© Springer Science+Business Media New York 2015
Steven M. Dubinett (ed.)Inflammation and Lung Cancer10.1007/978-1-4939-2724-1_77. Inflammation and Lung Cancer: Addressing Inflammation with Immunotherapy
(1)
Division of Pulmonary and Critical Care Medicine, Department of Medicine, Jonsson Comprehensive Cancer Center, David Geffen School of Medicine at UCLA, VA Greater Los Angeles, 90073 Los Angeles, CA, USA
(2)
Division of Hematology/ Oncology, Department of Medicine, David Geffen School of Medicine at UCLA, 90095 Los Angeles, CA, USA
(3)
Departments of Medicine, Pathology and Molecular and Medical Pharmacology, Jonsson Comprehensive Cancer Center, David Geffen School of Medicine at UCLA, 90095 Los Angeles, CA, USA
(4)
Division of Thoracic Surgery, Department of Surgery, Jonsson Comprehensive Cancer Center, David Geffen School of Medicine at UCLA, 90095 Los Angeles, CA, USA
Abstract
New ground-breaking discoveries in cancer immunotherapeutics are now applicable for lung cancer therapy, exemplified by the recent FDA approval of nivolumab for advanced squamous cell carcinoma of the lung as a second line therapy, harnessing the immune system to fight lung cancer. This opens the door for lung cancer to enter the mainstream of immunotherapeutics along with other malignancies that have been traditionally viewed as immune unresponsive. The durable responses that result in continued separation of the survival curves suggest a new emerging paradigm that may add significantly to the benefit of existing conventional therapies. This chapter reviews immunotherapeutic approaches for lung cancer that have shown promise in early clinical trials and/or have advanced to late-phase development and FDA approval.
Keywords
Lung cancerImmunotherapyTherapeutic vaccineImmune suppressionT cell immune regulatory checkpointImmune checkpoint inhibitorsIntroduction
Lung cancer consists of tumor cells, stroma, blood vessels, immune infiltrates, and the extracellular matrix. Genetic alterations in oncogenes and tumor suppressor genes, or epigenetic changes that modulate tumor growth and invasion orchestrate the persistence of inflammatory infiltrates. Lung cancers have the capacity to program and modulate the cellular infiltrates of the tumor microenvironment (TME) and dysregulate inflammation to sustain tumor growth, while limiting immune responses against the tumor [1]. It has been well-documented that NSCLC cells express a Th2 cytokine pattern [2] and have the capacity to potently enhance the production of immunosuppressive cytokines such as IL-10 in the TME while simultaneously suppressing those mediators that promote specific cell-mediated immunity such as antigen presenting cell IL-12 production [3, 4]. This altered cytokine balance has been found to promote tumor growth [5]. Both tumor-derived and tumor-induced cytokines and growth factors in the TME have the capacity to suppress specific immune responses. Particularly important are IL-10, TGF-β, and PGE2 which have been noted to induce both FOXP3 and myeloid-derived suppressor cells (MDSC) [6–9].
Cancer cells may resist immune responses via several pathways. Lung cancer cells may avoid immune-mediated rejection through a process of immunoediting in which the cell-mediated immune response sculpts the tumor expression of highly immunogenic neoepitopes [10]. The theory of immunoediting, first proposed by Schreiber [11], has recently gained support in clinical lung cancer by the demonstration of environmental and genetic mechanisms that impact tumor–immune interactions [12]. By analysis of The Cancer Genome Atlas (TCGA) samples, Rooney et al. discovered genetic determinants that provide evidence for immunoediting in human tumors including non-small-cell lung cancer (NSCLC) and revealed tumor-intrinsic resistance to cytolytic activity. These findings are consistent with recent studies documenting that neo-antigens predicted by tumor genome meta-analysis correlate with increased patient survival [13] as well as investigations suggesting that sensitivity to immune checkpoint blockade is determined by the mutational landscape in NSCLC [14]. Strategies that reprogram the inflammatory infiltrate in the TME from a hyporesponsive to an immune active state have the potential for anti-tumor activity and tumor destruction [15].
