Interpretation of Standard Hematologic Tests
CELLULAR ANALYSIS OF THE PERIPHERAL BLOOD AND BONE MARROW
The practice of hematology is characterized by safe and rapid access and analysis of the cellular elements of blood and bone marrow. Essential clinical information can be derived in less than an hour from a spun hematocrit and microscopic evaluation of peripheral blood or marrow using technology available for more than three quarters of a century,1 while an automated complete blood count, including the hemoglobin concentration, hematocrit, white blood cell and platelet count, white cell differential, and estimates of red cell size, can be provided within minutes.2
Complete Blood Count
Red Cell Parameters
Using traditional methodology, the hematocrit, defined as the packed red cell volume or percent of the blood occupied by red blood cells, was first measured by direct visual inspection of a tube of blood following centrifugation. The hemoglobin concentration and red cell count were also directly measured, by spectrophotometry and use of a counting chamber, respectively. Other red cell indices were indirectly calculated, including the mean corpuscular volume, MCV (hematocrit divided by the red cell count), mean corpuscular hemoglobin, MCH (hemoglobin divided by the red cell count), and mean corpuscular hemoglobin concentration, MCHC (MCH divided by the MCV). Automated cell counters, which directly analyze and count individual cells by changes in impedance or light scatter, provide information for many of the same parameters provided by traditional methods. Automated methods differ in that the hematocrit is derived indirectly, by multiplying the directly measured red cell count and mean corpuscular volume. The hemoglobin and MCV are thus more reliable, reproducible assays than the hematocrit when measured from an automated counter. The MCV is of great clinical utility in evaluating anemia according to red cell size (macrocytic, microcytic, and normocytic anemias), while the RBC count is useful in comparison of iron deficiency and thalassemia. The MCH, which represents the average total hemoglobin content of the red cells, is highly dependent on cell size, while the MCHC represents the average total hemoglobin concentration or degree of “redness” of the red cells. These parameters have less clinical utility than the MCV and RBC count, although the MCHC is classically increased in hereditary spherocytosis.
The RDW, red cell distribution width, is a measure of the variability in size within the red cell population that is provided by automated cell counters. The precise mathematical equation used for calculation varies with the specific instrument, but in all cases elevated values indicate anisocytosis. For casual observers, the RDW is more reliable than is inspection of the blood film for detecting this characteristic.3 An elevated RDW is a valuable clue to abnormalities in red cell morphology; when the RDW is markedly increased, inspection of the blood film is essential. The RDW is sensitive to the presence of small subpopulations of large or small red cells, thus it may be more useful than the MCV for the early detection of nutritional deficiencies. The RDW often remains normal in thalassemia, and the combination of a high or normal RBC count, a low MCV, and a normal RDW is a common pattern in thalassemia trait.4 The use of the RDW in conjunction with other red cell indices has had limited acceptance in the classification of anemias because results may be unreliable in complex settings.
Erythrocytes newly released from the bone marrow contain intracellular RNA, which usually disappears within a day of circulation in the peripheral blood. These young red cells, termed reticulocytes based on the microscopic appearance of RNA-containing reticulum caused by supravital staining, are quantitated by microscopic examination of the peripheral smear. Optical and fluorescent cell-counting methods are also able to detect the uptake of RNA-binding dyes by reticulocyte red cells, and quantitation of the reticulocyte count by automated cell counters has largely replaced the older manual method. Although traditional microscopic and automated techniques produce similar values, the automated technique is more precise. Both methods are vulnerable to interference from intracellular organisms, basophilic stippling, and other artifacts; thus, microscopic inspection of a standard blood film, and/or of a reticulocyte preparation is warranted whenever a reticulocyte count seems inappropriately high for the clinical scenario.
