Fig. 3.1
Inflammation in lung cancer development. Carcinogens in tobacco smoke induce inflammatory damage directly on normal epithelium. Additionally, exposure to tobacco smoke leads to induction and recruitment of proinflammatory responses and cells that also contribute to tumor-initiating inflammation as well as promote lung tumor development. Invasive and overt lung tumors themselves promote an inflammatory milieu which in turn facilitates lung tumor progression and dissemination. The photomicrographs represent the histopathological sequence of development of lung squamous cell carcinomas (SCCs)
Inflammatory Mediators and Modulators
Cytokines and their Receptors
Various cytokines, including chemokines, and their associated receptors play important roles in lung cancer-associated inflammatory response. Interleukins, such as IL-1β, IL-2, IL-4, IL-6, IL-8, IL-10, IL-12, IL-17, cytokines such as tumor necrosis factor alpha (TNFα), interferon gamma (IFNγ), stromal cell derived factor 1 (SDF1), chemokine C-X-C motif ligand 10 (CXCL10), chemokine C-C motif ligand 2 (CCL2), and cytokine receptors such as chemokine C-X-C motif receptors 2 and 4 (CXCR2 and CXCR4) have been shown to be linked to various stages of lung cancer development [12–14]. Importantly, the aforementioned inflammatory mediators have been shown to be produced by both lung cancer cells and the microenvironment comprising innate and adaptive immune cells. Notably, mounting evidence suggests that the profile of activated cytokines, as well as recruited leukocytes, lymphocytes, and macrophages vary based on the stage of lung cancer development and whether preneoplastic or neoplastic lung lesions have already been formed [2, 4]. Neoplastic microenvironments favor polarized chronic protumorigenic inflammatory states that are of the T helper 2 (Th2) type inflammatory response [2]. This type of inflammatory response involves suppression of cytotoxic CD8 + T-lymphocytes, production of IL-4 by innate immune cells, and of IL-8 and IL-1β by tumor cells themselves further perpetuating the inflammatory lung tumor microenvironment [2, 4]. In contrast, Th1 is the predominant inflammatory response before development of lung lesions (e.g., in COPD) that promotes a cytotoxic immune response comprising increased production of IL-12 rather than IL-4 [2, 14].
IL-8 and IL-6 have been shown to be produced by both resident macrophages and autonomously by lung epithelial cells as well as by tumors in the tumorigenic proinflammatory response [12]. Importantly, Il-8 was shown to be induced by the K-ras oncogene in mice to promote tumor-associated inflammation and neovascularization [33]. IL-8 was also shown to induce expression of the cytokine receptor CXCR1 in lung cancer cells [34]. Interestingly, IL-8 is a member of an 80-gene expression signature that was found to effectively identify lung cancer patients from smokers without lung cancer following global gene expression analysis of airway brushings from smoker patients with suspicion of the malignancy [35]. In the same report, IL-8 RNA and protein was elevated in histologically normal proximal bronchi from patients with lung cancer compared to phenotypically normal smokers [35]. IL-6 was shown to be produced by lung tumor cells and subsequently activate signal transducer and activator of transcription 3 (STAT3) [36, 37]. Moreover, Il-6 was shown to be upregulated by NTHi–induced COPD-like airway inflammation and lung tumorigenesis in K-ras(G12D) mutant mice and genetic ablation of Il-6 decreased lung tumor development [38].
IL-1β was also shown to be upregulated and released by lung tumor cells during the proinflammatory response [12]. This cytokine was shown to induce expression of gelatinase (matrix-metallopeptidase 9/MMP9) in human lung tumor cells [39] as well as cause activation and nuclear translocation of the proinflammatory NF-κB [40]. Notably, IL-1β immunohistochemical expression was found to be upregulated in the respiratory tract of smoking patients, compared with the normal ciliated epithelium of nonsmoking patients, concomitant with activation of NF-κB [41]. Moreover, anti-IL-1β or -IL-6 targeted therapies in cigarette smoke-exposed mice significantly reduced epithelial hyperplasia and the development of squamous metaplasia [41]. IL-1β may represent an attractive target for lung cancer therapy as inhibition of Il-1β was found to be sufficient for concomitant blockade of inflammation and tumor growth in mice [42]. Similarly, inhibition of TNFα inhibited inflammation and lung tumor growth [41]. IL-4 was shown to promote the pro-tumorigenic function of TAMs through activation of cathepsin proteases [43]. IL-4 was also demonstrated to function as an autocrine growth factor in lung tumor cells and prevent induction of apoptosis through upregulation of various antiapoptotic proteins [44].
