SUMMARY
Rare bleeding disorders (RBDs), accounting for the 3 to 5 percent of patients with abnormal hemostasis, include the nonhemophilia inherited deficiencies of coagulation factor II (prothrombin), factor V, combined factor V/VIII, factor VII, factor X, factor XI, factor XIII, and fibrinogen. The prevalence of RBDs is variable, both the relative frequency among the different factors and frequency in different regions of the world. The genetic transmission of these disorders is usually autosomal recessive. Bleeding manifestations caused by these inherited deficiencies are of variable severity and usually related to the extent of the decreased activity of the particular coagulation factor. Usually, only homozygous and compound heterozygous patients are symptomatic, although occasionally heterozygotes display a bleeding tendency. On the whole, the most typical symptom, common to all RBDs, is the occurrence of mucosal bleeding, whereas life-endangering bleeding, such as central nervous system or umbilical cord bleeding, is more frequent only in the some deficiencies, such as afibrinogenemia and severe factor XIII and factor X deficiencies, characterized by very low or undetectable coagulant activity. Treatment of patients affected with the various coagulation factor deficiencies could be (1) on demand for spontaneous bleeding episodes, (2) after surgical procedures, and (3) for prevention (prophylaxis). Because of the rarity of these disorders and the technical limitations of laboratory testing and the lack of specific concentrates, a unified, evidence-based therapeutic approach to many such patients is not always clear. To overcome these limitations, new strategies, such as the creation of global partnerships and networking between treatment centers, have been developed to increase our knowledge and create platforms for researchers and clinicians to exchange information.
Acronyms and Abbreviations:
aPTT, activated partial thromboplastin time; COPII, coat protein complex II; EGF, epidermal growth factor; ELISA, enzyme-linked immunosorbent assay; ERGIC, endoplasmic reticulum–Golgi intermediate compartment; FFP, fresh-frozen plasma; GGCX, γ-glutamyl carboxylase; Gla, γ-carboxyglutamic acid; LMAN, mannose-binding lectin; MCFD, multiple combined-factor deficiency; PAR, protease-activated receptor; PCC, prothrombin complex concentrate; PPH, postpartum hemorrhage; PT, prothrombin time; TAFI, thrombin-activatable fibrinolysis inhibitor; TF, tissue factor; TT, thrombin time; VKORC1, vitamin K epoxide reductase–oxidase complex.
Rare congenital deficiencies of plasma proteins involved in blood coagulation, such as fibrinogen, prothrombin, and factors V, V+VIII, VII, X, XI, and XIII, generally lead to lifelong bleeding disorders. These disorders have been described in most populations with an incidence varying from one case in 500,000 for factor VII deficiency, to one case in 2 to 3 million for prothrombin and factor XIII deficiency.1,2 However, their relative frequency varies among populations, being higher in regions where consanguineous or endogamous marriages are common, partly as a result of increased high frequencies of specific mutant genes in these inbred populations.3–8 Two large surveys were made by the World Federation of Hemophilia (WFH; www.wfh.org) and the European Network of the Rare Bleeding Disorders (EN-RBD; www.rbdd.eu), with the aim of collecting epidemiologic data and providing information to hemophilia organizations and treatment centers to reduce and prevent complications of bleeding. Data collected by these surveys showed that factor VII and factor XI deficiencies are the most prevalent rare bleeding disorders (RBDs), each accounting for approximately one-third of all RBDs, while the rarest disorders are factor II (prothrombin) deficiency and combined deficiency of factors V and VIII (Table 14–1). The severity of bleeding manifestations in affected patients is variable. The most typical symptom, common to all RBDs, is bleeding from the mucosal tracts or at the site of invasive procedures; life- and limb-endangering symptoms, such as umbilical cord and central nervous system bleeding, recurrent hemarthroses, and soft tissue hematomas, occur with higher frequency only in some severe deficiencies.9–15 Although heterozygotes for the coagulation factor deficiencies usually do not manifest a bleeding tendency, some cases of postdelivery and post–dental-extraction bleeding in heterozygotes for factor X deficiency have been reported.16
Deficiency | WFH Survey (%) | EN-RBD Database (%) |
---|---|---|
Fibrinogen | 7 | 8 |
Factor II | 1 | 1 |
Factor V | 9 | 10 |
Factor V + factor VIII | 3 | 3 |
Factor VII | 36 | 39 |
Factor X | 8 | 8 |
Factor XI | 30 | 24 |
Factor XIII | 6 | 7 |
The complexity of blood coagulation and the large number of proteins and nonprotein substances involved necessitate that a global test be used to simply and reproducibly assess its function: the screening tests such as prothrombin time (PT) and activated partial thromboplastin time (aPTT) are the first step in evaluating patients reporting a clinical and family history of bleeding. The PT interrogates the extrinsic coagulation pathway, and its prolongation is indicative of the deficiency of factor VII; a normal aPTT is highly dependent on the intrinsic coagulation pathway, so that its prolongation is indicative of deficiencies of factors XI, VIII, IX, and XII. However, all patients homozygous or compound heterozygous for factor XI deficiency have aPTT values longer than 2 SD above the normal mean,17 while heterozygotes substantially overlap the normal range.17,18 The prolongation of both the PT and aPTT indicates the lack of a factor belonging to the common pathway, including prothrombin, factor V, or factor X. However, patients with factor X deficiency harboring mutations that cause a defect only in the tissue factor (TF) pathway will only display a prolonged PT, and their aPTT will be normal. Other patients who carry mutations that only affect the intrinsic pathway activity of factor X will exhibit a normal PT and prolonged aPTT.19 Abnormal results of either of these screening tests should be followed by mixing studies where equal amounts of patient plasma and normal plasma are mixed and retested; the relevant test time is normalized in patients with factor deficiencies but is not corrected or only minimally corrected in patients with factor inhibitors. In case of correction, specific coagulation assays are then performed to make the diagnosis of the specific factor deficiency. To evaluate fibrinogen deficiency, all coagulation tests that depend on the formation of fibrin as the end point are necessary; hence, in addition to PT and aPTT, thrombin time (TT) has to also be performed. In factor XIII deficiency, PT and aPTT are normal. Diagnosis of factor XIII deficiency is established by demonstrating increased clot solubility in 5 M urea, dilute monochloroacetic acid, or acetic acid. However, this method, quantitative and not yet standardized, detects only severe factor XIII deficiency (with activity <5 percent), thus leading to a possible underdiagnosis of factor XIII deficiency. The factor XIII deficiency diagnosis protocol requires a number of assays, which test for both activity as well as antigen levels. In the case of estimation of factor XIII activity using quantitative (e.g., photometric assays, which measure the ammonia released during a transglutaminase reaction) or incorporation assays (dansylcadaverine-casein assay, which measures the level of incorporation of a labeled amine into a protein substrate) during transglutaminase-mediated cross linking,20 the plasma blanking procedure is mandatory to avoid the factor XIIIa–independent ammonia release that could lead to incorrect results in the low-activity range (below 5 to 10 percent).21,22 Factor XIII A-subunit antigen can be measured by enzyme-linked immunosorbent assay (ELISA).23 Factor antigen assays are not strictly necessary for diagnosis and treatment but are necessary to distinguish type I from type II deficiencies that become very important in fibrinogen or prothrombin deficiency, where normal antigen levels and reduced coagulant activity (dysfibrinogenemia and dysprothrombinemia) are associated with higher risk of thrombosis. Hereditary factor V deficiency is also a peculiar case that can be confused with combined deficiency of factor V + factor VIII because the two entities have similar manifestations and are characterized by prolonged PT and aPTT. Consequently, assays of factor V and factor VIII are mandatory for making the distinction.