By harnessing the immune system to specifically recognize cancer cells, immunotherapy has the potential for high specificity, low toxicity, and long-term protection against lung cancer. Although early attempts of immune stimulation for treatment of thoracic malignancies had not proven to be reliably effective, advances in cellular and molecular immunology and an improved understanding of tumor immunity and immune suppressive checkpoints has facilitated the development of promising immune based strategies against thoracic malignancies [16]. While there have been many different approaches to lung cancer immunotherapy, those that are most promising and/or in late stages of clinical development are immune checkpoint inhibitors and lung cancer vaccines. Programmed death-1 (PD-1) or PD-ligand 1 (L1) pathway checkpoint inhibitors have proven to be effective to induce a durable response in a minority of NSCLC patients, and will likely become the backbone of all cytotoxic (CD8+) T cell based cancer immunotherapies, given that they function at the effector phase of T cell activation. Further improvement of response and patient survival will most likely rely on combination strategies that can bring tumor antigen specific T cells into the tumors, and improve the effector function and/or the tumor immune suppressive milieu. In this chapter, we will discuss immunotherapy approaches that are most advanced in their clinical development for the treatment of lung cancer.
Immune Checkpoint Inhibitors for Lung Cancer Therapy
Despite the large number of tumor antigens induced by genetic and epigenetic alterations found in cancers [17] , tumors resist immune attack by inducing tolerance among tumor specific T cells and by expressing ligands that engage inhibitory receptors and dampen T cell functions within the TME [18]. One mechanism to circumvent immune tolerance in cancer is through the targeting of negative regulatory molecules. In both preclinical studies and early clinical trials , inhibitory antibodies to components of the B7 family, including the negative regulatory receptor, cytotoxic T lymphocyte antigen-4 (CTLA-4), and programmed death-1 receptor (PD-1), expressed on activated T cells, have proven promising targets for immunotherapy . By blocking inhibitory molecules or, alternatively, activating stimulatory molecules, these treatments are designed to release the brakes and/or enhance preexisting anti-cancer immune responses.
CTLA-4 Checkpoint
CTLA-4 is a molecule that can be upregulated at the priming phase of T cell activation, thus preventing autoimmunity and establishing tolerance to self-antigens via a homeostatic feedback loop [19, 20].Full T cell activation requires two signals. The first is initiated by T cell receptor binding to tumor-associated antigens (TAAs) presented by antigen presenting cells (APC) via Major Histocompatibility Complexes (MHC) Class I and Class II. The second signal is generated when the principal co-stimulatory receptor on the T cell, CD28, binds to B7 ligand subtypes CD80 and CD86 on the APC. The resulting dual signaling induces changes including antigen specific T cell proliferation, cytokine release, and amplification of the immune response. In response to T cell activation, CTLA-4 is upregulated and competes with CD28 for CD80 and CD86 binding on APCs, but with significantly higher affinity, therefore downregulating—or deactivating—T cell responses and APC function, resulting in a decreased immune response to TAAs and immune tolerance [21, 22]. Chronic T cell stimulation by TAAs results in persistently high CTLA-4 expression and T cells that are primed but no longer able to respond. CTLA-4 signaling also contributes to the immunosuppressive function of regulatory T cells (Treg) [23]; binding of Treg associated CTLA-4 to APC decreases APC function and effector T cell proliferation [22]. Therefore, CTLA-4 represents a critical immune checkpoint, controlling both the duration and the intensity of an immune response. Anti-CTLA-4 monoclonal antibodies block CTLA-4 signaling, improve T cell activation and proliferation, and thus amplify T cell-mediated immunity, which theoretically enhances the patient’s capacity to mount an anti-tumor immune response [21, 23].
