Fig. 15.1
Cholangiocarcinoma classification: a Intrahepatic cholangiocarcinoma: tumors in the bile ducts within the hepatic parenchyma b Extrahepatic cholangiocarcinoma: tumors from the junction of the left and right hepatic ducts to the common bile duct. c Hilar cholangiocarcinoma: at or near the junction of the left and right hepatic ducts. d Distal cholangiocarcinoma: distal to the confluence to just above the ampulla of Vater [Figure created using graphics from http://www.servier.com/Powerpoint-image-bank and PowerPoint. With permission from Creative Commons (https://creativecommons.org/licenses/by/3.0/)]
15.2 Epidemiology
The most recent assessment of cholangiocarcinoma in the USA according to SEER data reports that there were 11,296 patients (6036 men and 5260 women) with intrahepatic cholangiocarcinoma between the years 2000 and 2011. According to this data, the incidence of intrahepatic cholangiocarcinoma in the USA is 1.6 cases per 100,000 per year, with a prevalence of 15.88 per million persons. This incidence clearly increases with age and is highest in patients 80 years of age and older. When evaluated according to race/ethnic group, the incidence is higher in Asian and Hispanic populations as compared to non-Hispanic white and black populations [3].
Interestingly, the most recent estimates of intrahepatic cholangiocarcinoma incidence have significantly increased as compared to past SEER data, which demonstrated an incidence of 0.32 cases per 100,000 between 1975 and 1979, and 0.85 per 100,000 between 1995 and 1999 [4]. Given improvements in the diagnosis of cholangiocarcinoma in the USA since the 1970s, it is possible that some of this increase is related to increased detection of intrahepatic cholangiocarcinoma rather than a true increase in incidence. However, data evaluating this possibility has shown no trend toward a diagnosis of smaller tumors or earlier stage disease, and no increase in the degree of microscopic confirmation of disease, as may be expected in the case of improved diagnosis [4]. Furthermore, an increase related to increased detection of disease would be expected to plateau over time [3], which has not been seen according to most recent SEER data.
The true incidence of hilar cholangiocarcinoma is more difficult to assess, as it has been categorized as both intrahepatic and extrahepatic cholangiocarcinoma according to varied International Classification of Diseases for Oncology (ICD-O) coding schemes [5]. In addition, since hilar cholangiocarcinoma should be categorized as extrahepatic based on its anatomic involvement of the hepatic duct bifurcation, the incidence and prevalence data for intrahepatic cholangiocarcinoma may be falsely elevated by the inclusion of this subset, explaining some of the increase in its incidence described above. One study evaluating SEER registry data from 1973 to 2002 estimated that the misclassification of hilar cholangiocarcinomas led to overreporting of intrahepatic cholangiocarcinoma by 13% and underreporting of extrahepatic cholangiocarcinoma by 15%. However, even after the exclusion of hilar cholangiocarcinoma from the intrahepatic subset, the incidence of intrahepatic cholangiocarcinoma in the USA still increased between the years 1992 and 2000 [5].
A similar evaluation was undertaken in England and Wales, yielding comparable results. In addition, the latter study noted that there is no ICD code available for the term “hilar cholangiocarcinoma,” only for Klatskin tumors, leading to further confusion as the two terms are generally not used interchangeably for tumor registration purposes. Furthermore, the study identified that tumors that are not specifically classified beyond the term “cholangiocarcinoma” are most often categorized as intrahepatic cholangiocarcinoma by tumor registries [6]. According to this data, it is clearly very difficult to identify the true incidence of hilar cholangiocarcinoma.
Worldwide, cholangiocarcinoma as a whole represents about 10–25% of primary liver cancers, with incidence rates estimated between 0.3 and 1.5 per 100,000 in most Western countries as well as many Eastern Asian nations. However, cholangiocarcinoma represents the most common cause of cancer in men in Thailand, and the third most common cause in women, with incidence rates of 33.4 per 100,000 and 12.3 per 100,000, respectively, between 1998 and 2000 [7]. Though the same caveats of classification apply, the trend of increasing incidence of intrahepatic cholangiocarcinoma in the USA has also been seen in various European and Asian countries [8–10].
15.3 Risk Factors
A variety of risk factors have been found to contribute to the development of cholangiocarcinoma. Of these, liver fluke infection and biliary tract disorders associated with chronic inflammation are the best established.