The IL-17 cytokine is produced by T helper 17 cells (Th17 inflammatory response subtype) and has been found to augment tumor growth and cell proliferation in lung cancer and represent an attractive therapeutic target in lung malignancies [45]. IL-17 was shown to promote tumor growth by activation of IL-6/STAT3 pathway [46]. Lung tumor-associated macrophages express high levels of interleukin 17 receptor (IL-17R), and lung tumor cells were shown to express high levels of IL-17 [45]. Importantly, it was shown that IL-17 along with prostaglandin E2 (PGE2) are involved in the formation of a macrophage 2 (M2)-dominant lung tumor promoting microenvironment [47]. IL-17 binding to IL-17R was shown to activate NF-κB and mitogen-activated protein kinase (MAPK) signaling in pulmonary inflammation [48]. IL-17 producing cells were found in malignant pleural effusions [49] and IL-17 was shown to promote lymphangiogenesis and production of vascular endothelial growth factor C (VEGFC) in lung tumor cells [12]. It is worthwhile to mention that IL-17 and T helper 17 cells were shown to play a role in regulating neutrophilic and macrophage inflammation in COPD [50]. It is unclear if there are similarities in the role of IL-17 in the pathogenesis of COPD and lung cancer since the pro-inflammatory response in the former is Th1 predominant and cytotoxic, whereas the response in the latter is Th2 predominant and pro-tumorigenic [14]. Further studies are needed to elucidate the role of IL-17 in inflammatory conditions that predispose to lung cancer (e.g., COPD) relative to its role in lung cancer progression.
Several cytokines have been shown to be involved in the anti-lung tumor cytotoxic inflammatory Th1 response including IFN-γ, IL-2, IL-10, and IL-12 [45]. IL-2 immunotherapy was shown to exhibit anti-lung tumor activity [51]. IL-10 (CXCL10) is induced by IFN-γ in CD8 + cytotoxic T-lymphocytes in the pathogenesis of COPD [52]. Moreover, lung cancer patients with high percentage of stromal CD8 + /IL-10 + cells exhibit longer overall survival compared to patients with reduced infiltrates of these IL-10 generating cells, and IL-10 positive cells are more evident in lung cancer patients with early (stage-I) disease [10]. Similarly, patients with high lung tumor expression of IL-12 receptor exhibited favorable outcome and longer time to recurrence [53]. Moreover, IL-12, like IL-10, was shown to induce matrix-degrading enzymes, such as MMP12, during pulmonary inflammation and COPD-associated emphysema [54].
Various cytokine receptors have been shown to be involved in the pathogenesis of lung cancer. CXCR4 was shown to activate migration of small-cell lung cancer cells (SCLC) and inhibition of this receptor was shown to sensitize lung tumor cells to chemotherapy [12]. Inhibition of Cxcr2, a receptor for Il-8, was shown to cause lung tumor regression in mice [55]. Importantly, Cxcr2 ligands were found to be upregulated in preneoplastic alveolar lesions in a mutant K-ras lung tumor mouse model [Kras(LA1) mice] [56]. Treatment of Kras(LA1) mice with a Cxcr2-neutralizing antibody inhibited the progression of premalignant alveolar lesions and induced apoptosis of vascular endothelial cells within alveolar lesions suggesting that CXCR2 may contribute to the expansion of early alveolar preneoplastic lesions induced by oncogenic KRAS [56]. Interestingly, CXCR2 was shown to perpetuate epithelial mesenchymal transition (EMT)-mediated lung tumor progression since CXCR2 blockade abrogated SNAIL-mediated lung tumorigenesis [57] pointing to the role of CXCR2 in intersection of EMT and inflammation in lung tumor progression. More recently, CXCR2 was shown to be associated with smoking and poor prognosis in lung adenocarcinoma [58] and studies using CXCR2 small molecular weight inhibitor SB225002 highlighted the importance of this cytokine receptor in lung cancer cell growth, migration, and invasion [58]. Other proinflammatory receptors have been shown to be involved in inflammation-associated lung tumorigenesis such as toll-like receptors (TLRs). The induction of Th1-like and cytotoxic immunity by TLR signaling could lead to tumor cell death, resulting in tumor regression or arrest [59]. However, several studies have demonstrated that the activation of specific TLRs, such as TLR2, TLR4, and TLR9, may promote lung cancer progression [59].