The absolute reticulocyte count (absolute reticulocyte count = % reticulocytes X RBC count/100) is a more clinically useful parameter than the simple percentage of reticulocytes, which is influenced by patient total red cell count and hematocrit. Because a highly stimulated marrow produces larger, more polychromatophilic, RNA-rich reticulocytes (termed stress reticulocytes),5 which are released earlier from the marrow and stain with supravital dyes for up to 3 days after release into the peripheral circulation, the absolute reticulocyte count may overestimate the true rate of reticulocyte production in these clinical situations. Some automated counters may also provide the capability to detect reticulocyte subpopulations containing increased levels of RNA, designated as the immature reticulocyte fraction (IRF), which can indicate the early response to erythropoiesis-stimulating agents and early, pre-myeloid marrow recovery after chemotherapy or stem cell transplantation.6 Automated counters are also capable of selectively measuring the hemoglobin content of reticulocytes, which diminishes rapidly as accessible iron stores are exhausted, and this test may be of use in identifying early iron deficiency in blood donors undergoing repeated phlebotomy, iron-depleted dialysis patients receiving erythropoietin for the treatment of anemia, or iron-stressed women during the late stages of pregnancy.6
White Blood Cell Parameters
The automated white blood cell (WBC) count is measured using the same methods employed to count red cells and platelets. Since the peripheral blood in healthy individuals has approximately 1,000 fold more red cells and 40 fold more platelets than white cells, it is essential for the automated counter to distinguish among cell types. To facilitate the process, red cells are usually destroyed by osmotic lysis and residual red cells or platelets are excluded from the count based on size and granularity. Automated counting is faster, more reproducible, and usually more accurate than the older manual methods, particularly for leukopenic samples in which automated counters can quantitate WBC counts as low as 100 cells per μL.
Automated leukocyte differentials are quite accurate in characterizing white cells from normal controls or patients with qualitatively normal white cell morphology. In patients with hematologic disease or qualitative leukocyte abnormalities, automated differentials may still be accurate, but their reliability cannot be assumed. Thus the white cell differential of new patients with complex clinical findings should also be evaluated on the peripheral smear. The automated absolute granulocyte count plays a reliable and important role in evaluating the risk of infection in neutropenic patients, in grading chemotherapy-induced toxicity, and in following the recovery from hematopoietic stem cell transplantation.
Platelets
Similar to RBC and WBC counts, the automated platelet count is measured by impedance- and/ or optical-based techniques. Platelets are distinguished from red cells by size and in some cases by resistance to osmotic lysis. Automated counters are more accurate than older manual counting methods in monitoring thrombocytopenia, and they often can accurately count less than 10,000 platelets per microliter. On occasion, it may be difficult even with advanced optical techniques to distinguish true platelets from debris derived from precipitated proteins or cell fragments.
The mean platelet volume (MPV) is a parameter that is routinely measured by automated analyzers. The MPV has modest value as a measure of increased platelet turnover and/or activation due to the fact that young platelets are larger than older platelets. However, the MPV may increase rapidly during the first 2 hours after collection because of shape changes and swelling in EDTA, and the reference standards are not adequate for comparing values from different institutions.7
Some automated counters can measure the RNA content of new released platelets, expressed as the immature platelet fraction (IPF) or absolute immature platelet number (per cent IPF times the platelet count). These measurements may be useful clinical parameters in assessing marrow recovery after hematopoietic transplantation and marrow thrombopoiesis in cases of suspected idiopathic thrombocytopenic purpura (ITP).8,9
Sources of Artifact
Clinically relevant artifact at the point of sample collection may occur due to clot formation or due to dilution of blood with intravenous fluid.10,11 Although most automated cell counters are equipped to detect clot formation, some may not be detected, resulting in falsely lower platelet and WBC counts. In contrast, fluid dilution generally is apparent by some degree of change in all three cell lines (red cells, white cells, and platelets) as compared to a previous sample. Both clot formation and dilution may occur more frequently in samples drawn from indwelling catheters.