Liver fluke infections have been most closely linked to cholangiocarcinoma risk in endemic areas of Asia, specifically Thailand, which explains at least a portion of the significant disease burden in this country. The liver fluke Opisthorchis viverrini is most closely associated with cholangiocarcinoma risk, though Clonorchis sinensis has also been implicated. Infection in humans occurs through ingestion of raw or undercooked fish serving as hosts. After ingestion, the parasites move from the duodenum to the biliary tree where they mature and can survive for over 10 years. The link between liver fluke infection and cholangiocarcinoma is thought to be chronic inflammation and associated tissue and DNA damage induced in the setting of this chronic infection, though additional host and environmental factors likely contribute [11]. Efforts to reduce the burden of liver fluke infections in endemic areas such as northeast Thailand are ongoing, with a significant decrease in such infections documented in an approximately 20-year period of attempted parasite control through education and treatment with the anti-helminthic agent praziquantel. An associated decrease in cholangiocarcinoma incidence has yet to be observed in these areas, though work to strengthen prevention programs is ongoing [12].
Another well-defined risk factor for cholangiocarcinoma is primary sclerosing cholangitis (PSC), which is associated with an estimated 7–9% incidence of cholangiocarcinoma. Various studies evaluating the risk of cholangiocarcinoma in PSC have determined that approximately half of cholangiocarcinomas diagnosed in this patient population are found within the first year of PSC diagnosis [13]. Chronic inflammation is thought to be the primary pathogenic feature leading to increased risk of cholangiocarcinoma in PSC, and as of yet, no additional factors have been identified as reliable predictors of cholangiocarcinoma in this population. There are no clear guidelines for screening for cholangiocarcinoma in PSC, though a combination of imaging, ERCP, and Ca 19-9 monitoring is generally accepted [14].
Bile duct cysts have also been identified as an underlying risk factor for the development of cholangiocarcinoma, with an incidence of 10–30% documented in adult patients. Highest risk has been associated with type I and type IV cysts, with an ongoing risk of developing cholangiocarcinoma remaining even after cyst excision. Higher concentrations and stasis of bile acids due to cysts, as well as reflux of pancreatic enzymes and amylase in the bile ducts, are thought to contribute to malignant transformation in these patients [15]. Given the association of malignancy with bile duct cysts, surgical resection is generally recommended for these lesions [16].
Through similar processes of biliary obstruction and bile stasis leading to chronic inflammation, hepatolithiasis has also been identified as a risk factor for cholangiocarcinoma. Though rare in Western countries, the prevalence of hepatolithiasis has been documented at up to 47% in parts of Asia [17], with cholangiocarcinoma identified in up to 10% of patients with intrahepatic stones [18].
In addition to these well-documented risk factors for cholangiocarcinoma, recent studies have suggested that cirrhosis and viral hepatitis B and C may also be associated with an increased risk of developing intrahepatic cholangiocarcinoma. Three meta-analyses have evaluated the risk of hepatitis B and cholangiocarcinoma, all focusing on intrahepatic disease. All three demonstrated an increased risk of intrahepatic cholangiocarcinoma, with documented relative risk of 3.42 (95% CI, 2.46–43.74) [19] and odds ratio of 5.45 (95% CI, 3.19–9.63) [20] and 3.17 (95% CI, 1.88–5.34) [21], respectively. One study also evaluated overall risk of cholangiocarcinoma in patients with hepatitis B infection and found a relative risk of 2.66 (95% CI, 1.97–3.60) [19]. A greater association with Asian versus Western populations was not consistently demonstrated across these three meta-analyses.
An increased risk of cholangiocarcinoma in patients with hepatitis C has also been documented in a recent meta-analysis, with an odds ratio of 5.44 (95% CI, 2.72–10.89). In a pooled risk assessment of intrahepatic and extrahepatic diseases, the risk was greater in the intrahepatic subset (OR-3.38, 95% CI, 2.72–4.21) as compared to extrahepatic (OR = 1.75, 95% CI, 1.00–3.05). In addition, this risk seemed to be more pronounced in North America than Asia (pooled OR = 6.48 vs. 2.01) [22].
Other potential risk factors for the development of cholangiocarcinoma include diabetes, obesity, and alcohol use, though these associations have not been as widely studied and associations are less strong [23]. In addition, there are some hereditary cancer syndromes with which cholangiocarcinoma is thought to be associated, including hereditary non-polyposis colorectal cancer (HNPCC) syndrome [24] and BAP1 hereditary cancer predisposition syndrome [25].
15.4 Diagnosis
Due to its anatomic location, cholangiocarcinoma can be difficult to clearly appreciate by imaging and access by biopsy, making diagnosis challenging, especially in the early stages of the disease. In addition, presenting symptoms are often non-specific, including such complaints as fatigue, weight loss, and generalized abdominal pain, without features isolating to the biliary tree. Painless jaundice can be associated with hilar cholangiocarcinoma, though is uncommon in patients with primary intrahepatic disease [26]. Multiple modalities are often required to make the diagnosis of cholangiocarcinoma, as will be detailed below.