The development of guidelines for classification of RBDs has been historically hampered by a lack of sufficient knowledge about epidemiology and clinical outcomes, the difficulty in recognizing affected patients and collecting longitudinal clinical data, the limits of laboratory assays, and a lack of consensus concerning the criteria by which these disorders are classified. Classification of RBDs based on the residual level of plasma coagulant activity of the missing factor has considered for many years all RBDs as a single entity, and a mild, moderate, or severe classification as in hemophilia was adopted (except for some disorders such as afibrinogenemia and factor XIII deficiency). In 2012, the Rare Bleeding Disorders Working Group, under the umbrella of the Factor VIII & Factor IX Scientific and Standardisation Committee (SSC) of the International Society on Thrombosis and Haemostasis (ISTH), analyzed the results of data coming from four registries (EN-RBD, the United Kingdom Haemophilia Centre Doctors’ Organization registry, the North American Rare Bleeding Disorders Registry, and the Indian registry) including a total of 4359 patients. Despite the large number of patients evaluated in this overview (both from the literature and the aforementioned registries), there is a large heterogeneity in the preassigned severity definitions for both coagulant activity and bleeding symptoms.24 At the same time, the EN-RBD, based on a cross-sectional study using data from 489 patients and involving 13 European treatment centers, for the first time evaluated the correlation between the coagulant residual plasma activity level and clinical bleeding severity in each RBD. Clinical bleeding episodes were classified into four categories of severity based on the location and the potential clinical impact, as well as the trigger of bleeding (spontaneous, after trauma, or drug induced). By means of linear regression analysis, this study found a strong association between coagulant activity level and clinical bleeding severity for fibrinogen, combined factors V + VIII, X, and XIII deficiencies. A weak association with clinical bleeding severity was present for factors V and VII deficiencies, while coagulation activity level of factor XI did not predict clinical bleeding severity. From the same study, it also clear that the minimum level to ensure complete absence of clinical symptoms is different for each disorder, leading to the conclusion that RBDs should not be considered as a single class of disorders, but instead studies should focus on the evaluation of specific aspects of each single RBD and different from hemophilia.25
The molecular diagnosis of RBDs is based on the mutation search in the genes encoding the corresponding coagulation factor. Exceptions are the combined deficiency of coagulation factors V+VIII, caused by mutations in genes encoding proteins involved in the factor V and factor VIII intracellular transport (multiple combined-factor deficiency [MCFD] 2 and mannose-binding lectin [LMAN] 1) and the combined deficiency of vitamin K–dependent proteins (prothrombin and factors VII, IX, and X), caused by mutations in genes that encode enzymes involved in posttranslational modifications26 and in vitamin K metabolism (γ-glutamyl carboxylase [GGCX] and vitamin K epoxide reductase–oxidase complex [VKORC1]).27 Coagulant factors genes are located on different chromosomes except for the genes of factor VII (F7), factor X (F10), fibrinogen (FGA, FGB, FGG), and factor XI (F11) (Table 14–2). In particular, F10 lays only 2.8 kb downstream of F7; thus, the combined deficiency of the two factors can be also the result of chromosomal abnormalities of the long arm of chromosome 13.28–30 The strategy for molecular analysis is generally based on polymerase chain reaction amplification followed by Sanger sequencing of all exons, flanking intronic sequence and 5′ and 3′ untranslated regions. In contrast with hemophilia A, caused in approximately half of the patients by an inversion mutation involving introns 1 or 22 of the factor VIII gene, RBDs are often caused by mutations unique for each kindred and scattered throughout the genes. Information on already identified mutations causing RBDs is traceable from the mutation database on the ISTH website (http://www.isth.org/?MutationsRareBleedin). Missense mutations are the most frequent gene abnormalities, representing 50 to 80 percent of all identified mutations, except for LMAN1 variants where the most frequent mutations are insertions/deletions (50 percent). Insertion/deletion mutations represent 20 to 30 percent of the gene variations of the fibrinogen, factor V (F5), MCFD2, and factor XIII (F13A) genes, and less than 15 percent of the remaining coagulation factor gene mutations. Splicing and nonsense mutations comprise 5 to 15 percent of all identified mutations in all coagulation factors, with a maximum rate of 20 percent in the LMAN1 gene. Variants located in the 3′ and 5′ untranslated regions of the genes are the least-frequent types of mutation (<5 percent), found only at the fibrinogen, factor VII, factor XI, and factor XIII loci. The combined presence of more than one recessively transmitted coagulation factor defect may also rarely occur, resulting in combined deficiency of factors VII and X31–33 and combined deficiency of factors VII and V, VIII, X, or XI.34 Despite significant advances in our knowledge of the genetic basis of the RBDs, in 5 to 10 percent of patients affected with severe clotting factor deficiencies, no genetic defect can be found. In these patients, the use of next-generation sequencing might help to identify novel pathways in coagulation disorders.