Artifact may also be introduced by factors associated with the storage of blood samples. When stored at room temperature, absolute RBC, WBC, and platelet counts are stable for up to 3 days, but the red cell MCV increases within 24 hours due to cell swelling, resulting in associated increases in the hematocrit and red cell distribution width.12 After 2 days, the relative proportion of monocytes on the WBC differential also decreases, while relative leukocyte, lymphocyte, and eosinophil counts increase.12
Red cell agglutination within the test tube caused by cold or warm reactive antibodies or rouleaux formation can artifactually reduce the red cell count, while extreme leukocytosis, particularly with a WBC count of more than 500,000/μL, can falsely increase the RBC count and calculated hematocrit.11 Similarly, RBC counts in patients with extremely small or fragmented red cells may be artifactually low. Falsely decreased platelet counts, or pseudothrombocytopenia, caused by platelet clumping and platelet satellitism induced by the EDTA anticoagulant, may occur in up to 0.1% of normal samples and may be present in up to 15% of patients referred for evaluation of a low platelet count.13 In most cases, pseudothrombocytopenia can be eliminated by complete blood count performed on samples collected into a tube containing citrate anticoagulant, in which instance the measured platelet count should be multiplied by 1.1. Platelet clumping may sometimes also falsely elevate the white blood cell count, while small white or red cell fragments, or protein precipitates may artifactually increase the platelet count.10,11
Peripheral Blood Film
One common use of review of the peripheral blood film is by laboratory personnel when values reported by automated counters are “flagged” on the device result print out, or by hematologists in clinical situations circumstances in which verification of the automated result is indicated. The automated value can be compared to a manual estimate obtained by counting the average number of platelets present in 5 to 10 oil immersion high power (1,000×) fields (HPF) and/or the number of leukocytes in a similar number of low power (100×) fields (LPF). The selected fields are chosen from representative regions that contain an even monolayer of cells, avoiding the edge of the film. The platelet count and leukocyte count are estimated by using the formulas:
Platelet count (platelets/mm3) = average platelets per HPF × 15,000
Leukocyte count (cells/mm3) = average leukocytes per LPF × 250
Evaluation of the peripheral blood smear is valuable in the evaluation of hematologic disease and should always be performed in conjunction with review of the bone marrow aspirate and core biopsy. Examination of the peripheral smear is not necessary in simple cases of anemia due to iron, vitamin B12, or folate deficiency. However, it can be very useful in cases that do not respond to therapy and in instances of suspected hemolysis or complex anemias, and it is essential in the evaluation of microangiopathic disorders such as thrombotic thrombocytopenic purpura (TTP). The presence of spherocytes, microspherocytes, schistocytes, targets, sickle cells, red cell membrane irregularities, polychromasia, and intracellular inclusions or parasites should be sought in cases of suspected hemolysis, while tear drop cells and nucleated red cells may be present in marrow fibrosis or tumor invasion. Evaluation of white blood cell abnormalities such as the presence of immature cells and blasts are indicated in cases of suspected malignancy, while other changes such as hypogranulated and hypersegmented granulocytes, Pelger-Huet cells, toxic granulation, and Döhle bodies may point towards a previously unsuspected underlying process.
Review of granulocyte morphology by review of the peripheral smear is superior to automated methods for evaluation of a shift to the left in the myeloid series, or an increased proportion of immature forms, which may be a common finding during the early response to stress or infection. The neutrophil band count is performed manually by grading 1,000 neutrophils on a Romanowsky-stained peripheral blood film, and expressed in percent or as an absolute number.
Routine inspection of platelet morphology is indicated in all patients with newly diagnosed thrombocytopenia in order to exclude platelet clumping and artifactual thrombocytopenia. Review of the blood film may also reveal morphologic abnormalities such as hypogranularity, as in gray platelet syndrome or myelodysplasia, or the presence of very large platelets in inherited syndromes such Bernard-Soulier disease, and May-Hegglin anomaly.
Bone Marrow Aspirate/Biopsy
Indications
Examination of the bone marrow aspirate and biopsy may establish or exclude diagnoses such as aplastic anemia, myelodysplastic syndrome, hemophagocytosis or marrow replacement by non-hematopoietic cells in patients with unexplained persistent or severe thrombocytopenia and granulocytopenia. Bone marrow evaluation is not indicated in cases of isolated anemia in which clinical information and review of the peripheral smear suffice to make the diagnosis. However, in patients with unexplained anemia, particularly if requiring transfusions, a bone marrow study is appropriate. Although marrow aspiration and biopsy are invaluable in hematologic malignancies, and in screening for metastatic spread of non-hematologic malignancies, radiologic or nuclear medicine techniques may be more sensitive methods for detecting metastases. Bone marrow can also be used to culture and identify infectious agents such as mycobacteria and fungi. Disorders such as Gaucher disease or amyloidosis may also be discovered, but biochemical tests for the former or less invasive biopsies for the latter are more useful.