15.4.1 Laboratory Testing
Though the CA 19-9 tumor biomarker is often used to trend disease activity in patients with known cholangiocarcinoma, its use as a diagnostic tool is limited. This is largely due to elevations of CA 19-9 in the setting of biliary obstruction and inflammation from any cause, as well as the fact that CA 19-9 may also be elevated in a variety of other malignancies, including primary pancreatic cancer. The sensitivity and specificity of CA 19-9 for the diagnosis of cholangiocarcinoma range widely between studies, though in one assessment of 322 patients with biliary tract disease, the sensitivity and specificity of CA 19-9 were 77.6% and 83% in patients without cholangitis or cholestasis, while in patients with either of these conditions, sensitivity and specificity decreased to 74% and 41.5%, respectively [27]. Also of note, approximately 10% of individuals are not capable of producing CA 19-9 due to lack of Lewis antigen [28].
Various studies have attempted to combine serum biomarkers in hopes of improving test specificity. One such trial identified the combination of an elevated CA 19-9 and elevated alkaline phosphatase level as most specific out of a panel of serum makers, with diagnostic accuracy reaching 95% in their study set of approximately 170 patients [29]. Additional potential biomarkers to aid in cholangiocarcinoma are under investigation, though none are yet validated for clinical use [26].
15.4.2 Imaging
Various imaging studies are often utilized in the workup of cholangiocarcinoma, including multiphasic contrast-enhanced computed tomography (CT) and magnetic resonance imaging (MRI). Unlike hepatocellular carcinoma, such studies are not generally considered to be diagnostic of cholangiocarcinoma, though they can help to guide further testing. Though positron-emission tomography (PET) may be helpful for the assessment of the nodal and metastatic involvement of disease in some scenarios [30], it is rarely useful in the diagnosis of cholangiocarcinoma, especially in early-stage disease. Magnetic resonance cholangiopancreatography (MRCP) can be helpful in the diagnosis of hilar lesions, with sensitivity and specificity similar to those of more invasive methods such as endoscopic retrograde cholangiography (ERCP) [31].
The limitations of using imaging to diagnose cholangiocarcinoma are largely related to difficulties in distinguishing bile duct cancers from other intrahepatic and biliary processes, both malignant and benign. Small intrahepatic cholangiocarcinoma lesions can be difficult to differentiate from hepatocellular carcinoma in the setting of underlying cirrhosis. A study of gadolinium-based contrast-enhanced MRI was performed in 71 patients with intrahepatic cholangiocarcinoma and 612 patients with hepatocellular carcinoma, all with underlying cirrhosis. When pathologic specimens were compared to imaging findings, stable and progressive contrast enhancement was demonstrated in significantly more intrahepatic cholangiocarcinomas, while classic contrast washout in delayed phases was demonstrated in a greater proportion of hepatocellular carcinomas. However, given some overlap in imaging findings between the two malignancies, these imaging characteristics are not considered diagnostic of intrahepatic cholangiocarcinoma [32].
The utility of the Liver Imaging Reporting and Data System (LI-RADS) v2014—developed to assist in the classification of hepatic lesions in patients at risk of hepatocellular carcinoma—has been assessed for utility in the diagnosis of intrahepatic mass-forming cholangiocarcinomas by gadoxetic acid-enhanced MRI. In a retrospective review of 35 pathologically confirmed intrahepatic cholangiocarcinomas in patients with cirrhosis or chronic hepatitis B, approximately 80% were correctly assigned according to the LI-RADS algorithm, though up to 11% were incorrectly diagnosed as HCC [33]. This again suggests that current imaging protocols are not sufficient for the diagnosis of cholangiocarcinoma.
Hilar cholangiocarcinoma can be difficult to distinguish from the benign autoimmune condition known as immunoglobulin G4 (IgG4)-associated cholangitis by imaging. Specific characteristics have been identified to help differentiate these two processes, with cholangiocarcinoma more commonly associated with strictures longer than 12 mm, asymmetric areas of narrowing, indistinct margins, and venous hyperenhancement. In contrast, multifocal biliary strictures, significant thickening of the bile duct wall, smooth margins, visible lumen, late arterial hyperenhancement, and homogeneous delayed phase hyperenhancement are more commonly associated with IgG4-associated cholangitis [34]. However, additional testing including IgG4 levels [35], ratios to total IgG or IgG1 [34], and histologic evaluation are necessary to rule out IgG4-associated cholangitis in most cases.
15.4.3 Endoscopy
While of minimal utility in the diagnosis of intrahepatic cholangiocarcinoma, ERCP and endoscopic ultrasound (EUS) can be very useful in the diagnosis of hilar cholangiocarcinoma, especially in settings where a tumor mass or stricture is not clearly visible on standard imaging. In this setting, ERCP and EUS can be both diagnostic and therapeutic, providing an opportunity for visual assessment and biopsy as well as dilation and stent placement for strictures.