Ipilimumab (Yervoy™) is a fully human IgG1 antibody against CTLA-4, the first checkpoint inhibitor that was approved by the US Food and Drug Administration (FDA) in 2011 for the treatment of melanoma [24]. In the first-line setting, ipilimumab was evaluated in a randomized double-blind phase II trial that compared two schedules of ipilimumab in combination with six cycles of carboplatin and paclitaxel to chemotherapy alone in 204 patients with stage IV NSCLC [25], and 103 patients with extensive-stage small-cell lung cancer (SCLC) [26]. Eligible patients received ipilimumab either with cycle 1–4 of chemotherapy (concurrent) or with cycle 3–6 (phased), followed by either ipilimumab or placebo every 12 weeks until progression. The primary end point was immune related progression free survival (irPFS) to take consideration of the potential initial “progression” followed by tumor stabilization or regression unique to the response to immunotherapy [27]. The concurrent schedule did not meet the primary endpoint (irPFS 5.5 months with hazard ratio (HR) 0.81, p = 0.81; overall survival 9.7 months; overall response rate (ORR) 21 %), however, the phased schedule showed an improvement compared with chemotherapy in irPFS (5.7 versus 4.6 months; HR 0.72; p = 0.05), OS (12.2 versus 8.3 months), and ORR (32 versus 18 %). The concurrent group had more adverse events (AEs) than phased or chemotherapy control groups (treatment-related AEs were 41, 39, and 31 %; grade 3/4 immune-related AEs were 20, 15, and 6 %), and was associated with one treatment related death (septic shock secondary to epidermal necrolysis). Another death occurred in the chemotherapy arm (secondary to neutropenic sepsis). Subset analysis showed better irPFS (HR, 0.55; 95 % CI, 0.27–1.12) and OS (HR, 0.4; 95 % CI, 0.22–1.03) with the squamous histology and phased schedule. A similar result was seen in the subset of patients with extensive-stage SCLC. Significantly improved irPFS with the phased schedule was seen compared with chemotherapy alone (6.4 versus 5.3 months; HR, 0.64; p = 0.03) and improved in RR (57 versus 49 %) and OS (12.9 versus 9.9 months). Based on these results, two randomized phase III trials are ongoing comparing standard chemotherapy with or without phased ipilimumab in patients with squamous only NSCLC (NCT01285609), or with extensive-stage SCLC (NCT01450761), with OS as the primary endpoint.
Tremelimumab is a fully human IgG2 antibody against CTLA-4, and was tested in the maintenance setting in a phase II trial compared with best supportive care (BSC) in patients who experienced disease control (CR, PR, or SD) after four cycles of platinum-based chemotherapy [28]. However, no improvement of PFS was observed (20.9 versus 14.3 % at 3 months), and 9 (20.5 %) patients treated with tremelimumab had grade 3/4 AEs. There are ongoing early phase clinical trials combining tremelimumab with anti-programmed death-ligand 1 (PD-L1) antibody MEDI4736 in patients with NSCLC (NCT02000947), or with gefitinib in EGFR mutant NSCLC patients (NCT02040064).
PD-1/PD-L1 Checkpoint
The PD-1/PD-L1 checkpoint occurs at the effector phase of T cell activation [20]. Activated T cells express PD-1, a T cell co-inhibitory receptor [29] shown to negatively regulate TCR signaling upon engagement of its ligands PD-L1 (B7-H1) [30] and PD-L2 (B7-DC) [31, 32]. While normal tissues express little PD-L1, this pathway can be hijacked by tumors to evade immune control by preventing T cells from evoking the immune system to attack. High expression of PD-L1 on tumor cells has been found to correlate with poor prognosis and survival in various cancer types [33, 34], and PD-L1 expression can be induced on many tumors and cells within the TME in response to inflammatory stimuli, where it can inhibit cytokine production and the cytolytic activity of PD-1+, tumor-infiltrating CD4+ and CD8+ T cells [33, 35, 36]. These properties make PD-1 and PD-L1 potentially promising targets for cancer immunotherapy . Indeed, blockade of the interaction between PD-1 and PD-L1 potentiates immune responses in vitro [37] and mediates preclinical anti-tumor activity [33, 34]; early testing of cancer patients with monoclonal antibodies that block either PD-1 or PD-L1 leads to tumor shrinkage and improvement in long-term survival [38, 39].
BMS-936559 is a fully human IgG4 (S228P) monoclonal antibody that inhibits the binding of PD-L1 to both PD-1 and CD80 and is the first anti-PD-L1 antibody to demonstrate activity in NSCLC patients. In a multicenter phase I trial, BMS-936559 administered every 14 days induced durable tumor regression (objective response rate of 6 to 17 %) and prolonged stabilization of disease (rates of 12–41 % at 24 weeks) in patients with selected advanced cancers, including NSCLC [38]. The objective response was seen in 5 of 49 patients (10 %) with NSCLC patients who received anti-PD-L1.