Treatment of RBDs is a difficult task because the absence of longitudinal clinical data and the limitations of available laboratory assays make it difficult to develop evidenced-based guidelines for the diagnosis and treatment of RBDs. A patient’s personal and family history of bleeding is an important guide for management. Dosages and frequency of treatment depend on the minimal hemostatic level of the deficient factor, its plasma half-life (see Table 14–3), and the type of bleeding episode. At variance with patients affected with hemophilia A or B who have vastly improved the quality of life from advances in the manufacture of safe and effective products,35 patients with RBDs have seen less progress. The main treatments in RBDs are represented by replacement therapy of the deficient coagulation factor and nontransfusional adjuvant therapies (antifibrinolytic amino acids, estrogen/progestin). Fresh-frozen plasma (FFP) and cryoprecipitate are the backbone of RBD treatment, particularly in those countries with low economic resources. However, specific plasma-derived concentrates are currently available only for fibrinogen and factors VII, XI, and XIII, and they are licensed only in some European countries; replacement therapy of coagulation factors may require the prescription of unlicensed products that are not readily available.
Deficient Factor | Plasma Half-Life | Recommended Trough Levels | On-Demand Dosages | Recommended Trough Levels After Publication of the EN-RBD Results to Maintain Patient Asymptomatic |
---|---|---|---|---|
Fibrinogen | 2–4 days | 0.5–1.0 g/L | Cryoprecipitate (5–10 bags) SD-treated plasma (15–30 mL/kg) Fibrinogen concentrate (50–100 mg/kg) | 1 g/L |
Prothrombin | 3–4 days | 20–30% | SD-treated plasma (15–20 mL/kg) PCC (20–30 units/kg) with dosing based on labeled factor IX units | >10% |
Factor V | 36 hours | 10–20% | SD-treated plasma (15–20 mL/kg) | 10% |
Factors V and VIII | Factor V 36 hours Factor VIII 10–14 hours | 10–15% | As for factor V | 40% |
Factor VII | 4–6 hours | 10–15% | Factor VII concentrate (30–40 mL/kg) PCC (20–30 units/kg) rFVIIa (15–30 mcg/kg every 4–6 hours) | >20% |
Factor X | 40–60 hours | 10–20% | SD-treated plasma (10–20 mL/kg) PCC (20–30 units/kg) Factor X/factor IX concentrate (10–20 units/kg) | >40% |
Factor XI | 50 hours | 15–20% | SD-treated plasma (15–20 mL/kg) Factor XI concentrate (15–20 units/kg) | 15–20% |
Factor XIII | 9–12 days | 2–5% | Cryoprecipitate (2–3 bags) SD-treated plasma (3 mL/kg) Factor XIII concentrate (50 units/kg for high hemorrhagic events) | 30% |
Prothrombin and factor X deficiencies are often treated with prothrombin complex concentrates (PCCs), which often also contain uncontrolled amounts of factor II, factor VII, and factor X. Products to cover the need for a dedicated therapy of patients with factor V deficiency and to facilitate the prophylaxis scheme in patients with factor X deficiency are of recent production. Finally, only two recombinant products are currently available for treatment of RBDs: recombinant factor VIIa (rFVIIa;) and recombinant factor XIII (rFXIII) (see “Factor VII Deficiency” and “Factor XIII Deficiency”). Although there are a number of reports available in the literature reporting on treatment on demand and by prophylaxis in RBDs,36,37 no clear-cut guidelines are yet available apart from those of the United Kingdom Haemophilia Centre Doctors’ Organization.38 Table 14–3 shows available treatment for each deficiency and suggested dosages.
Women with RBDs require specific attention and care because in addition to experiencing the common associated bleeding symptoms, they may also experience bleeding complications from regular hemostatic challenges during menstruation, pregnancy, and childbirth, as well as from other gynecologic conditions, such as hemorrhagic ovarian cysts, endometriosis, hyperplasia, polyps, and fibroids. Menorrhagia, defined as blood loss of more than 80 mL per menstruation, is reported to be one of the most important symptoms in women with RBDs.39,40 Menstruation may be quite problematic for women with coagulation disorders who have excessive blood loss, which can have a major impact on their quality of life and employment.
Pregnancy and childbirth pose particular clinical challenges to women with RBDs, because, apart from factor XI deficiency, detailed information about these issues and their management is very scarce and limited to just a few case reports.41,42 Pregnancy is accompanied by increased concentrations of fibrinogen, factor VII, factor VIII, factor X, and von Willebrand factor, which are particularly marked in the third trimester.43–47 In contrast, prothrombin, factor V, factor IX, and factor XIII are relatively unchanged.43 All of these changes contribute to the hypercoagulable state of pregnancy; however, women with coagulation factor deficiencies do not achieve the same factor levels as those of women without deficiencies,39 increasing the possibility of pregnancy loss or bleeding complications, especially if the defect is severe.
Inherited prothrombin deficiency is one of the rarest coagulation factor deficiencies. It presents in two forms: type I, true deficiency (hypoprothrombinemia), and type II, in which a dysfunctional prothrombin is produced (dysprothrombinemia). These autosomal recessive disorders are genetically heterogeneous and characterized by a mild to moderate bleeding tendency. Both types of prothrombin deficiency impair the generation or function of thrombin, the central enzyme of the blood coagulation system.