Risks
Bone marrow aspirate biopsy can be performed with minimal discomfort using local anesthesia and is generally very safe when performed by an experienced operator. Informed consent should always be obtained, for the procedure as well as the performance of research studies or specialized laboratory tests. Attention should be paid to both patient comfort, as well as toward obtaining adequate sample volume—particularly of the core biopsy—to conduct the indicated analyses. Infiltration of the skin surface and periosteum with several milliliters of lidocaine provides sufficient anesthesia for most patients; conscious sedation should be reserved for those with significant anxiety and apprehension. Thrombocytopenia is not a contraindication provided adequate landmarks are identified and sufficient direct pressure is applied to the site post-procedure; some patients with platelet function defects or thrombocytopenia due to myelodysplasia may require platelet transfusion for control of bleeding. Anticoagulation should be discontinued or held for an adequate time prior to the procedure, and coagulation factor replacement be utilized for patients with serious factor deficits in order to avoid significant bleeding.
Hemorrhage and local infection occur rarely. In one study of more than 50,000 biopsies, there were 14 instances of serious hemorrhage with one death, and 6 instances requiring transfusion.14 Infection can be safely managed by the use of careful sterile technique and proper local care even in patients with neutropenia and compromised immune function.
In adults, the posterior iliac crest is the site of choice for most hematologists; it can be located using direct palpation with the patient in the prone position and generally provides adequate core and aspirate samples. The anterior iliac crest is a reasonable alternative when obesity, local irradiation, or local skin conditions preclude a posterior approach. Sternal aspiration may be justified when an iliac approach is not possible. However, the sternal approach cannot be used to obtain a core biopsy specimen, may be less well accepted by patients, and is more vulnerable to complications. Traumatic penetration or fracture of the sternum with damage to underlying structures is a rare but serious complication of particular concern when underlying structural damage secondary to malignancy or multiple myeloma is present.
Bone Marrow Aspirate
The bone marrow aspirate is obtained by advancing a specially designed needle fitted with an obturator through the cortex into the medullary space with use of negative pressure to withdraw cells into a syringe after removal of the obturator. The presence of individual marrow particles or particles is determined by visual inspection. The particles are spread on a glass slide, and they may be stained immediately to determine the presence and morphology of cellular marrow elements, such as megakaryocytes or blasts in the evaluation of suspected ITP or leukemia. Cell suspensions obtained from an aspirate may also be used in special studies such as cytogenetic and flow cytometric analyses, while the aspirate clot can also be fixed in a clot section, to be stained with hematoxylin and eosin, or other stains in the same way as a bone marrow biopsy. The marrow may be inaspirable in some patients due to hypercellularity, hypocellularity, fibrosis, or metastatic cancer cells. Aspirates are less reliable than biopsies in detecting marrow involvement with malignancy and not useful at all to detect myelofibrosis or granulomas.
Bone Marrow Biopsy
It is almost always possible to obtain a biopsy specimen, even when hematopoietic cells have been totally replaced by fibrous tissue or tumor. Hematoxylin and eosin-stained biopsy sections show less cytoplasmic and nuclear detail than Romanowsky-stained aspirate films, but provide other essential information about marrow architecture and cellularity. The bone marrow biopsy specimen, or core, is obtained by further advancing the bone marrow biopsy needle through the medullary space after removal of the obturator. In some situations, the core may be obtained from the same puncture site used to obtain the aspirate by advancing deeper into the marrow cortex after obtaining cells for the aspirate; this technique is convenient for the operator and patient but may be associated with artifactual hypocellularity, hemorrhage, and distorted architecture.15 Aspiration artifact may be reduced by obtaining a larger core sample size (1.5–2.5 cm), which may also enhance the evaluation of the marrow composition. The core biopsy sample is ejected from the needle by using a metal probe, fixed, decalcified, sectioned, and stained with hematoxylin and eosin, histochemical stains (for reticulin, collagen, iron), or a variety of immunohistochemical stains.
Marrow Cellularity
Marrow cellularity should be estimated from review of a large bone marrow core biopsy specimen. Because normal marrow cellularity decreases with age; normal percent cellularity can be estimated by subtracting patient age from 100. Inappropriately low cellularity suggests marrow damage, and high cellularity may be consistent with a proliferative disorder, dysplasia, stress reaction, or the use of growth factors.
