible beam directions, patient positioning, patient immobilization, and surface contours are initially planned using simulator radiographs to visualize the treatment volume. Since a detailed dose distribution in the volume to be irradiated is not available at this stage, the setting up of accurate margins between the target volume and the treatment volume is not possible. However, approximate field boundaries can be marked on the simulator film to include, with suitable margins, the target volume. Modification or fine-tuning of the plan should be possible after dose distribution has been obtained.
A more modern simulation procedure involves the acquisition of CT images every few millimeters in the region of interest, with the patient in the treatment position. Immobilization devices and fiducial markers are used to make patient positioning reproducible, as well as to allow registration of positioning landmarks. These closely spaced CT slices provide all the volumetric data one needs to perform a virtual simulation. Computer software allows processing of CT data to create images in any plane. Radiographs simulating any field orientation with appropriate beam divergence can be reconstructed. These digitally reconstructed radiographs (DRRs) form the basis of CT simulation. If PTV and critical structures (organs at risk) are outlined on each slice, the DRRs can incorporate these structures in radiographs reconstructed in any plane. Treatment planning tools allow treatment fields to be shaped and
directed for an optimally determined direction to provide appropriate coverage of the PTV and to minimize the dose to critical structures.
directed for an optimally determined direction to provide appropriate coverage of the PTV and to minimize the dose to critical structures.
In defining field boundaries, one takes into account the physical penumbra of the beam (e.g., lateral distance between the field edge and the 90% or 95% isodose curve). Therefore, the initial outline of the field includes the PTV plus estimated margins to allow for beam penumbra in order to provide adequate dosimetric coverage of the PTV. The final confirmation of the fields drawn must await the isodose plan.
Isodose Distribution
The objective of isodose planning is to optimize the irradiation technique and provide the best possible dose distribution. Ideally, one would like to deliver the necessary dose to the tumor-bearing areas and spare all the normal tissues. However, because of particular beam characteristics and limitations of the radiotherapy equipment, a certain amount of normal tissue irradiation is unavoidable. Moreover, the greatest problem continues to be the determination of CTV, as discussed earlier. Because of the uncertainty of microscopic tumor spread, targets have to be drawn more generously, at the risk of irradiating additional normal tissue.
Optimization of beam placement, beam modifiers, beam weights, and beam energy is traditionally done by iterative techniques. Numerous treatment techniques and field arrangements for different tumors and body sites have been developed over the years. Most treatment planners start with the well-established techniques and make modifications as needed to optimize dose distribution for a given patient. Since the process is essentially iterative and interactive, it is important to have computer software that is reasonably fast and user-friendly. Of course, as the system becomes more sophisticated, such as for three-dimensional (3D) planning, it can become slow and unfriendly.
Treatment planning for modern techniques such as IMRT and IGRT is best accomplished using inverse planning. These sophisticated algorithms allow planners to specify the desired dose distribution from the very start and let the computer program the machine settings, and the dynamic multileaf collimators (MLCs) achieve a treatment plan as close to the desired distribution as possible. These techniques will be discussed in the following chapters.
Treatment Fields
Next to target volume delineation, the most important task of treatment planning (non-IMRT) is treatment field delineation. Treatment fields initially set by simulation (conventional or CT scan) may require adjustments in shape, size, and orientation, depending on the isodose plan and other practical considerations. The final delineation of fields must be made after evaluating the isodose plan. For example, if a simulated field is not large enough to provide adequate isodose coverage of the target volume in three dimensions, it has to be suitably enlarged. The isodose plan can be used as a guide to make the final adjustments in field dimensions, shape, and other relevant treatment parameters. If these adjustments are major, such as beam orientation or beam location, it may be necessary to resimulate the patient. If it is simply a question of field margins around the target, these may be adjusted without resimulation or a repeat isodose plan. Nevertheless, any changes made in the isodose plan or the simulation films must be carefully and explicitly documented. One advantage of CT simulation is that once the volumetric data set is available, field modifications and other changes in the treatment plan can be made without resimulation. The DRRs can be obtained to document the final field arrangement.
Field Shaping
In designing fields, it is important to ensure that the appropriate margins are provided among the field, the target, and the critical structures, three-dimensionally. Although this can be ascertained more conveniently and completely by 3D planning systems, the adequacy of a treatment field can be evaluated slice by slice by measurement of field margins around the target, on which the target and isodose distribution have been displayed. As discussed earlier, field margins are established by examining 3D isodose plans or individually on representative CT scans.
