(1)
Institut Bergonié, Bordeaux, France
Abstract
Integrins constitute a family of heterodimeric transmembrane receptors, whose ligands are essentially the proteins of the extracellular matrix (collagens, laminins, fibronectin, etc.). They play a structural role by recruiting inside the cell the cytoskeleton-associated multiprotein complexes responsible for cell–matrix junctions; they also play a functional role, as their activation leads to proliferation and migration signals, via cytoplasmic kinases and small G-proteins. One of the original features of integrins is the bidirectional signalling that characterises their function: from outside to inside the cell, as most signalling pathways, and from inside the cell to outside, aiming at modulating cell attachment to the extracellular matrix as well as the activity of matrix metalloproteinases.
Integrins are, therefore, primarily involved in cell adhesion and motility mechanisms and, via signalling crosstalks, in cell proliferation processes. They play a central role, therefore, in epithelial-to-mesenchymal transition during development. Several integrins are involved in angiogenesis, as they mediate endothelial cells migration and multiplication. A subclass of integrins is essentially involved in immune processes, as they mediate lymphocyte attachment to their target cells. The alterations of integrins are found in diseases affecting the extracellular matrix (rheumatoid arthritis, multiple sclerosis, psoriasis) as well as in the invasiveness and metastatic capacity of endothelial and tumour cells; they represent, therefore, a major potential target in oncology and clinical trials are ongoing.
10.1 Integrins and Integrin Ligands
10.1.1 Structural Organisation of Integrins
Integrins are heterodimeric receptors with a single transmembrane domain per monomer, able to bind the components of the extracellular matrix (ECM) through their extracellular part. With 18 distinct α subunits (genes ITGA) and 8 β subunits (genes ITGB), a total of 24 different integrins can be assembled (Fig. 10.1). The extracellular part of integrins is considerably larger than their intracellular part; it can be represented as an N-terminal globular head above two legs.
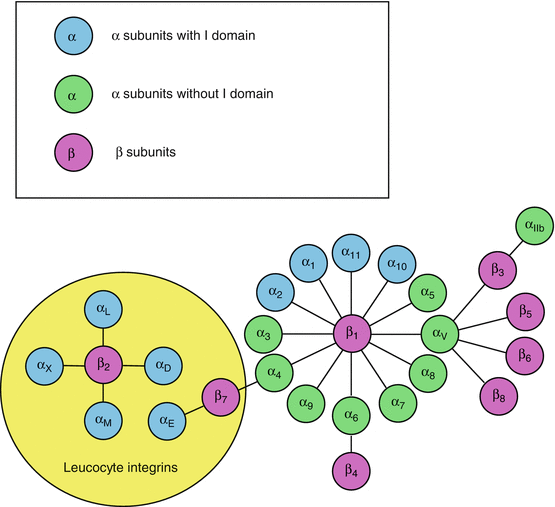
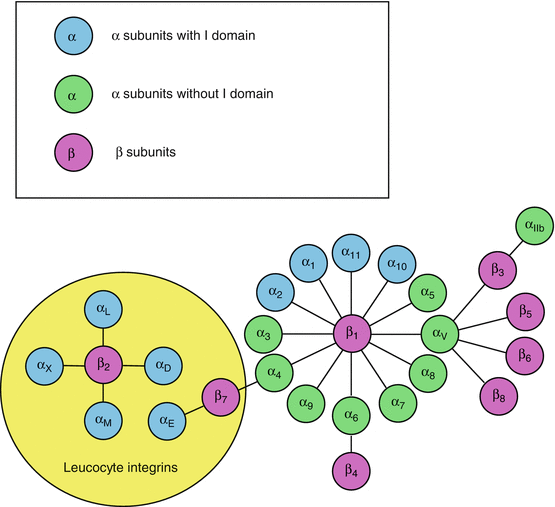
Fig. 10.1
Integrin assembly from their α and β subunits. The 18 α chains and 8 β chains can assemble in diverse ways to form the 24 integrins. Some integrins are specifically expressed in leukocytes and serve as immunoglobulin receptors. Some α chains have an interaction domain with the extracellular matrix (ECM); others do not and signalling operates only with the β-chain interaction (βI) domain
The globular head of the α chains consists of seven identical domains arranged as the blades of a propeller. Some integrins contain in addition, upstream the propeller domain, a domain analogous to type A von Willebrand factor (αI integrins). This domain enables the fixation of a divalent cation, Ca2+ or Mg2+, which interacts with the ECM; it is called the metal ion-dependent adhesion site (MIDAS). Downstream the propeller domain, the α chains contain domains sequentially called thigh, genu, calf1 and calf2, then the transmembrane domain and the intracellular domain (Fig. 10.2a).