Proteinases
Various proteinases, including elastases and matrix metallopeptidases (MMPs), have been implicated in the proinflammatory response associated with lung cancer development and progression. Neutrophil elastase (NE) is a major player in inflammatory pathological conditions in the lung such as COPD and emphysema [14]. NE was shown to degrade elastin in the lung and contributes to cigarette smoke-induced emphysema in mice [60]. Houghton and colleagues demonstrated that NE promoted lung carcinogenesis in the mutant K-rasG12D (LSL-K-ras) mouse model for lung adenocarcinoma [61]. The tumorigenic effects of NE were attributed to activation of the phosphatidyl inositol 3-kinase (PI3K) pathway activation through degradation of insulin receptor substrate 1 (IRS1) to induce lung tumor cell proliferation [61].
Several MMPs have been shown to be implicated in inflammation-associated lung cancer pathogenesis, namely MMP1 (collagenase), MMP9 (gelatinase B), and MMP12 (macrophage elastase). Mmp1 was shown to promote invasion and metastatic progression of mouse lung cancer [62] and to be involved in matrix degradation and cleavage and release of Egf-like ligands for lung tumor bone metastasis [63]. It is worthwhile to mention that a transgenic mouse model with expression of human MMP1 transgene in the lungs showed morphological and physiological abnormalities, similarly observed in emphysema in humans [64]. MMP9 expression in sputum was found to positively correlate with airway obstruction in COPD [65] and its expression in lung adenocarcinomas is correlated with that of immune response genes [66]. MMP12 encodes for an interesting proteinase that is expressed in macrophages and exhibits paradoxical roles in chronic lung inflammation and in inflammation-associated lung cancer pathogenesis [14]. MMP12 is induced by IL-10 through CXCR4 in a Th1 inflammatory response to cause matrix destruction in the lung and subsequent inflammation and emphysema [67]. Although MMP12 protein was found to be over-expressed in lung tumors and surrounding stroma evidenced by immunohistochemical analysis, growth of tail-vein-injected murine lung cancer cells was increased in Mmp12 knockout mice [68]. In addition, mice with knockout of Mmp12 develop significantly more lewis lung carcinoma metastases compared to their wild type littermates [69]. On the other hand, a study by Qu and colleagues demonstrated that a genetically modified mouse model of Mmp12 over-expression lead to increased inflammatory cell infiltration, epithelial cell proliferation and subsequent emphysema, and development of bronchioalveolar lesions suggesting that Mmp12 may promote lung carcinogenesis [70]. The basis for these disparate findings by the different studies is unclear; however, it is plausible to suggest that MMP12 may play important proinflammatory and protumorigenic roles in early stages of lung cancer progression and/or development of low-grade lesions (e.g., bronchioalveolar carcinoma) as well as anti-tumorigenic roles in later stages of lung carcinogenesis (e.g., metastases).
NF-κB
The transcription factor, NF-κB, is considered to be a lynchpin in inflammation-associated cancer with major proinflammatory and protumorigenic roles [71]. Mounting evidence suggests that chronic inflammation contributes to lung cancer development and progression through activation of NF-κB [15]. In NSCLC cell lines, it has been demonstrated that tobacco components stimulate NF-κB dependent survival [72] and the COX2 inhibitor celecoxib suppresses NF-κB activation induced by several carcinogens [73]. There are limited comprehensive studies on the expression patterns of NF-κB in lung tumors and lung preneoplastic lesions. Increased nuclear NF-κB expression was reported in a limited number of moderate and severe squamous dysplasias obtained from smokers without cancer relative to normal bronchial epithelium specimens [74]. In a study utilizing a relatively large series of primary lung cancer and preneoplastic histological tissue specimens, nuclear NF-κB (p65) immunohistochemical expression was prevalent in lung tumors and significantly higher in SCLCs compared to non-small cell lung cancers (NSCLCs) [75]. In adenocarcinomas, nuclear NF-κB expression level was significantly higher in advanced (III–IV) than earlier (I–II) stages [75]. Moreover, a relatively high level of nuclear NF-κB was detected in normal and mildly abnormal epithelium, and a progression with increasing histology severity was detected in preneoplastic lesions [75]. In addition, immunohistochemical expression of interleukin-1 receptor–associated kinase (IRAK-1), a kinase that relays signaling from IL-1R and TLRs to NF-κB activation was also reported to be elevated in squamous dysplasias compared to normal bronchial epithelium and the combination of its expression with that of NF-κB was a significant predictor of poor clinical outcome in lung cancer patients [76].