Custom blocking with cerrobend (non-IMRT) has freed the radiotherapist from having to use rectangular or square fields irrespective of target shape. Fields can be designed in any shape by drawing on to a beam’s-eye-view (field outlined on a plane perpendicular to beam’s central axis) simulator film or DRR. With the use of hot-wire Styrofoam cutters, custom blocks can be designed to provide the desired field.
Availability of computer-controlled MLC has almost completely replaced the cutting of custom cerrobend blocks. These and other related developments, such as conformational radiation therapy, will be discussed later in this book.
Intensity Modulation
Traditional radiotherapy uses beams of a uniform-intensity profile. The uniformity criteria for individual beams are specified by flatness and symmetry. In some cases, the intensity profiles are intentionally modified, as in wedges, so that by combining beams of modified profiles, a conformal and uniform composite dose distribution is obtained. Compensators are another class of beam modifiers that are designed to make the beam profile uniform when it has been skewed or modified by the patient’s surface contour
or tissue heterogeneity. Wedges and compensators can be called intensity modulators. Their use for what we call IMRT is possible but not practical.
or tissue heterogeneity. Wedges and compensators can be called intensity modulators. Their use for what we call IMRT is possible but not practical.
Intensity modulation for modern techniques of IMRT and IGRT (to be discussed later in the book) is best accomplished by computer-controlled MLCs as in sliding window or step and shoot techniques of IMRT or special collimators using dynamic apertures as in tomotherapy machines. The required intensity modulation of beams in IMRT, IGRT, or tomotherapy is obtained through the use of inverse planning algorithms, which are designed to produce dose distributions with preset criteria of dose uniformity and conformity for the PTV and tolerance dose for the organs at risk.
Brachytherapy
The treatment planning process for brachytherapy is very similar to that for the external beam except that the treatment indications and treatment parameters are uniquely determined for each procedure. In most brachytherapy applications, the goal is to provide a boost dose to the clinically or radiographically demonstrable tumor or tumor bed (after surgical excision) using interstitial or intracavitary implantation of radioactive sources. Therefore, one of the most important parts of treatment planning, as in external beam therapy, is the target volume localization. This is accomplished by clinical examination, radiologic imaging, and/or CT scanning.
A number of systems have been established for low-dose-rate (LDR) brachytherapy implants: The Manchester, Quimby, Paris, Memorial, and Computer. These systems are characterized by their own rules of implantation and dose specification. Some of them require the use of elaborate patterns of source distribution and precalculated dosage tables. Current treatment planning techniques, however, use computerized treatment planning systems in which dosages are specified according to isodose distribution in relation to the implanted target volume. The evaluation of implants is aided by computer displays of dose distribution in three dimensions or in multiple planes of interest.
While LDR using manual after-loading or remote after-loading techniques is still popular, there is a definite trend toward high-dose-rate (HDR) brachytherapy because of its many advantages over LDR such as treatments on an outpatient basis, higher throughput for institutions with high patient loads, greater capability and flexibility of optimizing dose distribution, and greater personnel protection against radiation exposure. Although the clinical advantages or disadvantages of HDR versus LDR are not well established, HDR technology is fast gaining acceptance and may well replace LDR altogether in the not-too-distant future.
Several chapters in this book are dedicated to brachytherapy techniques and treatment planning. Brachytherapy is a well-established treatment modality in radiation therapy and, in some cases, is considered indispensable.
Facility Organization
Many radiotherapy facilities in the United States have only a single megavoltage unit and a large number of them are staffed by a single physician or with no full-time medical physicist. Such facilities are often not equipped or staffed to do adequate treatment planning. Realizing this nationwide problem, the authors of the Blue Book (1) wrote: “Treatment planning skills, a computer-based treatment planning system, simulation, direct medical radiation physicist involvement, high energy photon and electron beams, skilled brachytherapy and the capability to fabricate treatment aids must be available to the patients in small facilities, either on-site or through arrangements with nearby centers.”
Each patient undergoing radiotherapy requires careful treatment planning. Clinical evaluation, therapeutic plan, imaging, simulation, and isodose planning are all part of this process. The quality of the final treatment plan and of the treatment itself depends on how the facility is organized to carry out these tasks. As demonstrated by the Patterns of Care Study (5), the outcome of radiotherapy process depends critically on the facility structure, principally equipment and staffing.
