Fig. 13.1
BOLD parametric map in normal, RAS, RAS+PTRA, and RAS+PTRA+EPC pigs 10 weeks after RAS and 4 weeks after revascularization. Stenotic RAS kidneys show greater distribution of cortical green and yellow colors, suggesting hypoxia, which was slightly attenuated by PTRA, but markedly improved by PTRA+EPC
There are several explanations for the potential mechanisms of renoprotection exerted by EPC. Considerable evidence shows that EPC have the ability to engraft the damaged tissues. In line with these observations, we have previously shown in our swine model of ARAS that CM-DiI labeled EPC engrafted in medullary tubular structures 4 weeks after revascularization (Fig. 13.2) (Ebrahimi et al. 2012b). Furthermore, by using double fluorescence of CM-DiI and CD31 or cytokeratin staining, we were able to demonstrate that an important percentage of the injected EPC engrafted into blood vessels and tubules, assuming endothelial and tubular features (positive double staining with CD31 and cytokeratin) (Chade et al. 2009).
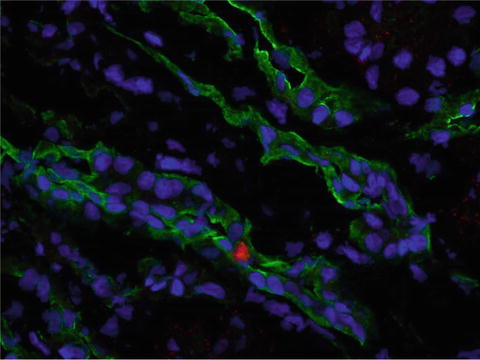
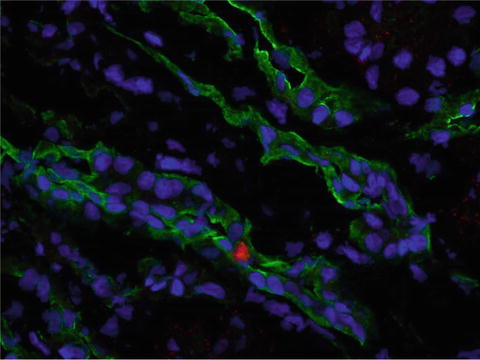
Fig. 13.2
Representative fluorescence of CM-DiI (red, 40×) EPC and cytokeratin (green) stained in the stenotic kidney 4 weeks after administration. Blue: DAPI nuclear stain. Injected EPC were incorporated into renal tubules
Previous studies have shown that the beneficial effect of EPC is mediated not only by their capacity for engraftment into damaged tissues, but also by transient secretion of vascular growth factors within this region (Rehman et al. 2003). In agreement, our experiments demonstrated that EPC isolated from both normal and RAS animals secrete vascular endothelial growth factor (VEGF) and endothelial nitric oxide synthase (eNOS) in culture media (Chade et al. 2009). Augmented availability of these potent angiogenic factors may have influenced the adjacent parenchyma, stimulating capillary proliferation and neovascularization.
Finally, recent data suggest that the regenerative effects of EPC on ischemic tissues could be attributed to the release of microvesicles. These small particles derived from the endosomal compartment play a key role in cell-to-cell communication by transferring proteins or RNA between cells (Deregibus et al. 2007). Cantaluppi and colleagues recently demonstrated in a rat model of ischemia reperfusion injury that microvesicles released from EPC exerted micro RNA-mediated protective effects characterized by a significant decrease in serum creatinine levels and by improvement in histological signs of microvascular and tubular injury (Cantaluppi et al. 2012). These observations suggest that micro-RNA shuttled by microvesicles may also contribute to their regenerative potential.
Mesenchymal Stem Cells
In contrast to EPC that need to be isolated from peripheral blood, MSC can be isolated from a variety of tissues, such as adipose tissue and bone marrow. This heterogeneous population of stromal cells has the ability to differentiate into a broad spectrum of cell types, including fibroblasts, epithelial cells, osteoblasts, chondroblasts, and adipocytes (Asanuma et al. 2010). Among the markers expressed by MSC are mesenchymal markers (CD44, CD90, and CD105), but not endothelial (CD31) or inflammatory (CD14 and CD45) markers. Furthermore, their immunomodulatory and tissue-trophic properties that promote tissue repair and decrease inflammation (Marigo and Dazzi 2011), make MSC unique candidates to protect the damaged kidney.
The homing potential of circulating MSC is primarily regulated by their adhesion to the endothelium (Potapova et al. 2009), yet MSC migration to the site of injury appears to be modulated by a variety of angiogenic and inflammatory cytokines such as VEGF, transforming growth factor (TGF)-β, interleukin (IL)-8, neurotrophin-3, tumor necrosis factor (TNF)-α, platelet-derived growth factor (PDGF), and SDF-1 (Schichor et al. 2006). Once MSC reach the damaged tissue, they secrete several bioactive molecules, such as cytokines, antioxidant, proangiogenic, and trophic factors, and impair migration and proliferation of inflammatory cells, stimulating repair and limiting the immune response (Charbord 2010).
Previous data from experimental rodent models of acute renal failure have shown that administration of MSC accelerated tubular proliferation, restoring renal structure and function (Morigi et al. 2004). Similarly, studies in animal models of ischemia/reperfusion injury uncovered a protective effect of MSC achieved by stimulating renal parenchymal regeneration (Morigi et al. 2004). In agreement, we have shown in swine ARAS that intra-renal administration of adipose-tissue derived MSC improved renal function and structure after revascularization and reduced oxidative stress, apoptosis, fibrosis, inflammation, and microvascular remodeling in the stenotic kidney (Eirin et al. 2012b). The protective effect of MSC in our study was partly mediated by improvement of microvascular remodeling (Fig. 13.3) and cortical reduction of oxidative stress and inflammation beyond the stenotic lesion via paracrine mechanisms. In support of this concept, we have shown that MSC secreted VEGF and TNF-α in the culture media. Nevertheless, 4 weeks after their infusion, MSC were detected in renal tissue sections, mostly at the interstitium, confirming their potential to engraft the damaged tissue.