It has been suggested that NF-kB exerts its oncogenic effects in both the tumor and the microenvironment and promotes the survival of premalignant epithelial cells [77]. NF-κB acts in a cell type-specific manner: activating survival genes within cancer cells and inflammation-promoting genes in components of the tumor microenvironment [78]. NF-kB has been shown to suppress apoptosis and induce expression of proto-oncogenes such as MYC and CCND1 (cyclin D1), which directly stimulate cellular proliferation [15, 78]. In addition, NF-κB regulates the expression of various protumorigenic molecules, such as MMPs, COX2, inducible nitric oxide synthase (iNOS) for production of DNA damaging and mutagenic nitrite species as well as chemokines and proinflammatory cytokines, all of which promote lung cancer progression [15, 78]. NF-κB is activated in mouse embryonic fibroblasts following loss of p53 and expression of oncogenic K-ras(G12D) which in turn was crucial for lung tumor development in mice [79]. This effect was attenuated by p53 restoration that resulted in inhibition of NF-κB. The same study also showed that NF-kB inhibition induced apoptosis in lung cancer cell lines deficient of the p53 tumor suppressor [79] suggesting that targeting this proinflammatory nuclear factor may represent an attractive strategy for lung cancer therapy . Nf-κB was shown to be activated by the chemokine receptor Ccr5 in vivo that was crucial for lung tumor progression, since mice with knockout of Ccr5 exhibited reduced urethane-induced lung tumor development and Nf-κb activation [80]. NF-κB also exerts cytoprotective roles and prevents apoptosis induction following DNA damage and accumulation of ROS in lung epithelial cells suggesting that NF-κB may aid in promotion of lung epithelial cell survival during chronic inflammation and elevated oxidative stress [81]. Importantly, aberrant NF-κB activation and/or expression are common to both inflammatory lung diseases (e.g., COPD and emphysema) and lung cancers and, thus, may contribute to development of lung cancer in patients with COPD and emphysema [14, 82]. Mice with knockout of Gprc5a, an orphan receptor that is preferentially expressed in normal fetal and adult mouse and human lung tissues, develop spontaneous and late onset lung adenocarcinomas at 12–18 months of age [31], which is augmented and enhanced by exposure to tobacco specific carcinogens [83]. Moreover, lung adenocarcinomas in Gprc5a knockout mice exhibited abundant TAMs, and lungs of the mice displayed significantly elevated levels of proinflammatory chemokines and cytokines compared to lungs of their wild type littermates [32]. Interestingly, loss of Gprc5a increased activation of Nf-κb in vivo following injection of mice with lipopolysaccharide (LPS) [32]. These reports suggest that the lung-specific tumor suppressor GPRC5A may suppress lung tumorigenesis through inhibition of the proinflammatory factor NF-κB. Interestingly, immunohistochemical expression of GPRC5A was found to be markedly decreased in normal bronchial epithelia from patients with COPD, or in epithelia from patients with COPD and lung cancer compared to bronchial epithelia from healthy smokers [84].
Efficient therapeutic strategies against NF-κB are still lacking [78]. In a study by Xue and colleagues, inhibition of Nf-κb activation by the proteasome inhibitor bortezomib or the small molecular weight inhibitor BAY-117082 caused lung tumor regression and prolonged survival in mice with mutant K-ras and targeted p53 [85]. However, lung adenocarcinomas subsequently developed acquired resistance to both treatment strategies against Nf-κb [85]. It is important to note that NF-κB is activated by inhibitor of kappa B (IκB) kinases (IKKs) through alleviation of inhibitory effects of IκB [71, 78]. Given that IKK is a kinase complex with several catalytical subunits [86], it may represent an additional and attractive target for inhibition of the NF-κB pathway in lung cancer prevention and therapy [86].
COX2
Cyclooxygenase 2 catalyzes the synthesis of prostaglandin endoperoxidase H2 (PGH2) from the membrane phospholipid arachidonic acid [7, 87]. PGH2 is then converted to potent inflammatory and growth-promoting molecules named prostaglandins (e.g., prostaglandin E2/PGE2) by the action of tissue specific isomerases [7, 87]. Various studies have demonstrated the involvement of the eicosanoid pathway and COX2 in the pathogenesis of lung cancer [15]. COX2 is an intermediate early response gene induced and activated by growth factors, oncogenes, carcinogens, and tumor promoters, such as phorbol esters and exerts pro-inflammatory effects in a PGE2-dependent manner, which contributes to carcinogenesis and tumor progression [7, 87]. Cigarette smoke exposure can induce COX2 expression and activity, increase PGE2 release, and lead to an imbalance in PGI2 and thromboxane A (TxA2) production in favor of the latter [88]. COX2 has been shown to be over-expressed in lung adenocarcinomas and squamous cell carcinomas (SCCs) [89] and COX2 immunohistochemical expression was demonstrated to be highly expressed in bronchial squamous dysplasias, especially those exhibiting high-grade histology (severe dysplasia and carcinoma in situ) [90]. Expression of this cyclooxygenase has been shown to be associated with poor outcome in lung cancer patients [91]. Multiple lines of evidence suggest that targeting COX2 may be an effective strategy for lung cancer prevention and therapy as well as for the clinical management of inflammatory pulmonary conditions such as COPD [13, 14]. As mentioned before, epidemiological studies revealed that long-term usage of non-steroidal anti-inflammatory drugs, which target cyclooxygenases , reduces cancer risk [13]. Celecoxib inhibits survival and induce apoptosis of immortalized and premalignant lung epithelial cells [92]. Furthermore, Kim and colleagues showed that the COX2-specific inhibitor celecoxib decreased proliferation and KI67 expression in normal bronchial epithelia of phenotypically healthy smokers [20] .