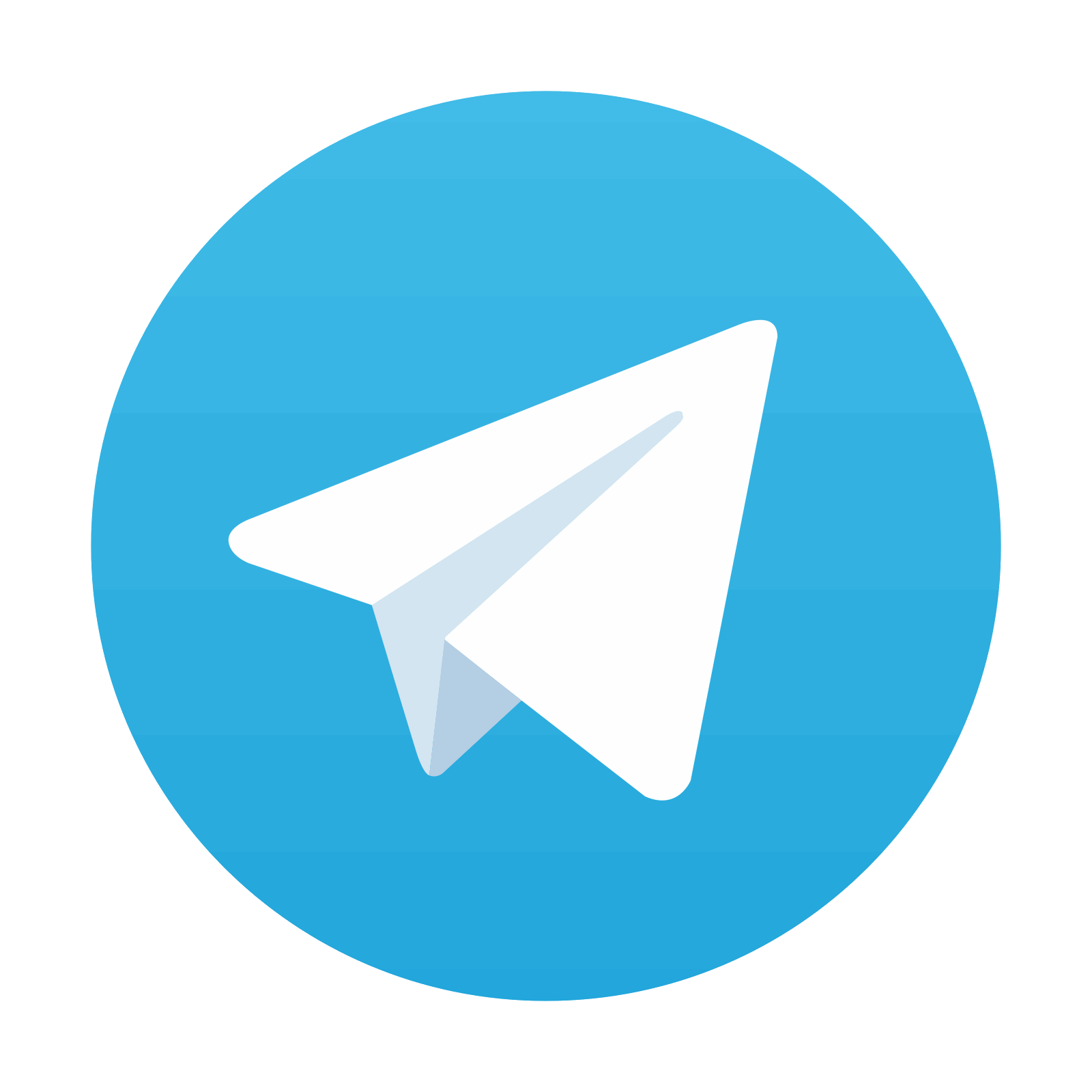
Stay updated, free articles. Join our Telegram channel

Full access? Get Clinical Tree
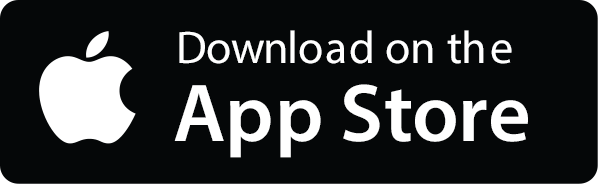
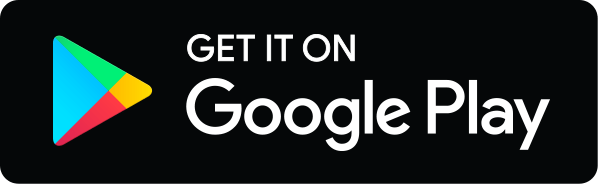