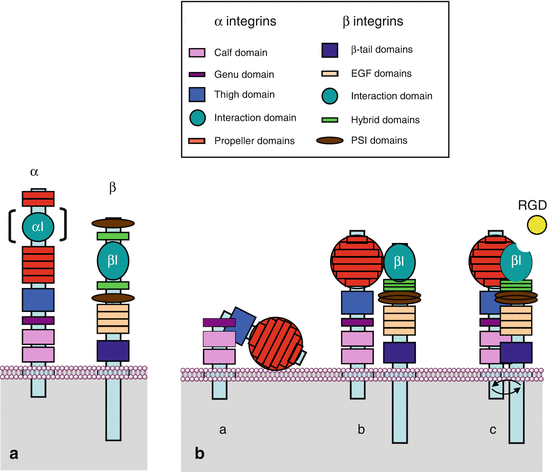
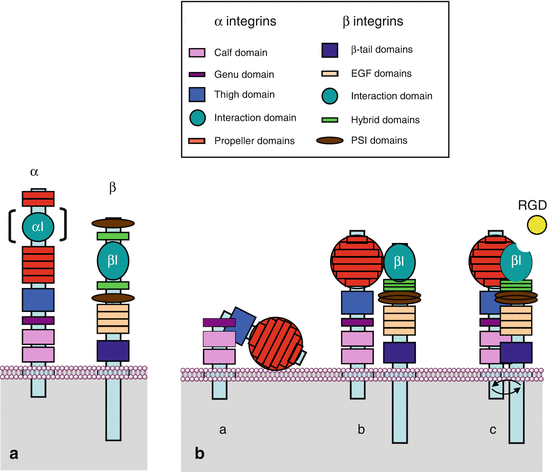
Fig. 10.2
Integrin structure and spatial conformation of α and β chains. (a) Schematic representation of the characteristic domains of the α and β chains of integrins along the polypeptidic sequence. The αI domain of interaction with ECM is not present on all α chains. (b) Spatial representation of integrins. The propeller domains of the α chains are organised in a globular part, the head; the βI domains are exposed following the folding of the hybrid domains over the PSI domains. (a) Integrins adopt a folded conformation in the absence of stimulation; (b) under the effect of an intracellular stimulation (inside-out signalling), integrins adopt an extended conformation; (c) they can thus unmask a binding site with the extracellular matrix (ECM), which allows the interaction between the cytoplasmic domains and the generation of a signal (outside-in signalling)
The β chains also contain a globular head, a βI domain analogous to the αI domain but constant, bearing a MIDAS that follows a folding of the N-terminal extremity; this domain is inserted between two homologous hybrid domains, themselves inserted between two homologous PSI (plexins, semaphorins and integrins) domains. Downstream are four EGF domains, a tail domain (TD), the transmembrane domain and the intracytoplasmic part (Fig. 10.2a).
The intracytoplasmic domains of α and β chains are short but are functionally important; the interaction between these two cytoplasmic domains is stabilised by an ionic bond between an arginine residue of the α chain and an aspartic acid residue of the β chain.