Nivolumab (Opdivo TM , BMS-936558) is a human monoclonal IgG4 antibody and was the first anti-PD-1 antibody to demonstrate activity in NSCLC patients [39]. In this phase I trial, NSCLC patients (among other tumor types) were treated with nivolumab 1, 3, or 10 mg/kg IV every 2 weeks. The patient response rate with the anti-PD-1 trial in lung cancer was 14 of 76 (18 %). Objective responses were observed across non-small-cell histologic types: in 6 of 18 patients (33 %) with squamous tumors, 7 of 56 (12 %) with non-squamous tumors, and 1 of 2 with tumors of unknown type. Given that the response rate was 3 % for the 1 mg/kg cohort compared with 24.3 % and 20.3 % for the 3 and 10 mg/kg cohort, respectively, the 3 mg/kg dose was selected for further studies. Drug-related AEs were seen in 53 % of patients such as fatigue, rash, diarrhea, and fever, however, only 5 % of which (6 % in the PD-L1 trial) were grade 3/4 including gastrointestinal, pulmonary (pneumonitis), hepatitis, and infusion reactions that required stopping treatment. Three patients who received the PD-1 targeted drug died of uncontrolled lung inflammation caused by the treatment.
The observed response to anti-PD-1/L1 therapy was quite unexpected. Although melanoma and renal-cell cancer are responsive to cancer immunotherapy (e.g., interleukin- 2 and anti–CTLA-4), NSCLC had been previously considered non-immunogenic and poorly responsive to immune-based therapies. Another important feature of anti-PD-1/L1 therapy was the durability of response across multiple tumor types. This was particularly notable given the advanced stage of disease and previous treatments of patients in these studies. This durability appeared to be greater than that observed with most chemotherapy and kinase inhibitors used in these diseases [40]. The therapeutic potential of these inhibitory pathways that places the brake on the immune system from recognizing cancers has been realized, and additional phase II trials of the PD-1 targeted agents and phase III trials involving patients with several cancers have been opened and are ongoing, including NSCLC. A phase III open label, randomized trial is ongoing, comparing first line nivolumab to investigator-choice chemotherapy in patients with PD-L1-positive tumors (NCT02041533), given that correlative studies of the phase I trial showed that PD-L1 expression by IHC was associated with a response to anti-PD-1 therapy. However, whether baseline PD-L1 expression can be a reliable predictive biomarker is still controversial [41]. In addition, a phase I trial is ongoing, evaluating nivolumab in combination with first-line platinum-based chemotherapy in NSCLC patients (NCT01454102) [42]. In the second line setting, two phase III randomized trials were launched comparing nivolumab with second-line chemotherapy (docetaxel) in non-squamous and squamous NSCLC (NCT01673867; NCT01642004). The trial in 272 patients with advanced squamous NSCLC was stopped early after showing a survival benefit (data not officially published yet). Patients were randomly assigned to treatment with either nivolumab 3 mg/kg IV q2W or docetaxel 75 mg/m2 IV q3W (open label) with OS as the primary endpoint. Median OS was 9.2 months (95 % CI, 7.3–13.3) in the nivolumab group compared to 6 months in the docetaxel group (95 % CI, 5.1–7.3), showing a 41 % reduction in the risk for death (hazard ratio, 0.59; 95 % CI, 0.44–0.79; P = 0.00025). Based on this data, nivolumab was approved by the FDA 3 months ahead of schedule for patients with advanced squamous NSCLC after progression on or after platinum-based chemotherapy. This is the first immunotherapy showing survival benefit and FDA-approved in the treatment of lung cancer. Phase I trial is currently evaluating nivolumab alone or in combination with ipilimumab in select tumor types including SCLC (NCT01928394).
Pembrolizumab (Keytruda TM , MK3475) is another humanized IgG4 anti-PD1 antibody and is the first FDA approved anti-PD1 antibody to treat advanced melanoma patients who have progressed on or after ipilimumab [43]. Preliminary results from a phase I trial [44] with pembrolizumab given at 2 mg/kg every 3 weeks (Q3W), 10 mg/kg Q3W, or 10 mg/kg Q2W in 262 patients with 5.4 months of median follow-up, showed ORR of 21 % by RECIST v1.1 in overall study population (26 % treatment naïve, 20 % previously treated) and 18 % in patients with squamous and 23 % in patients with non-squamous histology. The ORR was 33 % at 2 mg/kg Q3W, 21 % at 10 mg/kg Q3W, and 21 % at 10 mg/kg Q2W. In treatment-naïve patients, the 24-week PFS rate was 51 % and 6-month OS rate, 86 %. In previously treated patients, the 24-week PFS rate was 26 % and 6-month OS rate was 59 %. Out of half of the patients whose tumors were evaluated for PD-L1 expression by IHC, the ORR was 23 % in patients with ≥ 1 % PD-L1 staining and 9 % in patients with negative PD-L1 staining. The PFS and OS were longer in patients with PD-L1 strong-positive tumors (HR 0.52 and 0.59 respectively). On the other hand, treatment was tolerated with grade 3–5 drug-related AEs occurred in 24 (9 %) patients, most commonly pneumonitis. Ongoing trials are comparing two different schedules of pembrolizumab with standard chemotherapy (docetaxel) as second-line therapy in patients with NSCLC that are positive for PD-L1 expression (NCT01905657), or combined with standard chemotherapy and immunotherapy in an ongoing phase I trial (NCT02039674; NCT01840579).