Megakaryocyte Number and Appearance
A normocellular biopsy and aspirate specimen should contain multiple megakaryocytes per low power (100×) field. An increase in megakaryocytes is consistent with increased turnover secondary to peripheral destruction, inflammation, iron deficiency, or myeloproliferative/myelodysplastic disorders. Reduced megakaryocyte numbers may reflect a primary marrow disease such as aplastic anemia, amegakaryocytic thrombocytopenia, or suppression secondary to chemotherapy. Normal megakaryocytes appear as large cells with multilobated (three or more) attached nuclei. The presence of a substantial number of smaller megakaryocytes with less than three nuclear lobes indicates a shift to the left in megakaryocyte maturity due to increased platelet turnover or dysplasia; megakaryocytes with a single nucleus or multiple small separated nuclei and mature cytoplasm are particularly suggestive of myelodysplasia.
Myeloid to Erythroid Ratio
The myeloid to erythroid (M:E) ratio is quantitated by counting 300 to 500 cells from a marrow aspirate sample. In adults, the normal M:E ratio varies from 1:1 to approximately 3:1. The M:E ratio should be interpreted in the context of overall cellularity, the qualitative appearance of the affected cells, and the clinical setting. Erythroid hyperplasia, characterized by a low M:E ratio in a cellular marrow, suggests an erythroid response to anemia, (especially hemolytic anemia), myelodysplasia, or erythropoietin administration.
Erythroid hypoplasia may indicate red cell aplasia, decreased erythropoiesis due to autoimmunity, medication side effects, or major-ABO incompatible hematopoietic stem cell transplantation. Myeloid hyperplasia may be seen in response to physiologic stress, infection, exogenous growth factors, or in myeloproliferative disorders. Myeloid hypoplasia with a “maturation arrest” (an absence of myeloid precursors beyond the promyelocyte or myelocyte stage) may reflect drug-induced or autoimmune agranulocytosis.
Myeloid and Erythroid Cells
Myeloid and erythroid cell lines should demonstrate normal morphology, a normal distribution of maturation, and synchronous maturation of nucleus and cytoplasm. Disruption of the normal maturation sequence (such as a complete maturation arrest or a preponderance of immature cell forms, or a “shift to the left” of the differentiation pattern), the presence of excess numbers of blasts, or dysplastic changes affecting at least two of the three major hematopoietic cell-lines suggests a serious hematologic disorder. A mild shift to the left and mild megaloblastic changes are more nonspecific.
Lymphocytes and Plasma Cells
There is substantial normal variation in the number of lymphocytes encountered in a marrow specimen, and these elements may be distributed diffusely or in well-defined lymphoid aggregates. Paratrabecular lymphoid aggregates may occur in follicular lymphoma. Benign lymphoid aggregates typically contain more T cells than B cells when immunohistochemical stains are performed, while B cell-rich lymphoid collections are more likely due to clonal B cell lymphoproliferative disorders.
Plasma cells usually constitute less than 2% of marrow cells; increases may be noted in inflammatory disease, benign monoclonal gammopathy, and multiple myeloma. Distinguishing reactive from neoplastic plasma cells in a routine aspirate film may be difficult, as reactive plasmacytosis caused by inflammation or liver disease may reach 20% to 30%, and hypocellular marrows are often rich in plasma cells. Extensive multinuclearity or plasma cell aggregates of more than 5 to 10 cells are suspicious for malignancy, while conspicuous variation in cell size and immature nuclei with nucleoli may be present in myeloma. Immunohistochemical staining for intracellular kappa and lambda chains within plasma cells in the marrow biopsy can indicate the presence of a monoclonal process; distinguishing benign monoclonal gammopathy from multiple myeloma may require additional clinical and laboratory data. The presence of concentrated collections of plasmacytoid lymphocytes suggests Waldenström macroglobulinemia or lymphoplasmacytic lymphoma.
Other Cells Anomalous Present in the Marrow
Malignant cells of epithelial or mesenchymal origin adhere more tightly together and aspirate poorly, forming tightly clumped groups of unusually large cells with a very high nuclear-cytoplasmic ratio. Such clumps may be rare; therefore, when screening an aspirate for malignant cells, the whole slide should be scanned at low power including the leading edge of the film. Tumor cells can also be readily identified in marrow biopsies, and specialized immunohistochemical stains can sometimes help identify the site of origin.