In the absence of stimulation, integrins present a bent conformation, with the globular head lying down on the membrane (Fig. 10.2b). Intracellular messages induce their straightening, allowing them to interact with extracellular ligands. Ligand–integrin binding takes place at the level of the αI domain, for those having such a domain, and at the level of the βI domain for the others. These domains exist under two conformations, one closed, with low affinity for the ligand, and the other one open, with a MIDAS bound to Ca2+ or Mg2+, enabling the formation of a ionic bond with an aspartic acid residue of the ligand (Fig. 10.2b). Binding of integrins to their ligands induces a conformational change of the cytoplasmic domains of the α and β chains. The α chains are constitutively phosphorylated, whereas the β chains display phosphorylation sites allowing the regulation of their activity. Diverse protein kinase C (PKC) enzymes are involved in the phosphorylation of β chains serine/threonine residues, but it appears that tyrosine residues may also be phosphorylated.
An important feature in inside-out signal transduction is the aggregation of several integrin dimers; such a clustering is required in order to increase the attachment of the cell to the ECM. All along the polypeptidic chains of the matrix proteins are present numerous integrin binding sites, requiring a sufficient number of cell adhesion molecules for efficient gripping; these clusters of integrin dimers attached to the ECM are called focal adhesions. Signal transduction arising out of these clusters depends on both affinity changes (conformational switch of the intracytoplasmic domain) and avidity changes (clustering of integrin dimers).
10.1.2 Integrin Ligands
The main extracellular ligands of integrins, considered as receptors, are the ECM proteins: fibrinogen (FG), fibronectin (FN), von Willebrand factor (VWF), collagens (COL), laminin (LAM) and vitronectin (VTN). Some integrins display high ligand specificity, while others are in contrast of low specificity. Particularly, a tripeptidic sequence present in some ECM proteins is specifically recognised by αV integrins: the RGD (arginine–glycine–aspartic acid) sequence. This sequence can serve as a guide for the design of competitive inhibitors of integrins; some snake venoms take advantage of this sequence for the disruption of the ECM. Other integrins recognise different tripeptidic sequences, such as IET or LDV, and some integrins recognise more complex tridimensional structures.
A subgroup of integrins (see Fig. 10.1) are selectively present at the surface of leukocytes, where they participate to immune functions. These integrins, such as αLβ2 or LFA1 (lymphocyte function-associated antigen 1) and αMβ2 or MAC1 (from macrophage), can recognise molecules of the immunoglobulin superfamily, such as ICAMs (intercellular adhesion molecules) and VCAM (vascular cell adhesion molecule). The five ICAMs (ICAM1 to ICAM5) are leukocyte transmembrane immunoglobulins whose binding to integrins allows cell–cell adhesion (see Chap. 11). Other extracellular integrin ligands are bacterial polysaccharides and viral proteins.
On the intracellular side, integrins are able to interact with many proteins involved in cytoskeleton structure regulation; these proteins bind the integrin β chain on the one hand and actin on the other hand, linking thus the ECM to the cytoskeleton. The most important of these proteins are talins (TLN), kindlins (FERMT, for fermitin family homologues), α-actinins (ACTN), filamins (FLN), cytohesins (CYTH), parvins (PARV) and tensins (TNS). These proteins not only are structural proteins, but they display diverse functions which are involved in transduction of signals received and transmitted by integrins. Adapter proteins are also involved in signal transduction: vinculin (VCL), paxillin (PXN) and various 14-3-3 proteins. The layout of integrin clusters with these proteins constitutes the focal adhesions (Fig. 10.3).