Other anti-PD-L1 antibodies under early phase development include MPDL3280A and MEDI4736, both human IgG1 antibodies. A phase I trial that included 85 NSCLC treated with MPDL3280A reported 23 % of RR, higher (83 %) in tumors that were PD-L1 IHC positive (10 % or more tumors staining), and in former and current smokers (11 of 43) compared with non-smokers (1 of 10) [45, 46]. Treatment-related AEs occurred in two thirds of patients, but only 11 % of these AEs were grade 3/4 including fatigue, nausea, dyspnea, and emesis. Another ongoing phase I trial of MEDI4763 with NSCLC reported on the first 11 patients showed similar toxicities profiles and responses observed with other agents in this class in NSCLC patients [47]. Trials of MPDL3280A are ongoing in patients with tumors that are positive for expression of PD-L1, are ongoing including a single-arm phase II trial of MPDL3280A (NCT01846416; NCT02031458), and a randomized phase III trial comparing MPDL3280A with standard chemotherapy (docetaxel) (NCT02008227). In addition, an upcoming phase I trial is combining MPDL3280A with or erlotinib in NSCLC patients (NCT02013219). A phase Ib trial is evaluating MEDI-4736 in combination with tremelimumab in NSCLC patients (NCT02000947).
Lung Cancer Vaccines
While lung cancers express tumor antigens [48], they are often ineffective as APC [49]. In fact, the tumor’s lack of co-stimulatory molecules, in combination with the tumor’s production of inhibitory factors, promotes a state of specific T cell anergy [50, 51]. In the past, extensive efforts to produce an effective cancer therapy focused on methods to restore tumor antigen presentation, and T cell infiltration of the tumor were not associated with clinically significant benefit, likely due to the checkpoint inhibitions, especially PD-1 checkpoint at the effector phase of T cell activation. However, these efforts have regained interest as strategies to combine and potentially synergize with the checkpoint inhibitors. Two types of cancer vaccines are being evaluated in NSCLC, antigen-based or tumor cell-based vaccines.
Tumor Protein and Peptide Therapeutic Vaccines for Lung Cancer Therapy
Activated proto-oncogenes, inactivated tumor suppressor genes and genetic mutations have been linked to molecular events involved in lung cancer tumorigenesis and have led to the identification of TAAs ideal for the development of vaccines. A vaccine is an agent that elicits the adaptive host immunity (B or T cell responses) against specific disease. Therapeutic vaccines for cancer differ from the classical concept of vaccines. In contrast to prophylactic vaccines, which elicit protective immunity, therapeutic vaccines aim to induce strong antigen specific immune responses against active disease.