Bone Marrow Iron Stores
When marrow iron is markedly increased, yellow granules of hemosiderin may be seen in routinely stained aspirate and biopsy preparations. The Prussian blue stain, which specifically detects iron, is necessary to evaluate lesser iron stores and iron granules in erythroid cells. Ringed sideroblasts are erythroid precursors containing coarse iron granules immediately surrounding at least half the nuclear circumference due to accumulation of iron within mitochondria. They are always abnormal and imply anomalous porphyrin synthesis secondary to a congenital abnormality, pyridoxine deficiency, exposure to toxins (such as lead or alcohol), medication, or myelodysplasia.
Although tissue iron stores can be assumed to be adequate when stainable marrow iron is demonstrated, this does not indicate that the stores can be mobilized for effective erythropoiesis. For example, when hepcidin levels are elevated by chronic inflammation, iron will be trapped in the macrophages and unavailable for hemoglobin synthesis. In contrast, the absence of stainable iron suggests depleted iron stores. The sensitivity of this method depends heavily on the quantity of sample obtained; for maximal yield, at least 7 separate particles should be evaluated for stainable iron.16 In addition, the absence of stainable marrow iron may incorrectly suggest iron depletion due to hypocellularity, technical error, or presence of cellular antigens binding to antibodies labeled with dyes.17
Additional Studies
Flow cytometry, which allows computerized digital analyses of cells according to size, granularity, and light scattered from colored dyes attached to specific cell markers, is an essential element in the analysis of hematologic malignancy, clonality, and other abnormalities, and can be performed on cells from peripheral blood and marrow.18
Because a very wide variety of possible analyses can be performed, the clinician should specify the clinical question and appropriate differential diagnosis to the laboratory prior to collection to facilitate proper labeling and use of appropriate device settings. As with the automated CBC, flow cytometry is subject to errors and artifacts due to clotting and cell clumping; thus, samples should be carefully collected into appropriately prepared tubes and rapidly and safely transported to the laboratory.
Molecular diagnostic studies such as cytogenetics and fluorescent in situ hybridization (FISH) have an important role in diagnosis and monitoring of hematologic disorders, especially myeloid malignancies, while immunohistochemical stains and molecular analysis of T and B cell receptor rearrangements are critical in documenting clonality in lymphoid malignancies.
SERUM TESTS TO EVALUATE NUTRITIONAL AND HYPOPROLIFERATIVE ANEMIAS
Serum Iron and Total Binding Capacity Assays
Serum iron is measured by automated chemical assays following dissociation from transferrin.19 Total iron binding capacity (TIBC), which is mainly binding to transferrin, can be measured by the addition of excess iron to the sample. Unbound iron is removed by absorption, and the iron bound to protein is again dissociated and measured by the serum iron assay. In many laboratories, unsaturated iron binding capacity is now measured directly by automated chemistry analyzers and the TIBC is calculated.19 Measurement of serum iron can be falsely elevated in specimens containing hemoglobin (hemolysed specimens) and also for many hours transiently after transfusion of older units of red blood cells due to clearance of red cells damaged in storage.20 The percent saturation of transferrin by iron is calculated by dividing the serum iron by the total iron binding capacity and then multiplying by 100.