If tissue sampling by ERCP is performed, there is a significant chance that pathologic assessment of brushing and biopsy results will be inconclusive, with the sensitivity of such testing ranging from 20 to 60% [36]. New endoscopic technology has emerged that may help improve diagnostic accuracy. Probe-based confocal laser endomicroscopy (pCLE) is a technique that utilizes a specialized probe and fluorescein contrast to provide real-time visualization of the epithelial and subepithelial tissue of suspicious biliary strictures to assist in making the diagnosis of malignancy [37]. A prospective study of pCLE with ERCP applied to indeterminate biliary strictures in 112 patients demonstrated a sensitivity of 89% and specificity of 82%, as compared to tissue sampling alone with a sensitivity of 56% and specificity of 100% [38].
15.4.4 Percutaneous Biopsy
There are concerns that percutaneous biopsy of a mass suspicious for cholangiocarcinoma increases the risk of tumor seeding and ultimately tumor spread. This concern is based on retrospective data demonstrating a significant increase in peritoneal metastasis in patients with hilar cholangiocarcinoma who underwent diagnostic percutaneous FNA biopsy prior to liver transplant. Of note, only six of 191 patients had a diagnostic FNA biopsy pre-transplant, although five of these six patients (83%) later developed peritoneal metastasis. None of nine patients with FNA biopsies that were negative for adenocarcinoma developed peritoneal disease, and only 8% of the patients who did not undergo biopsy were found to have peritoneal spread [39]. A second retrospective analysis of 67 patients with extrahepatic cholangiocarcinoma treated with percutaneous transhepatic biliary drainage (PTBD) prior to resection demonstrated a 6% incidence of catheter tract metastasis [40].
Concerns of seeding based on these data are primarily relevant to patients with localized disease being considered for a curative treatment. Given these patients will ultimately undergo surgical resection or transplantation, biopsy for tissue diagnosis is often deferred in this setting in hopes of mitigating the potential risk of seeding. However, biopsy should still be pursued in those patients who will be treated upfront with chemotherapy or radiation.
15.4.5 Pathologic Assessment
Though pathologic assessment is required to make the diagnosis of cholangiocarcinoma (Fig. 15.2), as noted above, obtaining sufficient biopsy tissue for histologic diagnosis is often limited by tumor location and paucicellular character [41]. The sensitivity of cytologic diagnosis for cholangiocarcinoma has been improved upon with the integration of fluorescence in situ hybridization (FISH) testing of biliary duct brushings (Fig. 15.3) [42]. The combination of cytology and polysomy per FISH further increases the diagnostic sensitivity (58%) above and beyond either modality alone (cytology, 21%; FISH 47%) [43]. Testing for polysomy by FISH is now considered a standard diagnostic tool, especially in cases where cytology alone is inconclusive for diagnosis.
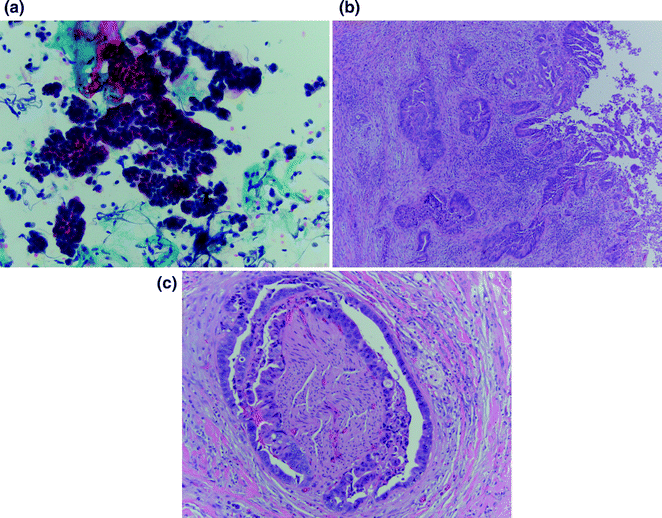
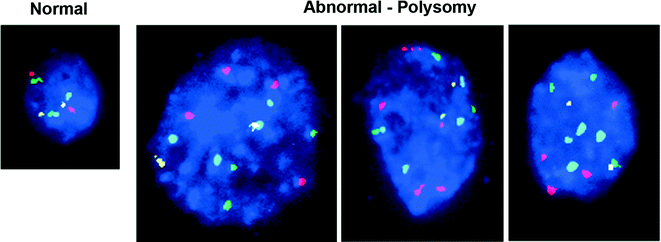
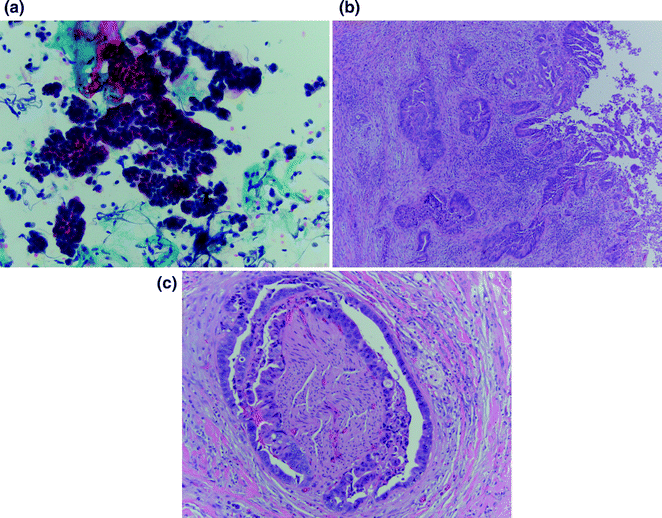
Fig. 15.2
Histologic diagnosis of cholangiocarcinoma: a Cytologic specimen consistent with adenocarcinoma by pap stain at 20×. b. H&E tissue specimen demonstrating high-grade dysplasia at the bile duct surface (right edge) with infiltrating adenocarcinoma (center) at 4×. c Classic finding of perineural invasion on H&E tissue specimen at 10×. [Courtesy of Dr. Kalpana Devaraj, University of Colorado, Anschutz Medical Campus]