In the setting of the tumor, vaccination refers to an intervention that unmasks tumor antigens leading to generation of specific host-immune responses against the tumor. Proteins and peptides are processed via MHC molecules and presented by APC on the cell surface to T lymphocytes resulting in the generation of a specific immune response. Only a short segment of peptide sequences from the original tumor proteinare immunogenic, and epitopes are presented by MHC molecules according to a complex set of cellular rules. However, peptides are restricted to specific HLA types for presentation, which may not allow universal application to all patients. Therapeutic peptide cancer vaccines aim at inducing strong CD8 and CD4 T cell responses and require the involvement of host APC to efficiently present the peptide antigens for the activation of the respective T cell subsets. The rationale for the use of peptide vaccines is based on extensive preclinical studies that demonstrated the requirement of T lymphocytes for the eradication of solid tumors. Cytotoxic T lymphocytes (CTLs) or CD8 T cells represent the primary effector cells involved in tumor-specific immune-mediated destruction of cancer cells. CTLs recognize, engage, and destroy targets cells through the tri-molecular interaction of the antigen-specific receptor (TCR) on the CTL and peptides that are presented by the target cell to the CTL in the context of class I MHC, also referred to as human leukocyte antigens or HLA. All somatic cells express HLA molecules on their surfaces and use them to present antigens to T cells. Whole proteins within the cell are processed into small peptide fragments (8–10 amino acids in length) that are displayed on the cell surface in the context of HLA molecules. The HLA-peptide molecular complex enables CTLs to recognize TAAs and results in the targeted destruction of the cancer cell expressing these antigens by the CTL. Some primary cancers express adequate HLA molecules and are capable of being recognized and destroyed by TAA-specific CTLs. The identification and reintroduction of tumor associated specific peptides in increased concentration to the immune system via vaccination, activates and deploys the appropriate CTLs to destroy cancer cells. The use of a single peptide antigen or a few peptide antigens in a therapeutic cancer vaccine is based on the assumption that the initial induced anti-tumor response to one or few antigens will eventuate into a broad immune response to a multitude of TAAs following uptake of dying tumor cells and presentation by host APC.
The expression of the melanoma-associated antigen (MAGE) genes is silent in all normal cells except germ cells [52]. There have been more than 50 related MAGE genes identified thus far, and have been shown to play an important role physiologically and pathologically during embryogenesis, germ cell development, cell cycle progression, and apoptosis [52]. Nearly 75 % of small-cell lung cancers (SCLCs) and approximately 40 % of NSCLC express MAGE-3 and as a result this testis cancer antigen has received attention as an immunotherapy target. Atanackovic et al. reported on the successful induction of humoral and specific cell mediated immunity in early stage NSCLC (I and II) patients vaccinated with MAGE-3 protein [53]. Seventeen patients with MAGE-3 expressing NSCLC were analyzed in two groups, one receiving MAGE-3 protein alone and the other receiving MAGE-3 protein with adjuvant AS02B [53]. Of the 9 patients in the first cohort, 3 patients developed marginal Ab titers and another patient had a CD8 T cell response to HLA-A2-restricted peptide MAGE-3 271–279 [53]. In contrast, of 8 patients from the second cohort vaccinated with MAGE-3 protein and adjuvant, 7 patients developed antibody high titers to MAGE-3, and 4 had a strong concomitant CD4 T cell response to HLA-DP4-restricted peptide 243–258 [53]. One patient simultaneously developed CD8 T cells to HLA-A1-restricted peptide 168–176 [53]. Although the clinical relevance of the immune responses was not addressed, this study demonstrated the importance of CD4 T cell-mediated immunity that correlated with antibody production following vaccination, in addition to the traditionally understood involvement of antigen specific CD8 T cell response. Moreover, this study provides the foundation for further evaluating integrated humoral and cell mediated immune responses in vaccine strategies.
GlaxoSmithKline’s specific vaccine against the MAGE 3 expressing NSCLC consists of purified MAGE-A3 recombinant protein in liposomal formulation containing the AS02B immunoadjuvant system. Based on early studies of this vaccine in patients with NSCLC, the largest clinical trial is underway testing this vaccine (the Phase III MAGRIT trial).This trial was designed for post-surgical patients with resected stage IB to IIIA NSCLC and MAGE A3 + tumors randomized to placebo or vaccine after adjuvant chemotherapy. The study is powered for disease free survival (DFS) as the primary endpoint in the total population and in the cohort without postoperative chemotherapy [54]. A press release from GSK on March 30, 2014 reported that the trial enrolled 2312 patients but did not meet the primary endpoint of prolonged DFS.
After a long developmental phase, a mucin 1 (MUC1) peptide vaccine candidate in NSCLC patients has demonstrated increased survival in phase II clinical trials [55, 56] that provided the rationale for the initiation of phase III trials in large cohorts of lung cancer patients. The vaccine targets MUC1, a cell membrane glycoprotein over-expressed in many types of cancers including NSCLC, breast, colorectal, prostate, pancreatic, ovarian, and multiple myeloma. MUC1 expression in tumors promotes growth and survival and is associated with disease progression and poor prognosis. [57].