Prothrombin, approximate Mr 72,000, is structurally homologous with other members of the vitamin K–dependent proteins, factors VII, IX, and X, proteins C, S, and Z, and bone γ-carboxyglutamic acid (Gla) protein. Prothrombin is synthesized in the liver as a prepropeptide of 622 amino acids, and its plasma concentration is 100 to 150 mcg/mL. The circulating protein in its mature form is a single-chain glycoprotein of 579 residues, composed of the Gla domain (residues 1 to 37) and the catalytic domain (residues 272 to 579), where a light A chain is disulfide-bonded to the heavy B chain containing the catalytic triad. In the zymogen molecule, there are several exodomains, such as two kringle domains—kringle 1 (F1; residues 38 to 155), kringle 2 (F2; residues 156 to 271)—and the prepropeptide region.48,49 The prepropeptide domain is responsible for protein processing, targeting, and carboxylation, and it is removed prior to secretion from the cell. The Gla domain constitutes the aminoterminus of the mature prothrombin molecule and contains the 10 glutamic acid residues that are posttranslationally modified through action of vitamin K–dependent carboxylase to Gla. As a result of this modification, prothrombin acquires the capacity to bind calcium and membranes containing acidic phospholipids. The kringle domain contains two extensively folded, disulfide-bonded “kringle” motifs. They are present in diverse proteins and are thought to mediate protein–protein interactions. For example, the kringle 2 domain of prothrombin mediates interaction of prothrombin with activated factor V (Va).50 The catalytic domain contains the enzyme active site, which is responsible for fibrinogen cleavage. The residues characteristic for the serine protease family, His363, Asp419, and Ser525, constitute a charge relay system responsible for bond cleavage. The crystal structure of prothrombin has not been determined, but the crystal structure of human α-thrombin complexed with D-Phe-Pro-Arg chloromethylketone (an inhibitor that is a transition state analogue covalently bound to the enzyme) has been determined.51
Prothrombin plays a central role in coagulation, functioning in both TF and contact activation pathways. Prothrombin is converted to its proteolytically active form, thrombin, by the prothrombinase complex consisting of activated factor X (Xa), factor Va, and phospholipid surface of platelets and other cells. Two forms of thrombin are generated: meizothrombin, if prothrombin is cleaved at residue 320, and α-thrombin, if cleavage occurs first at residue 271, removing prothrombin fragment 1.2, and subsequently cleaved at residue 320. Thrombin is a multifunctional serine protease. In addition to converting fibrinogen to fibrin, thrombin also exerts functions in the coagulation cascade, consisting of both pro- and anticoagulant effects52 and activates platelets by cleavage of the protease-activated receptor (PAR)-1 and PAR-4, initiating signals leading to platelet adhesion and aggregation.53,54 Thrombin also stimulates wound healing through its action as a growth factor and its proangiogenic activity.55
The prothrombin gene is located on the short (p) arm of chromosome 11.56 It is 20-kb long and consists of 14 exons separated by 13 introns. Fifty-four mutations that cause prothrombin deficiency have been identified, of which 42 are missense, three nonsense, seven deletions/insertions, and two splicing mutations (see mutation database on the ISTH website, http://www.isth.org/?MutationsRareBleedin and Ref. 9). Type II deficiency (dysprothrombinemia) results from missense mutations that are located throughout the gene. As expected, many mutations are in the catalytic domain, imparting catalytic dysfunction on thrombin. Other mutations give rise to abnormally slow activation of prothrombin. Only about 10 mutations were identified in patients with type I deficiency, of which five were present in homozygotes. Globally, there is a clear prevalence of patients with a Latin/Hispanic origin, as nearly 70 percent of all patients with thrombin gene defects come from such areas (Barcelona, Padua, Segovia, and Puerto Rico).9
A number of polymorphisms have been identified in the prothrombin gene. One of these polymorphisms, a G>A change at nucleotide 20210 in the 3′ untranslated region of the prothrombin gene, is associated with increased plasma levels of prothrombin and an increased tendency to venous thrombosis (Chap. 20).57
According to a recent classification by the SSC of the ISTH, prothrombin deficiency may be classified as severe, moderate, and mild, corresponding to blood levels of less than 5 percent, 5 to 10 percent, and greater than 10 percent, respectively.24 In severely prothrombin-deficient patients, bleeding may be marked, including spontaneous hemarthroses; less severe patients may show mild to moderate mucocutaneous and soft tissue bleeding that usually correlates with the degree of functional prothrombin deficiency. Heterozygous subjects, having plasma prothrombin levels between 30 and 60 percent of normal, are usually asymptomatic; however, occasionally, excessive bleeding after moderate-intensity trauma, tooth extractions, or surgical procedures may occur. Patients with dysprothrombinemia show a variable bleeding tendency that is usually less severe than in type I deficiency. Women with prothrombin deficiency may suffer from menorrhagia. Because of the extreme rarity of such deficiency, reports on events during pregnancy/delivery are very scarce, with only one described in four of eight pregnancies in a hypoprothrombinemic woman.58 In the same report, one postpartum hemorrhage (PPH) episode in the four term pregnancies was reported, despite administration of clotting factor concentrate. However, these data were not confirmed by a following Iranian series, including a total of 14 patients with the same deficiency (coagulant activity levels 4 to 10 percent).59
Undetectable plasma prothrombin probably is incompatible with life, as inferred from the partial embryonic and neonatal lethality of prothrombin knockout mice, which do not survive to adulthood.60,61
Replacement therapy is needed only in severe patients, in case of bleeding or to ensure adequate prophylaxis before surgical procedures. In severe clinical settings, higher levels of prothrombin may be achieved with FFP, or with PCCs, which avoids the risk of volume overload sometimes associated with the use of FFP.62 However, PCCs contain other vitamin K–dependent coagulation factors (VII, IX, and X) and small amounts of their activated forms, which could potentially induce thrombotic complications; those containing an amount of factor VII below 10 percent are commonly known as three-factor PCCs. These concentrates are heated or treated with solvent–detergent, processes that remove HIV, hepatitis B, hepatitis C, and other viruses but that do not remove parvovirus B19 or hepatitis A virus63–65; the latter viruses can be effectively removed by dry heat and nanofiltration.66 However, transmission of other possible bloodborne agents, such as prions causing Creutzfeldt-Jakob disease and its new variant, has not been totally eliminated. Bruises and mild superficial bleeding generally do not require replacement therapy. Antifibrinolytic agents (tranexamic acid and gabexate mesylate) have also been used for minor surgical procedures. The oral contraceptives have been shown to exert beneficial effects on menometrorrhagia in women characterized by prothrombin coagulant levels less than 3 percent.9 Thromboprophylaxis in dysprothrombinemic patients considered at high risk for a thrombotic event (e.g., orthopedic surgery) is a controversial issue. It is likely that administering low-molecular-weight heparin prophylactically to surgical patients at the same doses and schedules as those recommended for nondefect patients having similar procedures may be a valuable and safe procedure after correction of factor II deficiency by FFP or PCC infusion.