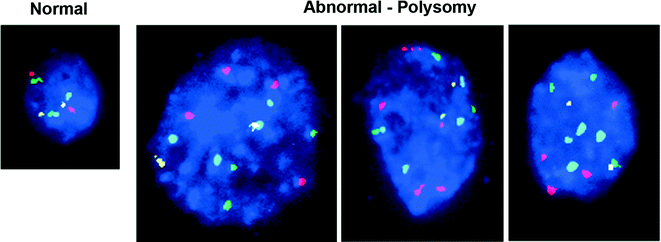
Fig. 15.3
Bile duct brushing fluorescence in situ hybridization: Each color corresponds to a probe specific for the centromere of a particular chromosome. A normal cell compared to malignant cholangiocarcinoma cells demonstrating polysomy; three or more copies of at least two of four probes. [Courtesy of Colorado Genetics Laboratory, University of Colorado, Anschutz Medical Campus]
15.5 Molecular Pathogenesis
As may be assumed from the risk factors associated with the development of cholangiocarcinoma, inflammation is thought to be the key driver of pathogenesis in this malignancy. In addition, activation of signaling pathways related to these inflammatory processes is considered an associated contributor.
Oxidative stress has been identified as a likely mediator of inflammatory carcinogenesis in cholangiocarcinoma, as evidenced by the identification of oxidized biomolecules in cholangiocarcinoma tissues [44], and activation of anti-apoptosis pathways in cholangiocyte cells exposed to such molecules [45]. Generation of such molecules has been related to activation by pro-inflammatory cytokines in chronic inflammatory states and is thought to cause direct damage to DNA and proteins [46]. In addition, inflammatory activation of cyclooxygenase-2 (COX-2) expression has been linked to activation of the epidermal growth factor receptor (EGFR) and downstream pathways associated with cancer cell growth and invasion [47].
It is thought that clonal expansion of those cells that are able to survive under oxidative stress conditions ultimately leads to malignant transformation to cholangiocarcinoma. Studies of cholangiocyte cell lines resistant to hydrogen peroxide demonstrated a higher proliferation rate, pseudopodia formation, loss of cell-to-cell adhesion, and increased expression of DNA methyltransferase-1, supporting this hypothesis [44].
Interestingly, it has recently been determined that in the setting of intrahepatic disease, these processes of cholangiocarcinoma development may affect not only cholangiocytes as precursor cells, but also mature hepatocytes. This concept is supported by the malignant transformation of mature hepatocytes into intrahepatic cholangiocarcinoma cells in the setting of overexpression of activated Notch1 and AKT in preclinical models [48, 49]. Similar studies have confirmed biliary epithelial cells as a progenitor of intrahepatic cholangiocarcinoma as well [50], indicating this subset of cholangiocarcinoma may originate from various cell types, and that Notch pathway activation plays an important role in cholangiocarcinoma pathogenesis.
Somatic mutations associated with aberrant cell signaling have also been associated with cholangiocarcinoma pathogenesis. Recent advances in molecular profiling through next-generation sequencing have led to the identification of distinct profiles for intrahepatic and extrahepatic diseases [51–53]. While mutations in KRAS, CDKN2B (responsible for cell cycle regulation), and ARID1A (associated with chromatin remodeling) are documented with some frequency in both intrahepatic and extrahepatic cholangiocarcinomas, IDH1/2 mutations and FGFR1–3 fusions and amplifications are most often found in intrahepatic disease [53]. In addition, extrahepatic cholangiocarcinoma is associated with a higher frequency of TP53 and ERBB2 mutation [52]. As these studies did not distinguish hilar disease in their analyses, the genetic profile of this cholangiocarcinoma subtype remains unclear at this time.