NRF2
The transcription factor NRF2 is a master regulator of intracellular homeostasis and oxidative stress [93]. Basally, NRF2 is kept inactive through binding to its inhibitor Kelch-like ECH-associated protein 1 (KEAP1) that causes NRF2 degradation [93]. The NRF2 and KEAP1 pathway has been extensively studied in various pathological conditions and cancer and has garnered tremendous interest owing to the important roles this pathway plays. Upon activation and translocation to the nucleus, NRF2 transactivates the expression of various genes with antioxidant response elements (AREs) that can reduce oxidative and genotoxic stress induced by agents such as cigarette smoke or proinflammatory cells such as macrophages and neutrophils [94]. Experiments in mice, in particular using mice with knockout of both alleles of the Nrf2 gene, revealed cytoprotective and tumor suppressor roles for this transcription factor [93–96]. Nrf2 knockout mice exhibit increased epithelial cell apoptosis and emphysema compared to their wild type littermates following exposure to tobacco carcinogens [95]. Satoh and colleagues demonstrated that Nrf2-deficient mice exhibit a higher number of lung metastatic nodules, increased numbers of inflammatory cells, including myeloid-derived suppressor cells (MDSCs), a potent population of immunosuppressive cells that attenuate CD8 + cytotoxic T-lymphocytes [2], and elevated ROS levels compared to wild type mice [96]. These findings point to the potential use of activators of NRF2 function (e.g., oltipraz, [97]) for the prevention and therapy of lung cancer. However, recent reports have alluded to an oncogenic role for this transcription factor [93]. DeNicola and colleagues reported the activation of Nrf2 by various oncogenes, such as K-ras, B-raf, and myc, with concomitant cytoprotection, ROS decrease and tumorigenesis [98]. NRF2 was also shown to promote lung cancer cell proliferation and resistance to cisplatinum treatment [99]. Using 304 histological tissue specimens of human lung cancer, Solis and colleagues demonstrated abnormally high immunohistochemical expression of NRF2 in a large fraction of the tumors and low expression of its inhibitor, KEAP1, in adenocarcinomas [100]. Moreover, high or low expression of NRF2 and KEAP1, respectively, were significantly associated with poor prognosis [100]. Notably, the same study revealed that high or positive immunohistochemical expression of nuclear NRF2 was a significant predictor of poor response to adjuvant chemotherapy in lung SCCs [100]. Similar findings have been observed in other malignancies [93]. More recently, studies from the cancer genome atlas using next-generation sequencing (NGS) technology have confirmed and identified gain-of-function mutations in NRF2 and loss-of-function mutations in KEAP1 [101, 102]. The same studies also reported deletion of KEAP1 in a fraction of tumors and that loss of KEAP1 function or expression was the fourth most recurrent and significant event in lung adenocarcinomas [102]. These studies strongly point to an oncogenic role for the NRF2 transciption factor and a tumor suppressor function for its inhibitor KEAP1. Therefore, NRF2, as mentioned for proinflammatory responses above, seems to exhibit paradoxical functions and properties in tumor progression with both tumor suppressive and oncogenic roles. However, this begs the question whether NRF2 function is dependent on the stage of tumorigenesis [93] where its role in tumor initiation or cancer-free inflammatory lung diseases (e.g., COPD) is different from that in later stages of lung cancer. Indeed, it has recently been shown that NRF2 exhibits suppressive roles in tumor initiation and promoting roles during lung cancer progression [103]. Nrf2 deficient mice exhibited increased number of tumors compared to their wild type littermates following urethane exposure [103]. However, at later time points (16 weeks), the number of invasive adenocarcinomas was significantly higher in the wild type mice [103]. Importantly, whereas all Nrf2 wild type tumors exhibited K-ras mutations, tumors that developed in the knockout mice seldom exhibited mutations in this oncogene [103].