Hereditary factor V deficiency was initially termed parahemophilia because of its similarities with classical hemophilia.67 In most of the affected individuals, the phenotype is characterized by the concomitant deficiency of factor V activity and antigen (type I deficiency); however, approximately 25 percent of patients have normal antigen levels (type II deficiency), thus indicating the presence of a dysfunctional protein.68
Factor V is synthesized by the liver,69 and its plasma concentration is approximately 20 nM (7 mcg/mL).70–72 Factor V is a high-molecular-weight (Mr ~330,000), single-chain, large glycoprotein that consists of 2196 amino acids that bears significant, regional sequence homology to factor VIII. Analysis of the approximately 7-kb factor V complementary DNA showed that the protein is organized according to the following domain structure: A1-A2-B-A3-C1-C2. The A and C domains have approximately 40 percent homology with analogous domains in factor VIII.72,73 The large B domain shows no homology with the corresponding B domain of factor VIII. Factor V is converted to its activated form following several proteolytic cleavages by thrombin74 or factor Xa.75 These cleavages remove the B domain and yield factor Va, which consists of a heavy chain (A1-A2 domains) associated by Ca2+ with a light chain (A3-C1-C2 domains). The light chain contains the binding sites for membrane phospholipids, prothrombin, and activated protein C; both light and heavy chains probably are necessary for factor Xa binding. Assembly of factors Va and Xa on the phospholipid membrane of platelets in the presence of calcium ions forms the prothrombinase complex, which catalyzes the conversion of prothrombin to thrombin. The contribution of factor Xa in the absence of factor Va to overall thrombin generation is relatively minor. Importantly, incorporation of the cofactor into the macromolecular enzyme complex enhances prothrombin activation by several orders of magnitude.76
In addition to hepatocytes, the primary site of factor V secretion, approximately 20 percent of the protein in whole blood is localized in the α granules of platelets, where it is complexed with an extremely large protein, multimerin.77 Megakaryocytes do not synthesize factor V; rather, endocytosis of plasma-derived factor V accounts for the platelet factor V pool.78 Following endocytosis, factor V is modified intracellularly; these changes to platelet factor V appear to provide the cofactor with unique physical and functional characteristics, which render it more procoagulant compared with its plasma counterpart.79 Platelet degranulation and release of platelet factor V at the site of vascular injury is thought to be a critical contributor to the local factor V concentration. Furthermore, there is some evidence that, because platelet factor V is locally released in high concentrations, it is less susceptible to inhibition and may function normally in hemostasis. Factor Va is inactivated by activated protein C through limited proteolysis at Arg506, Arg306, and Arg679 in the presence of protein S, calcium ions, and either platelet or endothelial cell membrane phospholipids.80 Partial protection from this cleavage is provided by factor Xa when bound to factor Va on the surface of platelets.81
The factor V gene maps to chromosome 1q21–25.82 It is greater than 80 kb in length, and the coding sequence is divided into 25 exons, ranging in size from 72 to 2820 base pairs (bp), and 24 introns, varying between 0.4 kb and 11 kb.83
A total of 132 distinct mutations of the factor V gene have been identified, of which 64 are missense, 36 are insertions/deletions, 17 are nonsense, 15 are splice site mutations, and one is a deletion of the whole gene (see http://www.isth.org/?MutationsRareBleedin and Ref. 10). Most mutations cause truncations and are localized throughout the gene. Several mutations have interesting features. One, a Tyr1702Cys transition, was identified in eight unrelated families, of whom six were Italian. The frequency of this mutant allele in Italy is 0.002.84 Another mutation, an Ala221Val (New Brunswick) alteration, characterized in the homozygous state by activity and antigen levels of 29 and 39 percent of normal, respectively, displays decreased stability of the expressed protein and was the first genetic defect reported to be associated with type II deficiency.85 Additional mutations exhibit decreased secretion of the protein from producing cells.86,87 Remarkably, the Gln773ter and Arg1133ter mutations and a 4-bp deletion mutation, all present in exon 13 and predicted to result in partial truncation of the B-domain and complete truncation of the A3-, C1-, and C2-domains, cause no bleeding or only a mild bleeding tendency in affected patients having factor V antigen and activity levels 1 percent of normal.88–90
Factor V Leiden (Arg506Gln) is a highly prevalent (up to 5 percent in some populations) polymorphism in the factor V gene that decreases the efficiency of factor Va inactivation by activated protein C.91 Patients with factor V Leiden are at increased risk of unprovoked thrombosis, with homozygotes at greater risk than heterozygotes. The trans association of factor V Leiden and a mutation in factor V that causes factor V deficiency results in a prothrombotic state comparable to factor V Leiden homozygosity. This is sometimes termed “pseudohomozygous” activated protein C resistance and does not cause bleeding despite low factor V antigen levels.92 Among several polymorphisms detected in the factor V gene, His1299Arg in exon 13 is particularly interesting because it is associated with a reduced plasma factor V level and mild activated protein C resistance.93 His1299Arg co-segregates with several other polymorphisms encoding several amino acid changes, together named R2 haplotype.94 In two heterozygotes for factor V Arg506Gln mutation who presented with venous thrombosis, reduced factor V activity resulting from the His1299Arg polymorphism harbored by the non-Leiden chromosome, imparted a pseudohomozygous phenotype for activated protein C resistance.95 Additional polymorphisms or mutations in the factor V gene have been observed to increase the risk of venous thrombosis.96
In addition, there are at least two examples in which platelet factor V is reduced. In the Quebec platelet disorder, initially described as an autosomal dominant disorder with severe bleeding manifestations, platelet factor V levels are reduced because of enhanced proteolysis resulting from overexpression of urokinase-type plasminogen activator,97 as they are in factor V New York.98
Factor V deficiency is inherited as an autosomal recessive trait. Heterozygotes, whose plasma factor V activity ranges between 25 and 60 percent of normal, usually are asymptomatic, although an American registry recorded mild bleeding in 50 percent of the cases.99 According to a recent classification by the SSC of the ISTH, factor V deficiency may be classified as severe, moderate, and mild when factor V levels are undetectable, less than 10 percent, and 10 percent or greater, respectively.24
Common manifestations include ecchymoses, epistaxis, gingival bleeding, hemorrhage following minor lacerations, and menorrhagia.99–101 Severe deficiency typically presents at birth or in early childhood, but depending on factor levels, some patients remain asymptomatic. Bleeding from other sites is less common, but instances of hemarthroses unrelated to trauma and intracerebral hemorrhage have been reported.100 Trauma, dental extractions, and surgery confer a high risk of excessive bleeding.