In addition to these molecular alterations, ROS kinase fusions have been documented in 9% of resected cholangiocarcinomas [54]. Though reported in a small study limited to 23 Chinese patients, this finding remains of interest given the targetable nature of this mutation with existing therapies. Targeting of this and other molecular aberrations identified in both intrahepatic and extrahepatic diseases is currently being evaluated in clinical trials.
15.6 Basic Principles of Staging
15.6.1 Intrahepatic Cholangiocarcinoma
The staging of intrahepatic cholangiocarcinoma has evolved in recent years, moving away from staging systems based on hepatocellular carcinoma toward a unique system designed to provide a more specific prognostic assessment. This transition has resulted in the development of four proposed systems: Okabayashi et al. [55], Liver Cancer Study Group of Japan (LCSGJ) [56], Nathan et al. [57], and AJCC/UICC 7th Edition (Table 15.1) [58]. Though generally similar, the four systems differ on the specific prognostic factors used to determine tumor stage. While all four include tumor number, vascular invasion, and lymph node metastases as key determinants, the LCSGJ system also incorporates tumor size and serosal invasion, the Nathan et al. [59] system includes extrahepatic extension, and the AJCC classification system involves periductal and visceral peritoneal invasion. A comparison of these four systems has been performed in 163 patients with intrahepatic cholangiocarcinoma, with only the AJCC system found to significantly correlate stage with clinical outcome in this study [60]. Still, none of the staging systems addresses resectability, which significantly affects outcome and prognosis as the only curative treatment for this malignancy.
Table 15.1
AJCC/UICC staging for intrahepatic and hilar cholangiocarcinomas
Intrahepatic cholangiocarcinoma | Hilar cholangiocarcinoma | |
---|---|---|
Primary tumor | ||
T1 | Solitary tumor without vascular invasion | Tumor confined to the bile duct, extension up to the muscle layer or fibrous tissue |
T2a | Solitary tumor with vascular invasion | Tumor invades beyond the wall of the bile duct to surrounding adipose tissue |
T2b | Multiple tumors, with or without vascular invasion | Tumor invades adjacent hepatic parenchyma |
T3 | Tumor perforating the visceral peritoneum or involving extrahepatic structures through direct invasion | Tumor invades unilateral branches of the portal vein or hepatic artery |
T4 | Tumor with periductal invasion | Tumor invades main portal vein or its branches bilaterally; or the common hepatic artery; or the second-order biliary radicals bilaterally; or unilateral second-order biliary radicals with contralateral portal vein or hepatic artery involvement |
Regional lymph nodes | ||
N0 | No regional lymph node metastasis | No regional lymph node metastasis |
N1 | Regional lymph node metastasis | Regional lymph node metastasis (including nodes along the cystic duct, common bile duct, hepatic artery, and portal vein) |
N2 | – | Metastasis to periaortic, pericaval, superior mesenteric artery, and/or celiac artery lymph nodes |
Distant metastasis | ||
M0 | No distant metastasis | No distant metastasis |
M1 | Distant metastasis | Distant metastasis |
Stage | ||
I | T1N0M0 | T1N0M0 |
II | T2N0M0 | T2N0M0 |
III | T3N0M0 | – |
IIIA | – | T3N0M0 |
IIIB | – | T1–3N1M0 |
IVA | T4N0M0, T1–4N1M0 | T4N0–1M0 |
IVB | T1–4N0–1M1 | T1–4N2M0, T1–4N0–2M1 |
15.6.2 Hilar Cholangiocarcinoma
The Bismuth–Corlette classification (Fig. 15.4) has long been used to categorize hilar cholangiocarcinoma based on the extent of hepatic ductal involvement in order to better guide surgical management. Hilar cholangiocarcinomas are divided into four types according to this system: Type I, tumor below the confluence of the left and right hepatic ducts; Type II, tumor reaching the confluence; Type III, tumor occluding the common hepatic and either the right hepatic ducts (Type IIIa) or left hepatic ducts (Type IIIb); Type IV, tumor that involves the confluence and both right and left hepatic ducts or tumors that are multicentric [61]. As this classification does not take prognostic factors into consideration, it is not truly a staging system, but rather a descriptive tool useful in determining management.