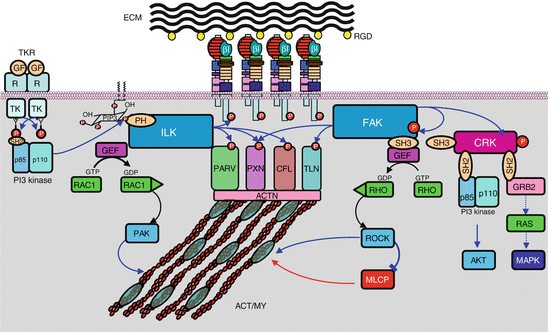
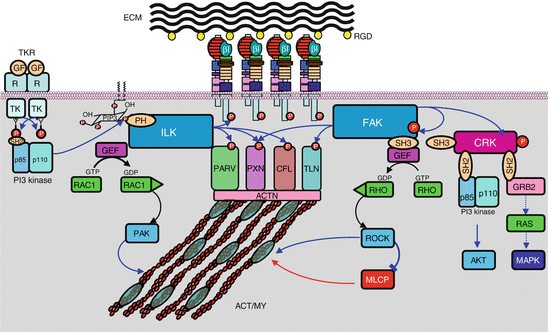
Fig. 10.3
Integrin-activated signalling pathways. A focal adhesion is schematised in the middle of the graph, showing the association between extracellular matrix (ECM) and intracellular actomyosin cytoskeleton (ACT/MY), via the assembly between integrins and several interactants (parvin, paxillin, cofilin, talin, actinin), some of which only are represented here. Two signalling pathways generated by integrin activation are shown: On the left, ILK (integrin-linked kinase), recruited, for instance, by PI3 kinase, phosphorylates the integrin β chains as well as several focal adhesion proteins; ILK also activates exchange factors (GEF) of small G-proteins of the RHO family, such as CDC42 and RAC1, which, once activated by GTP binding, can activate in turn some kinases like PAK, which can directly act on cytoskeleton proteins. On the right, FAK (focal adhesion kinase), recruited through integrin activation, phosphorylates focal adhesion proteins as well as SRC family kinases (SFK). Adapter proteins are able to activate proliferation pathways such as the AKT and the MAP kinases pathway. FAK also activates, via GEFs, small G-proteins of the RHO family, which, once activated, can activate in turn some kinases like ROCK (RHO-activated kinase), which can act on cytoskeleton proteins, either directly or through the phosphatase MLCP (myosin light chain phosphatase)
10.2 Signalling Pathways Arising from Integrins
Integrins operate via two signalling ways: inside-out signalling, allowing intracellular messages to induce extracellular ligand recognition and binding via integrin activation, and outside-in signalling, as all receptors, allowing extracellular messages to induce specific cell responses. Integrins are therefore bidirectional receptors. These two activities are however inseparable: intracellular signals originating especially from TKR or GPCR activation activate first the receptor function of integrins by inducing their straightening, which governs ligand recognition; afterwards, this binding with extracellular ligands activates the formation of focal adhesions clusters and generates various intracellular messages. Integrin activation leads especially, according to cell type and integrin dimer, to the activation of cytoplasmic kinases, among which the tyrosine kinase FAK (focal adhesion kinase) (gene PTK2, protein tyrosine kinase 2) and the serine/threonine kinase ILK (integrin-linked kinase). These kinases are committed to various signalling pathways, leading either to the activation of transcription factors or to the activation of small G-proteins. These pathways are presented on Fig. 10.3.