PPH occurs in more than 50 percent of pregnancies in women with factor V deficiency,102,103 especially those with low factor V activity levels. Venous and arterial thromboses have been described in patients with factor V levels ranging between 2 and 14 percent of normal.104 Factor V deficiency deprives activated protein C of one of its essential substrates, thereby downregulating the inhibitory function of the protein C system.
Factor V is indispensable for life, as was demonstrated by experimental knockout mice lacking the factor V gene, which die either in utero at embryonic day 9 or 10 because of defects in yolk-sac vasculature and somite formation; the remaining half develop to term but die of massive hemorrhage within hours of birth.105 The expression of a minimal factor V activity because of the introduction of a liver-specific transgene, below the sensitivity threshold of the detection assay (<0.1 percent), leads to the survival of mice.106
Patients with epistaxis and gingival bleeding may respond to tranexamic acid (1 g four times daily), and local hemostatic measures may suffice for minor lacerations. Menorrhagia can also be managed directly using oral contraceptives, progestin-containing intrauterine devices, endometrial ablation, or hysterectomy. If these measures fail, severe spontaneous bleeding occurs, or surgery is performed, treatment option is limited to FFP replacement as no specific factor V concentrate is yet available on the market and factor V is not present in cryoprecipitate or PCCs. Development of a functional factor V inhibitor after receiving plasma transfusions was reported in only two patients with hereditary deficiency; the inhibitor disappeared in one patient, but a low titer of the inhibitor persisted in the other patient.107,108
A new factor V concentrate has been developed for clinical use in patients deficient in factor V, and preclinical studies are currently being performed for the orphan drug designation application to the European Medicines Agency (EMA) and the Food and Drug Administration (FDA) so as to make it available on the market as soon as possible.
Combined deficiency of factors V and VIII (F5F8D) is completely separate from factor V deficiency and factor VIII deficiency. The latter two are transmitted with different patterns of inheritance (autosomal recessive for factor V, X-linked for factor VIII) and involve proteins encoded by two different genes (F5 gene and F8 gene). F5F8D was first described in 1954109; however, the molecular mechanism of the association of the combined factor deficiency was not understood until the late 1990s,110,111 when null mutations in the endoplasmic reticulum–Golgi intermediate compartment (ERGIC)-53 gene, now called LMAN1, were determined to be causative. In 2003, a second locus associated with the deficiency in approximately 15 percent of affected families with no mutation in LMAN1 was identified26: the MCFD2 gene encoding for a cofactor for LMAN1. Even if a debate were carried out on the possible existence of other loci involved in the intracellular transport of factors V and VIII and associated with the disease, until now, previous biochemical studies failed to identify additional components of the LMAN1–MCFD2 receptor complex,112 supporting the idea that F5F8D might be limited to the LMAN1 and MCFD2 genes.113 The disorder has been detected in many populations, but a relatively high frequency occurs among Tunisian and Middle Eastern Jews residing in Israel114 and among Iranians.115
Factors V and VIII are essential coagulation factors that circulate in plasma as precursors. Upon limited proteolysis by thrombin or factor Xa and in concert with negatively charged phospholipid surfaces, factors VIIIa and Va exhibit profound cofactor activities for activation of factor X by factor IXa and for activation of prothrombin by factor Xa, respectively. Inactivation of factors Va and VIIIa is accomplished by activated protein C in the presence of protein S and phospholipids through several proteolytic cleavages at distinct sites. Factor V and factor VIII have similar domain organizations with partial homology (see “Factor V Deficiency” above).
The pathogenesis of combined deficiency of factors V and VIII puzzled investigators for more than 40 years. The enigma was resolved by the finding that the disease stems from the deficiency of either one of two interacting proteins, LMAN1 and MCFD2, which play a role in the intracellular transport of factors V and VIII. LMAN1 is a 53-kDa type 1 transmembrane nonglycosylated protein with homology to leguminous lectin proteins.116 It displays different oligomerization states—monomer, dimer, and hexamer—which have been implicated in its exit/retention within the endoplasmic reticulum (ER), and is thought to bind correctly folded glycosylated cargo proteins, including factors V and VIII in the ER, recruiting the cargo for package into coat protein complex II (COPII)–coated vesicles and to transport them first to the ERGIC and then to the Golgi. MCFD2 is a small (146 residues) soluble protein of 16 kDa with a signal sequence mediating translocation into the ER and two EF-hand motifs that may bind Ca2+ ions in the C-terminal region.117 MCFD2 forms a Ca2+-dependent 1:1 stoichiometric complex with LMAN1, which works as a cargo receptor for efficient ER–Golgi transfer of coagulation factors V and VIII during their secretion. Although several proteins have been identified as cargo of LMAN1 (factor V, factor VIII, cathepsin C, cathepsin Z, nicastrin, and α1-antitrypsin),118–121 MCFD2 is only known to be required for transport of the blood coagulation factors, suggesting a possible role for MCFD2 as a specific recruitment factor for this subset of LMAN1 cargo proteins.122 The three-dimensional structure of the complex between MCFD2 and the carbohydrate recognition domain (CRD) of LMAN1 was determined, and a model of functional coordination between the two proteins was proposed: MCFD2 is converted into the active form upon complex formation with LMAN1, thereby becoming able to capture polypeptide segments of factors V and VIII. The coagulation factors bind the LMAN1 oligomer in the ER but are released upon arrival in the acidic post-ER compartments because the sugar binding of ERGIC-53 is pH-dependent.123