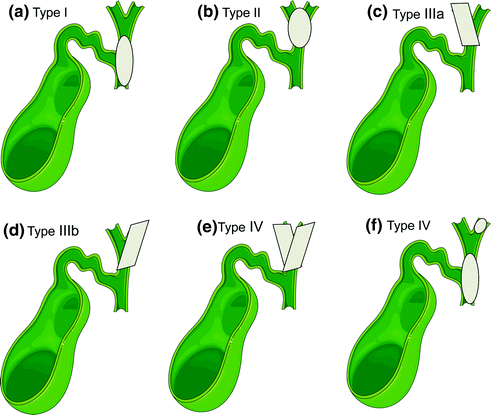
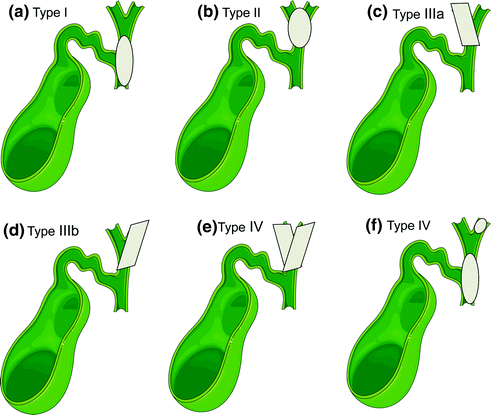
Fig. 15.4
Bismuth–Corlette classification: a Type I, tumor below the confluence of the left and right hepatic ducts; b Type II, tumor reaching the confluence; c Type IIIa, tumor occluding the common hepatic and the right hepatic ducts; d Type IIIb tumor occluding the common hepatic and the left hepatic ducts; e Type IV, tumor that involves the confluence and both right and left hepatic ducts; or f. Type IV, multicentric tumors [Figure created using graphics from http://www.servier.com/Powerpoint-image-bank and PowerPoint. With permission from Creative Commons (https://creativecommons.org/licenses/by/3.0/)]
A separate AJCC/UICC staging system has been developed for hilar cholangiocarcinoma in the 7th Edition (Table 15.1) [58]. This is a significant change from prior AJCC systems in which hilar cholangiocarcinoma was staged as distal cholangiocarcinoma. The current staging system takes into account depth of bile duct invasion, hepatic extension, portal vein and hepatic artery involvement, and nodal and distant metastasis, though does not assess factors important in determining resectability, which are closely tied to disease stratification and outcomes.
Since the current AJCC system does not assess these determinants of resection, additional staging systems for hilar cholangiocarcinoma that incorporate such have been proposed. The Memorial Sloan Kettering Cancer Center (MSKCC) system was the first of this type. This system assigns patients with hilar cholangiocarcinoma to one of the three T-stages according to the extent of ductal involvement by tumor, presence or absence of portal vein involvement, and presence or absence of hepatic lobar atrophy. Though in the original study the resectability rate (p < 0.00001, odds ratio = 0.21 [0.13–0.35]) and R0 resection rate (p < 0.00001, odds ratio = 0.3 [0.18–0.5]) were shown to decrease with increasing T-stage, and T-stage correlated with overall survival [62], later assessment of overall survival according to MSKCC stage in a unique patient data set did not confirm these findings [63]. Limiting factors of this system include lack of assessment of arterial involvement as well as nodal or distant metastatic disease.
A new classification system that takes into account features from each of these previously developed systems has been proposed by the International Cholangiocarcinoma Group for the Staging of Perihilar Cholangiocarcinoma. This system uses the extent of bile duct involvement, tumor size and growth type, portal vein and hepatic artery involvement, liver remnant volume, underlying hepatic disease, and lymph node and distant metastases as factors for determining the stage. The new system remains to be validated, though the group has created an online registry to help achieve this goal [64].
Overall, there have been significant improvements in the systems designed to stage cholangiocarcinoma in recent years, namely creating staging systems specific to the clearly unique entities of intrahepatic and hilar disease. However, there is still a need for a staging system that can accurately provide a pre-surgical assessment of prognosis. Such a system has been proposed for hilar cholangiocarcinoma, though validation is ongoing.
15.7 Treatment of Localized Disease
As the only confirmed curative intervention for the treatment of cholangiocarcinoma, surgical intervention in the form of resection, or in select cases liver transplant, should be considered in all patients with localized disease. Unfortunately, many patients are not candidates for such therapy. Locoregional therapies may provide some benefit in this setting, as will be described.
15.7.1 Surgery
Achieving the goal of complete resection with negative microscopic margins (R0) for intrahepatic cholangiocarcinoma often requires a very extensive surgery, frequently with extended hepatectomy and extrahepatic bile duct reconstruction [65, 66]. Even in patients in whom complete resection is achieved, the risk of recurrence is high, reported in 45–60% of patients [66–68]. Similarly, overall survival rates following resection of intrahepatic cholangiocarcinoma are low, with most documented at or below 50% at 5 years [67–71].