CTLA-4 and PD-1/PD-L1
As mentioned before, the types of proinflammatory responses in chronic inflammatory lung diseases or in very early phases of lung cancer development are different from that occurring during later stages of lung tumor progression [2]. Several lines of evidence have pointed to the suppression of cytotoxic T-cell function facilitating evasion of tumor cells from immunosurveillance and anti-tumor immunity [2]. Cytotoxic T-lymphocyte-associated protein 4 (CTLA-4), programmed cell death 1 (PD-1), and PD-1 ligand (PD-L1) have emerged as key mediators and suppressors of cytotoxic T-cell function [104]. The CTLA-4 receptor regulates the amplitude of early activation of naïve and memory T-cells [104] evidenced by the significant immune toxicity observed in mice with knockout of this receptor. Several preclinical studies suggest that targeting CTLA-4 function may be an efficacious strategy for lung cancer strategy [105]. In a phase II clinical trial, ipilimumab, an antibody against CTLA-4, with chemotherapy was effective against squamous NSCLC [106, 107]. The PD-1 receptor and its ligand PD-L1 is an important pathway that functions to limit the activity of T-cells during an acute inflammatory response to infection and to limit autoimmunity as well [104]. T-cells with higher expression of PD-1 exhibit impaired immune function and cytokine production and proliferative capacities. Several studies have demonstrated that PD-L1 is up-regulated in different tumor types inhibiting local anti-tumor T-cell responses by binding to the T-cell PD-1 receptor [108, 109]. Moreover, PD-1 was found to be expressed on the majority of tumor infiltrating CD8 + lymphocytes, which was lower compared to that on T-cells of healthy controls [110]. Several preclinical studies in mice have demonstrated the usefulness of blocking the PD-1/PD-L1 pathway for cancer immunotherapy [104]. Targeting the PD-1/PD-L1 pathway is a promising strategy for the clinical management of patients with advanced lung cancer. In a recent phase-I clinical trial, a human IgG4-blocking monoclonal antibody directed against PD-1 (BMS-936558, nivolumab), was tested in 296 patients with advanced solid tumors (76 NSCLC patients) who had prior chemotherapy and targeted therapy [111]. Durable responses were observed in 18 % of NSCLC patients [111]. Importantly, the same study demonstrated that tumor immunohistochemical expression of the receptors ligand PD-L1 was significantly associated with objective responses as 36 % of patients with PD-L1 positive tumors exhibited an objective response [111]. A separate phase I clinical trial also tested an antibody directed against the PD-1 ligand PD-L1 in 75 advanced NSCLC patients [112]. Despite toxic effects observed in a fraction of patients, complete or partial responses were observed in approximately 10 % of NSCLC patients [112]. These significant findings provide strong proof for clinical relevance of reactivating the dampened antitumor immune response as a therapeutic strategy for lung cancer.
Genetic Polymorphisms in Inflammation-Related Genes and Lung Cancer
Although the majority of diagnosed lung cancer cases are attributable to smoking, only approximately 15 % of lifetime smokers will continue to develop a lung malignancy suggesting the presence of host factors that render certain persons more susceptible and at higher risk for developing the disease [15, 24, 25]. The involvement of genetic changes in inflammation-related genes has further attested to the role of inflammation in the etiology of lung cancer [13]. Several genome-wide association studies (GWAS) and genetic linkage data have identified single nucleotide polymorphisms (SNPs) or common substitution variants in inflammation-related genes that are associated with altered lung cancer risk.
Engels and colleagues identified a strong association between lung cancer risk and an SNP in the IL-1β gene (IL-1β C3954T) [113] in a study that included over 1500 cases. Persons with this SNP exhibited a significantly increased risk for lung cancer and with a low false-positive probability [113]. The association of SNPs in IL-1β with elevated risk for lung cancer has also been demonstrated in Asian populations [12, 13]. A case-control study in a Japanese population found that lifetime smokers with the IL-1β rs1143634 SNP exhibited a 45 % higher risk for lung cancer [114]. Polymorphisms in several cytokines and inflammation-related genes that are associated with risk for lung cancer have been identified including those in IL-6, IL-8, COX2, and IL-10 and are well reviewed by Engels and colleagues [13]. In addition, a SNP in CTLA-4 has been shown to be indicative of poor prognosis in a Chinese population with advanced NSCLC [115] and Karabon and colleagues demonstrated that SNPs in CTLA-4 predict increased risk for lung cancer development in a Caucasian population [116]. Notably, studies from the International Lung Cancer Consortium have identified additional polymorphisms in genes with increased risk for lung cancer. Truong and colleagues identified that a nonsynonymous SNP in p53 binding protein 1 (TP53BP1) was significantly associated with an increased risk for lung cancer [18]. In a separate study by the consortium, previous associations identified in Caucasians [117, 118], namely SNPs in chromosome regions 15q25, 5p15, and 6p21 that predicted elevated risk for lung cancer, were confirmed in an independent Asian population [119].