The BLP25 liposomal vaccine (L-BLP25, tecemotide) approach for lung cancer is a peptide liposomal vaccine to target the exposed core peptide of MUC1. The BLP25 lipopeptide vaccine consists of a 25-amino acid MUC1 sequence and contains a palmitoyl lysine residue at the carboxy terminal to enhance the incorporation of the lipopeptide into the liposome particle. The vaccine is a lyophilized preparation consisting of BLP25 lipopeptide, immunoadjuvant monophosphoryl lipid A, and three lipids (cholesterol, dimyristoyl phosphatidylglycerol, and dipalmitoyl phosphatidylcholine) to enhance the immunogenicity. The monophosphoryl lipid A is the toll-like receptor-4 agonist that activates DC and macrophages. The liposomal delivery system is designed to facilitate uptake by APC such that the lipopeptide is delivered into the intracellular space for presentation by MHC molecules to activate specific T cells that will identify and target cancer cells expressing MUC1. L-BLP25 cancer vaccine was evaluated in a phase III trial for the treatment of unresectable, stage III NSCLC who did not have PD after chemoradiation [58]. There was much optimism for this vaccine based on the favorable toxicity profile and the benefit in survival in patients with local and regional stage III NSCLC. After the primary treatment, 829 and 410 patients were randomized to tecemotide or placebo. The study did not meet the primary endpoint of improving OS, with the median OS increasing from 22.3 months in the placebo to 25.6 months in the tecemotide arm (HR, 0.88; p = 0.12). However, subset analysis of patients received concurrent chemoradiation showed significantly improved median OS for the 538 patients receiving tecemotide compared with the 268 patients randomized to placebo (30.8 versus 20.6 months; HR, 0.78; p = 0.016). Therefore, a randomized phase III trial comparing tecemotide with placebo in patients with stage III NSCLC treated with concurrent chemoradiation was started in March 2014.
Mounting evidence suggests that personalized therapy utilizing neoantigen-based therapeutic cancer vaccines could have efficacy [59, 60]. This could be particularly important in lung cancer because the mutational burden is among the highest compared to other malignancies (Lawrence 2013). In addition, neoantigens in lung cancer predicted by genome meta-analysis have been demonstrated to correlate with survival [13] as well as respond to checkpoint inhibitor therapy [14]. Both murine and human studies suggest that effective CTL responses can be generated in response to neo-antigens [61–63].
Dendritic Cell Vaccines for Lung Cancer
The predominant mechanism of anti-tumor immunity is a T cell mediated destruction of tumor cells. Specifically, the expansion of CTLs capable of recognizing tumor antigens presented in association with MHC molecules is the goal of most immunotherapy strategies. APC appear to play a central role in T cell activation by presenting tumor antigens and providing essential co-stimulatory signals necessary for the production of CTL. In optimal circumstances, APC can migrate and gain access to the TME , and overcome tumor-induced obstacles to have effective function. T cell activation results in the generation of CTL capable of recognizing and destroying cancer cells, and the production of cytokines , such as IFN-γ and TNFα, which can suppress both tumor cell proliferation and induction of angiogenesis [64, 65]. CTL can cause lysis of tumor cells mediated by perforin and/or Fas [64, 66]. Therefore, therapeutic efforts have focused on identifying tumor antigens, providing the antigens in immunogenic contexts, manipulatingT cell responses to increase the number of CTL, and thus augmenting their effector functions.
Dendritic cells (DCs) are potent APC that present TAAs to T cells and thereby initiate tumor-specific immunity [67–69]. DCs are bone marrow-derived leukocytes characterized by a high level of expression of MHC and co-stimulatory molecules [70]. As a result, they are capable of capturing antigens and producing largenumbers of immunogenic MHC-peptide complexes [70] and they migrate to secondary lymphoid organsto select and stimulate antigen-specific T cells. With the applicationof appropriate cytokines, one can generate large quantitiesof DC. Human DC maybe generated from proliferating CD34+ cells or from non-proliferating CD14+ progenitor cells. The production of DC from CD34+ cells requires GM-CSF and tumor necrosis factor alpha (TNF-α), whereas CD14+ cells require stimulation with GM-CSF and interleukin-4 (IL-4) to produce sufficient quantities of DC.