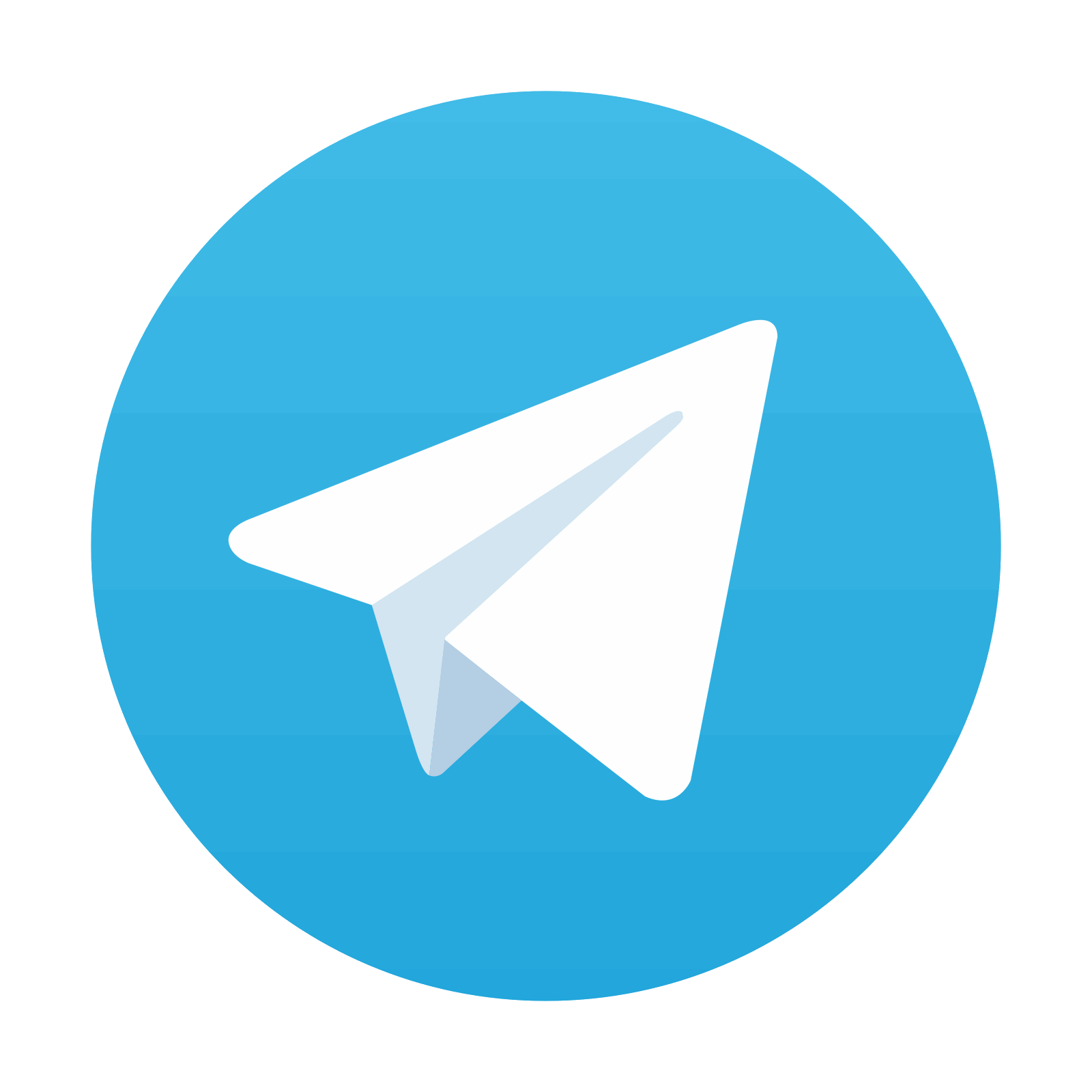
Stay updated, free articles. Join our Telegram channel

Full access? Get Clinical Tree
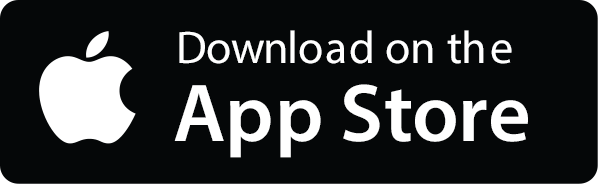
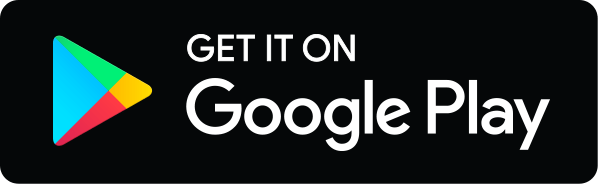