Polymorphisms in genes have also been described in both lung cancer and predisposing chronic lung inflammatory conditions such as COPD. It is important to point out the significance of these findings and polymorphisms, since they may link the pathogenesis of COPD and/or emphysema with lung cancer [14] and may be the basis for explaining the differential susceptibility and risk COPD patients exhibit for developing lung cancer. SNPs in the nicotinic acetylcholine receptors CHRNA3 and CHRNA5, that reside in the 15q25 chromosome region mentioned above, have also been shown to be associated with increased risk for emphysema and airway obstruction besides that for lung cancer [120]. Polymorphisms in the MMP1 proteinase have been found to be associated with poor pulmonary function as well as an elevated risk for lung cancer [121]. However, it is noteworthy that the same study by Hu and colleagues revealed association with polymorphisms in the MMP2 and MMP9 proteinases with decreased risk for lung cancer, which warrant confirmation in additional studies.
Interestingly, a recent study by Huang and colleagues highlighted polymorphisms in the canonical NF-κB pathway that are strongly associated with increased risk for either COPD or lung cancer [122] using relatively large Asian populations (1511 COPD patients and 1677 normal lung function controls as well as 1559 lung cancer cases and 1679 cancer-free case controls). The study demonstrated that SNPs in NF-κB and its inhibitor IκB were strongly associated with increased risk for COPD and lung cancer, respectively [122]. Interestingly, the SNP in IκB was found to decrease IκB expression leading to increased activation of the proinflammatory NF-κB [122]. These important findings suggest that genetic factors leading to activation of the NF-kB pathway are common to the development of both lung cancer and chronic inflammatory lung diseases such as COPD.
Inflammatory Markers and Lung Cancer
Disease-driven cytokines have been shown to be prognostic in lung cancer and have been detected in lung tumors [12] and surrogate tissues such as the airway [35, 41, 123], blood [124], and even exhaled breath condensate [125]. As mentioned before, immune cell infiltrates around lung tumors have been shown to be associated with prognosis in lung cancer patients [8, 10]. Infiltrates of CD8 + cytotoxic lymphocytes or IL-10 producing cells have been shown to predict favorable prognosis in lung cancer patients [10], whereas tumor infiltrates containing macrophages, neutrophils, or mast cells predict poor survival in lung adenocarcinoma patients [8]. A cytokine expression signature measured in lung adenocarcinomas and in the surrounding tumor microenvironment was found to be predictive of prognosis in lung cancer patients [126].
As mentioned before, autoantibodies have been detected in sera of lung cancer patients [6]. Various proinflammatory cytokines have been detected in the blood and sera of lung cancer patients including IL-6, IL-8, IL-10, and TNF-α [12, 124, 127–130]. Macrophage colony stimulating factor (M-CSF) levels have been shown to be an independent prognostic factor in NSCLC [128]. In addition to cytokines, levels of serum amyloid A (SAA) have been detected in plasma of lung cancer patients and have been shown to be predictive of an elevated risk of lung cancer [131]. Sung and colleagues demonstrated that serum levels of both SAA1 and SAA2 were 14-fold higher in lung cancer patients relative to healthy controls [132]. Moreover, SAA levels in plasma were also shown to be predictive of lung cancer prognosis [133]. Levels of the proinflammatory C-reactive protein (CRP) have been shown to predict poor survival in early-stage and definitively treated NSCLC patients [134] and in advanced lung cancer [135]. Notably, in a case-control nested study, serum levels of CRP were predictive of increased lung cancer risk [136–138] even when CRP levels were measured well before lung cancer diagnosis. Similar findings were observed for serum levels of IL-6 and TNF [137]. Using patients and control subjects in the prospective Prostate, Lung, Colorectal, and Ovarian (PLCO) cancer screening trial, Pine and colleagues demonstrated that serum levels of IL-6 and IL-8 were both associated with increased risk of lung cancer with only the latter cytokine being strongly associated with elevated risk several years before diagnosis [124]. The same group also showed that combined serum levels of IL-8 and CRP was a robust biomarker for predicting lung cancer development [124]. These findings point to the importance of inflammation in the etiology of lung cancer that translates to the potential development of noninvasive biomarkers comprising inflammation-related markers for lung cancer detection. Future studies are warranted to confirm many of these significant findings and to determine their effectiveness of predicting risk of lung cancer in nonsmokers as many of these changes may be surrogates of tobacco consumption.