There is a marked diurnal variation of serum iron in healthy people, with highest levels in the morning; by midnight, serum iron levels can be very low and may fall into the iron deficiency range. Iron levels also vary with the menstrual cycle, with a 10% to 30% increase premenstrually and a similar decrease at the time of menstruation.19
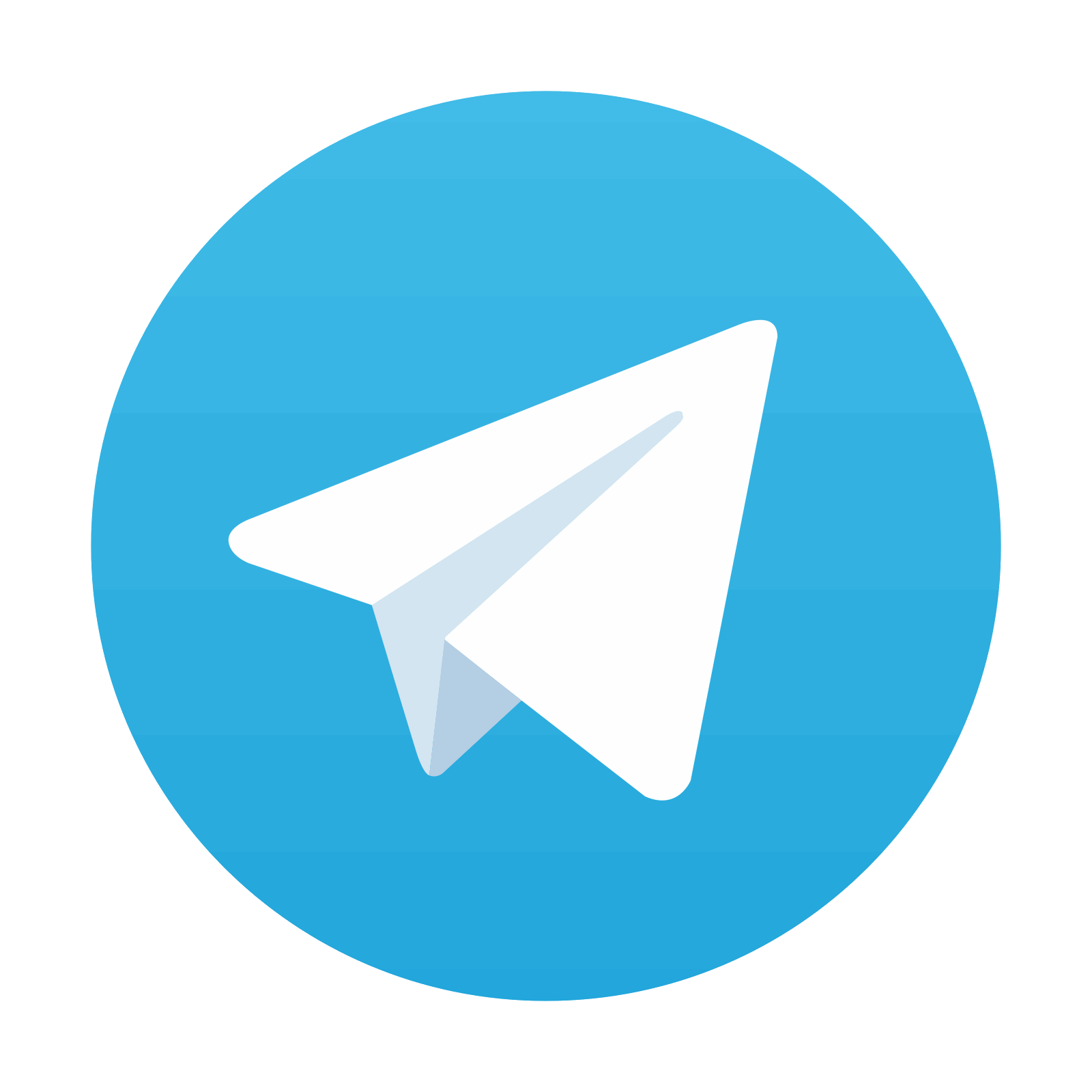
Stay updated, free articles. Join our Telegram channel

Full access? Get Clinical Tree
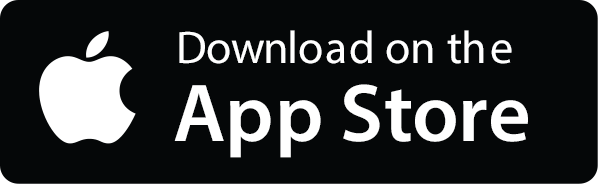
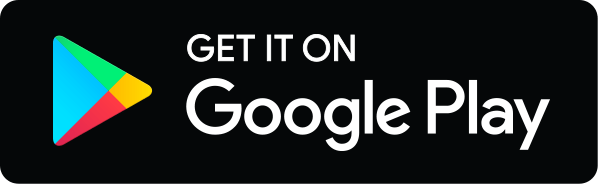