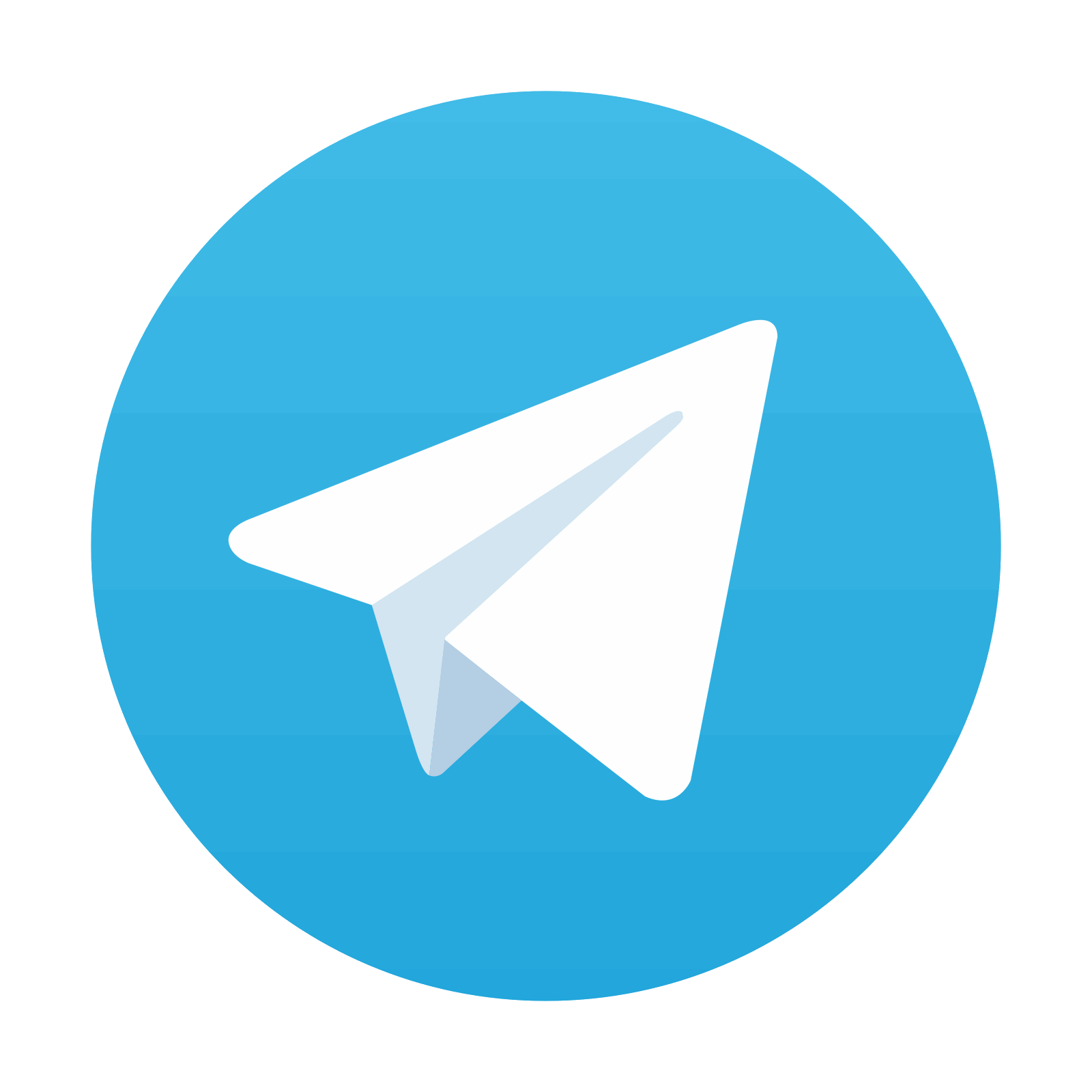
Stay updated, free articles. Join our Telegram channel

Full access? Get Clinical Tree
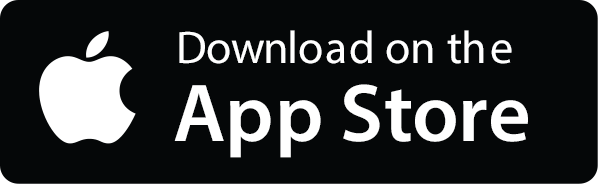
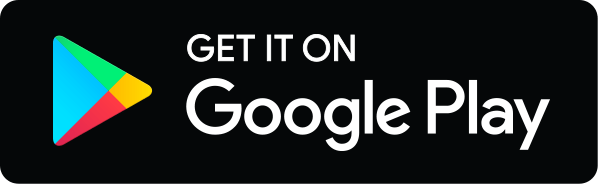