Homozygosity mapping and positional cloning in nine unrelated Jewish families demonstrated that LMAN1, composed of 13 exons, localizes on the long arm of chromosome 18.110,111,124 Using a similar approach in other families with the combined factors V and VIII deficiency, the short MCFD2, made up of four exons, was localized on the short arm of chromosome 2.26 Thirty-four mutations identified in LMAN1 predicted either a truncated protein product or no protein at all, being more than 90 percent deletion/insertion, null, or splicing mutations. In contrast, of the 22 mutations identified in the MCFD2, 11 are missense and 11 are null mutations. Missense mutations are located at the EF-2 domains, giving rise to defective binding to LMAN1.125 A distinct founder haplotype was found in patients belonging to six unrelated families of Tunisian-Jewish origin bearing a donor splice-site mutation in intron 9 of LMAN1.110,125 All six families originated from an ancient Jewish community that has resided on the island of Djerba for more than 2 millennia. A survey of this community, which presently lives in Israel, disclosed that the mutation is prevalent at an allele frequency of 0.0107.126 Another founder effect for a G insertion in exon 1 of LMAN1 was observed in eight unrelated Jewish families of Middle Eastern origin.110,125 A Met to Thr mutation in LMAN1 has been detected in several unrelated Italian families, implying another founder effect.125
Symptoms of combined factors V and VIII deficiency are generally mild. Comparison of relatively large cohorts of patients with such disorder in India, Iran, and Israel indicates that bleeding from trauma/surgery is the most frequently reported clinical manifestation.114,115,127,128 This observation likely reflects the fact that often the combined factors V and VIII deficiency is brought to the attention of physicians following excessive bleeding during and after trauma, surgery, and labor. Homozygous patients exhibit spontaneous and posttraumatic bleeding. Menorrhagia, epistaxis, easy bruising, hemarthrosis, and gingival hemorrhage are commonly observed and are unrelated to trauma in approximately 20 percent of cases.115,129 Hematuria, gastrointestinal tract bleeding, and spontaneous central nervous system bleeding are less common.115 There are insufficient data on the incidence of bleeding during pregnancy and PPH in women with combined factor V and VIII deficiency.
Heterozygotes exhibit slight but significantly reduced mean levels of factors V and VIII.114 In a literature survey of 161 heterozygotes, 22 reported having significant bleeding manifestations.130 However, no correlation between the factor V or factor VIII levels and bleeding tendency was noted.25
LMAN1 gene knockout mice duplicate the F5F8-deficient phenotype in humans, albeit with a milder presentation, resulting from a lesser reduction in plasma levels of factors V and VIII.131 The partial perinatal lethality observed in LMAN1-deficient mice on some genetic backgrounds was unexpected and has been explained as the result of a further drop in the level of LMAN1-dependent protein(s) below a critical threshold or as a result of a strain-specific difference in another cargo receptor whose function overlaps with LMAN1.
Because of the mild-to-moderate bleeding symptoms, treatment is on demand, depending on the severity of bleeding. According to a recent result from the EN-RBD project, however, the level of both factors to ensure the absence of bleeding symptoms should be greater than 40 percent.25 The recommended therapy includes FFP, which provides factor V, and factor VIII concentrate, which compensates for the shorter half-life of plasma factor VIII. An antifibrinolytic agent such as tranexamic acid or ε-aminocaproic acid can be helpful in patients exhibiting menorrhagia, epistaxis, or gingival bleeding. DDAVP (1-deamino-8-D-arginine vasopressin, desmopressin) could be administered for less severe bleeding. Patients with severe bleeding episodes or patients undergoing surgical procedures, including dental extractions, should receive FFP as replacement for factor V and cryoprecipitate or factor VIII concentrate as a source of factor VIII. DDAVP can be used to increase factor VIII level, but this treatment sometimes fails.132
Factor VII was first identified as serum prothrombin conversion accelerator or proconvertin and its hereditary deficiency described by Alexander and colleagues in 1951.133 Among the rare clotting factor deficiencies, the relative frequency of factor VII deficiency is high (see Table 14–1).99,100 A presumptive diagnosis can be easily made because, except for very rare cases of factor X deficiency only affecting the TF pathway of coagulation (see “Laboratory Diagnosis”), factor VII deficiency is the only coagulation disorder that produces a prolonged PT and a normal aPTT.
Human factor VII is a single-chain glycoprotein (Mr ~50,000) that is secreted from the liver parenchymal cells as a zymogen. The mature protein consists of 406 amino acids organized in three main domains: a Gla domain at the N-terminus containing 10 Gla residues, an epidermal growth factor (EGF) domain in the center, and a serine protease domain at the C-terminus.134 Factor VII zymogen circulates in blood at an extremely low concentration (~500 ng/mL)135 and has the shortest half-life of all coagulation factors (4 to 6 hours; see Table 14–3). Factor VII is converted to the activated form, factor VIIa, by cleavage of an Arg152-Ile153 bond, resulting in a two-chain molecule held together by a disulfide bond. This conversion is mediated by factor Xa,136 factor IXa,137 factor XIIa,138 thrombin,136 and factor VIIa in the presence of TF, in an autoactivation reaction.139 Binding of factor VII to TF strikingly enhances these reactions.140–144
The initial generation of thrombin that heralds blood coagulation occurs when blood is exposed to TF present in the subendothelium in tissues or on the surface of stimulated monocytes or microparticles. The exposed TF forms a complex with circulating factor VIIa and supports the initiation of coagulation by converting factors IX and X into their active forms (factor IXa and factor Xa).145,146 Hence, the TF–factor VIIa complex has two roles: to increase the conversion of factor VII to factor VIIa and to increase the proteolytic activity of factor VIIa toward its substrates, factors IX and X. Factors IXa and Xa may remain associated with cells that display the TF or disseminate in the blood and bind to the surface of activated platelets, which form the initial platelet plug.147
The factor VII gene (F7) spans approximately 12.8 kb148 and is located on chromosome 13q34,30,149 2.8 kb upstream from the factor X gene (F10).150 The gene contains a prepro leader sequence and eight exons that encode the mature protein.