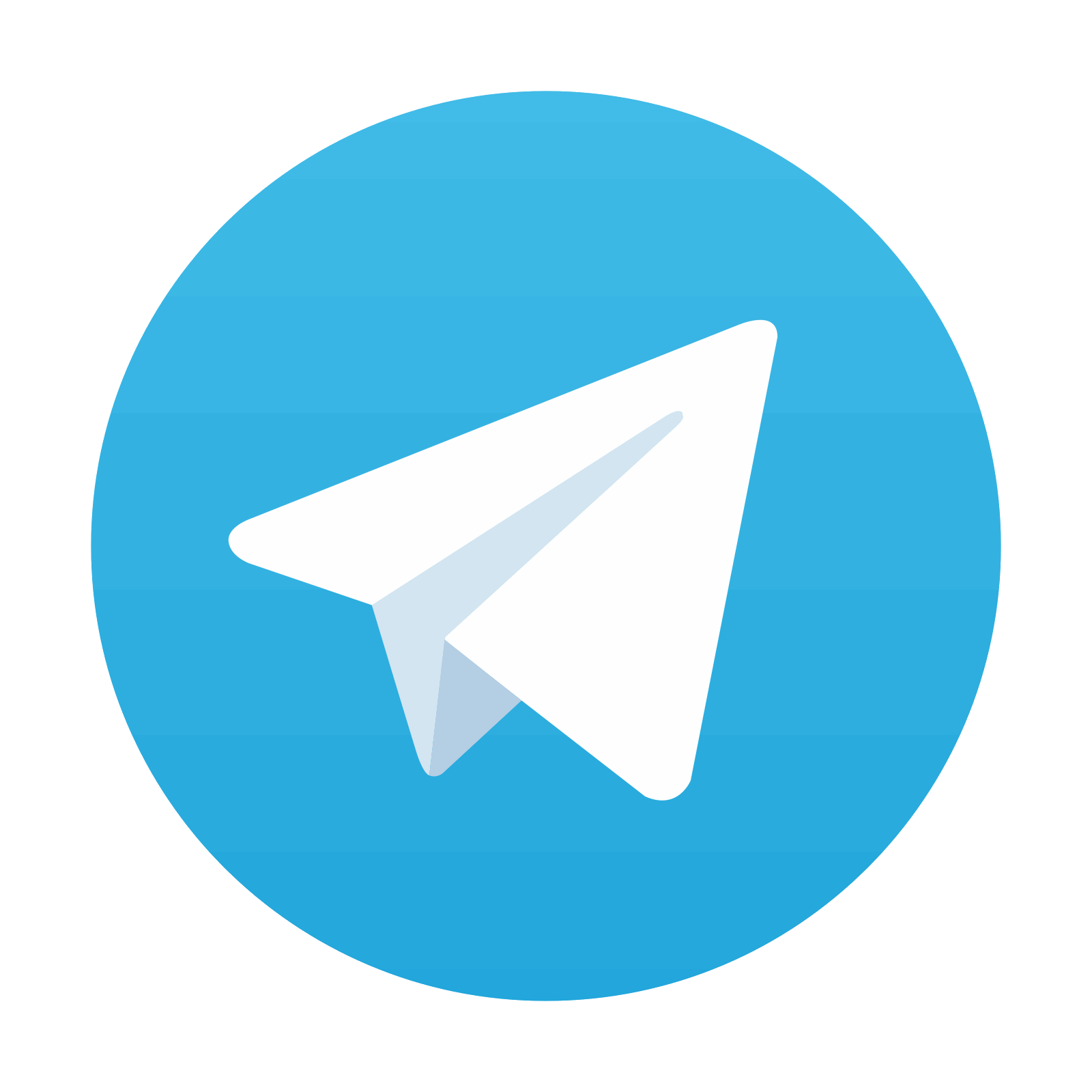
Stay updated, free articles. Join our Telegram channel

Full access? Get Clinical Tree
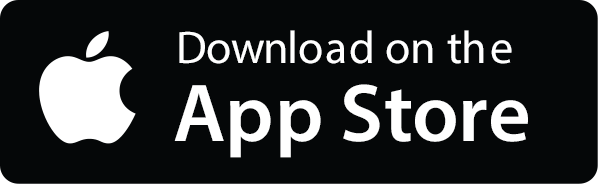
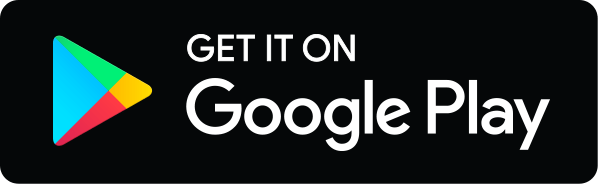