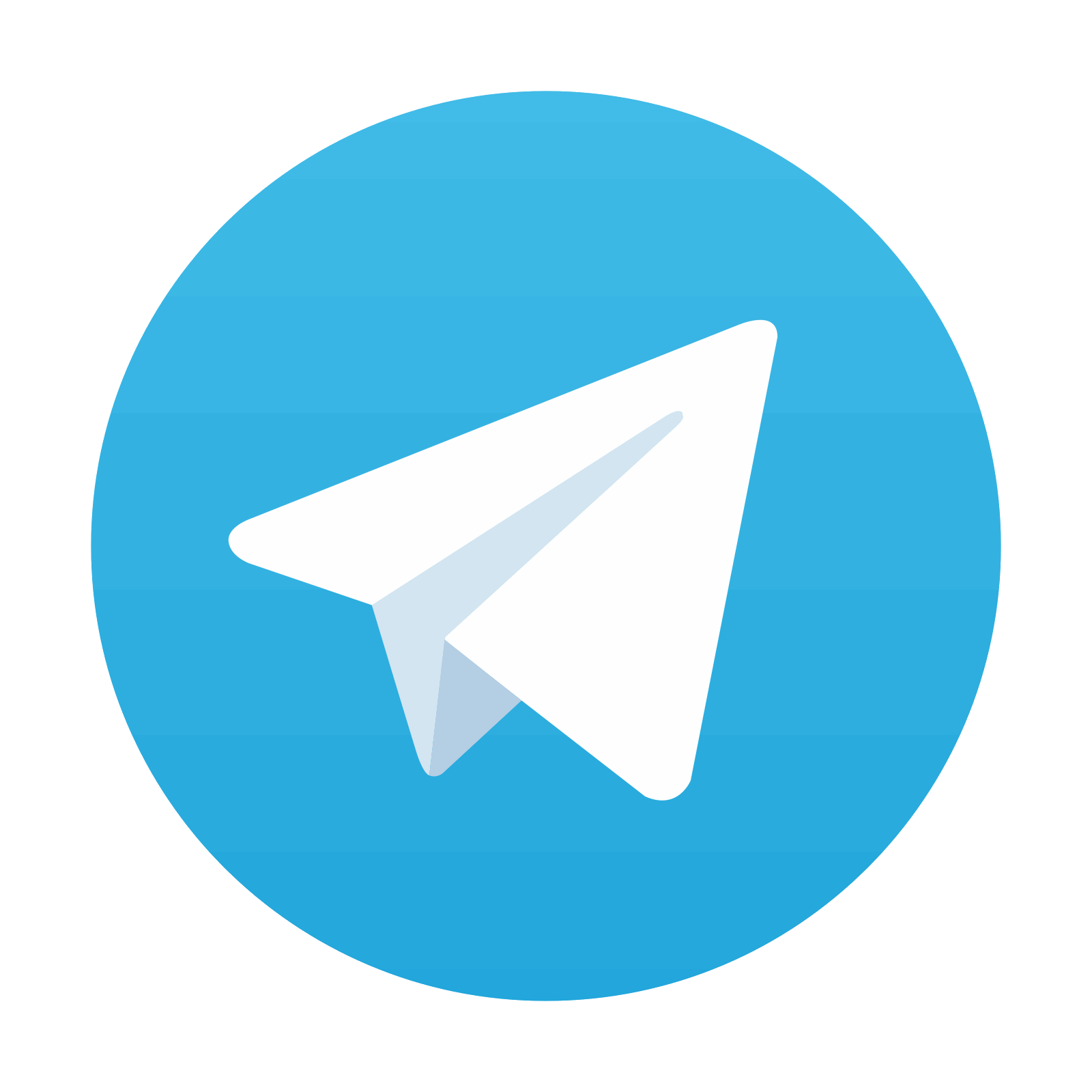
Stay updated, free articles. Join our Telegram channel

Full access? Get Clinical Tree
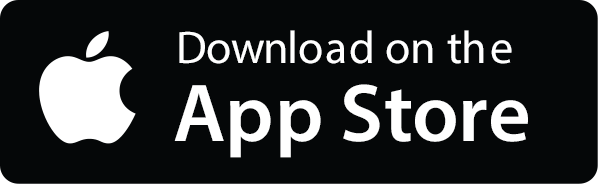
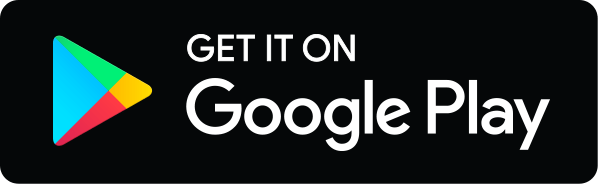