More than 240 mutations have been reported (see http://www.isth.org/?MutationsRareBleedin and Ref. 12). The mutations are distributed throughout the gene, and most are missense mutations (62.2 percent); other type of mutations are equally present (ranging from approximately 6.2 percent of mutations in 3′-5′ untranslated region [UTR] to 12.3 percent of deletions/insertions [del/ins]). Most mutations causing factor VII deficiency have been observed in individual patients. However, one missense mutation (Ala244Val) was detected in 102 (84 percent) of 121 independent mutant alleles discerned in 88 unrelated patients in Israel.151 Most subjects were of Iranian and Moroccan-Jewish origin and shared an identical haplotype, consistent with a founder effect. In the general Iranian-Jewish and Moroccan-Jewish populations, the prevalence values of the Ala244Val allele are 0.023 and 0.025, respectively.152 Several additional clusters of patients with a specific mutation were reported: (1) Ala294Val, with or without a deletion of nt C, at position 11128, prevails in patients from Poland and Germany but has also been identified in other Europeans153,154; (2) 12 unrelated families from Norway who carry Gln100Arg155; (3) IVS75G>A, which was detected in six unrelated patients from the Lazio region in Italy, all of whom bear the same haplotype, suggesting a founder effect156; and (4) Gly331Ser, which was identified in 10 Italian and four German patients on one haplotype.157 The widely distributed and common Arg304Gln mutation probably is a recurrent mutation.158
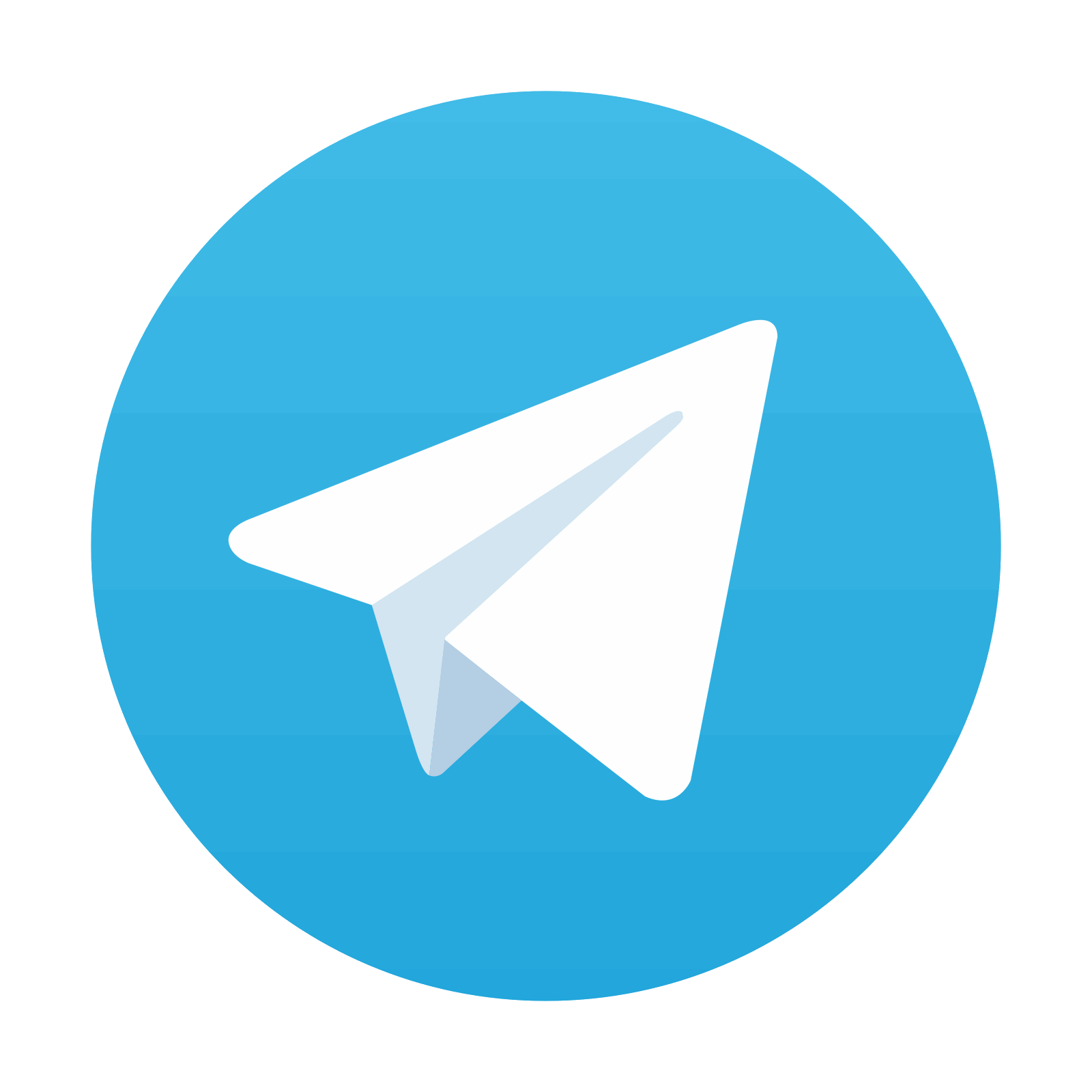
Stay updated, free articles. Join our Telegram channel

Full access? Get Clinical Tree
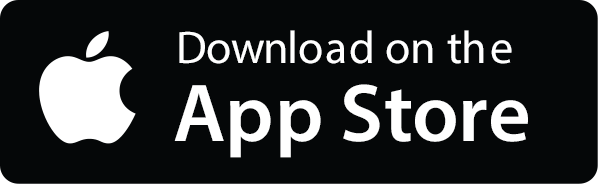
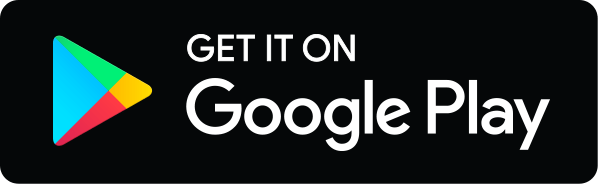