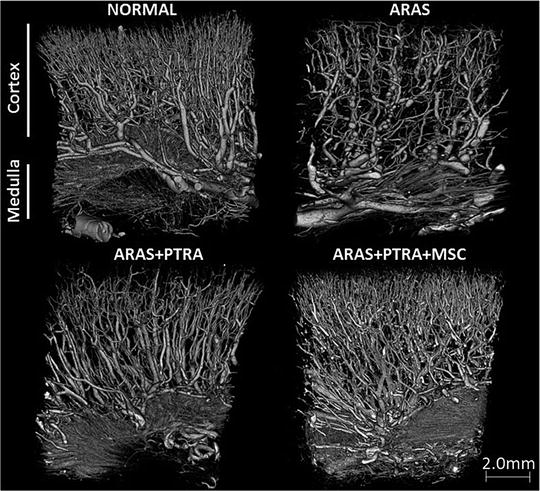
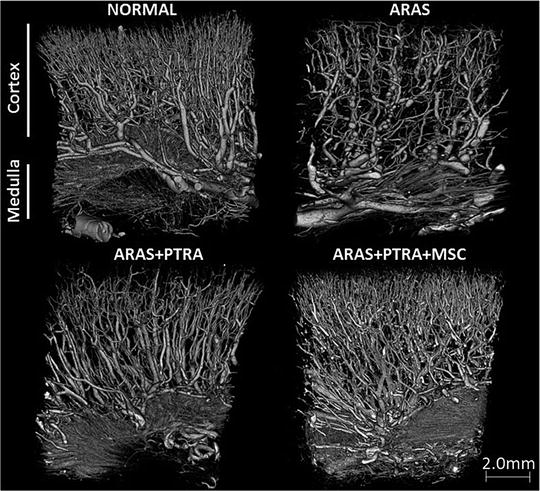
Fig. 13.3
Representative micro-CT 3D images (18 μm size voxels) of the stenotic-kidney showing improved microvascular architecture in ARAS animals treated with PTRA+MSC
Endothelial Progenitor Cells or Mesenchymal Stem Cells?
Based on the data presented above, it is reasonable to speculate on which of these treatment approaches would confer more efficient renoprotection in ARAS. Although is evident that MSC possess several advantages (easier to obtain, immunomodulatory properties, potent paracrine mechanisms), EPC might enhance neovascularization more efficiently in than MSC (Aguirre et al. 2010). However, a recent study from our group demonstrated that EPC and MSC induced comparable restoration of the renal microcirculation in RAS, although by employing different mechanisms (Zhu et al. 2012). While EPC showed direct pro-angiogenic potency achieved by upregulation the expression of VEGF and its receptors, MSC induced a substantial decrease in apoptosis and endoplasmic reticulum stress-induced apoptosis by reducing the expression of caspase-3 and C/EBP homologous protein (CHOP) in tubular cells through mechanisms involving cell contact. Therefore, the mechanisms of action and pathophysiology of these regenerative strategies may need to be considered during selection of cell-based therapies to improve the damaged kidney.
Safety and Future Strategies
Several clinical studies have shown that EPC and MSC can be well tolerated in patients with cardiovascular diseases and have an excellent safety record (Mathiasen et al. 2009; Wang et al. 2007). Likewise, in our studies, we have not observed any evidence of cellular rejection, micro-infarcts, or tumors masses in tissue sections from cell-treated pigs up to 12 weeks after injection (Chade et al. 2009, 2010; Ebrahimi et al. 2012b; Eirin et al. 2012b; Zhu et al. 2012). However, the ability to MSC to suppress the immune response and to promote tumor growth (Tolar et al. 2007) warrants long-term follow-up studies to determine the safety of MSC therapy.
Increasing evidence supports that modulation of ex-vivo culture conditions might potentiate the protective effects of cell-based approaches. For example, Peterson and colleagues demonstrated the beneficial effect of hypoxia preconditioning preserving MSC viability and function, through preservation of oxidant status (Peterson et al. 2011). Similar results were obtained after preconditioning with melatonin (Mias et al. 2008) or kallikrein (Hagiwara et al. 2008). Furthermore, manipulation of MSC to overexpress factors that increase their homing, adhesion, differentiation, or potency may also enhance their therapeutic potential (Chavakis et al. 2010). Results from these studies offer additional promise as novel therapies for improving MSC-induced renal protection.
Conclusion
The evidence available from a large number of studies identifies a potential for cell-based therapies for preservation of renal structure and function, alone or in conjunction to PTRA in experimental atherosclerotic renovascular disease. These studies suggested that the protective effects of EPC and MSC could be due to their ability to engraft the damaged tubules as well as secrete paracrine factors. No doubt, more experimental and clinical data, and appropriate guidelines are needed to define more precisely how to treat ARAS patients with either EPC or MSC.
Acknowledgements
These studies were supported by grants from the National Institutes of Health (HL85307, HL77131, DK77013, DK37608) and the American Heart Association.
References
Aguirre A, Planell JA, Engel E (2010) Dynamics of bone marrow-derived endothelial progenitor cell/mesenchymal stem cell interaction in co-culture and its implications in angiogenesis. Biochem Biophys Res Commun 400:284–291PubMedCrossRef
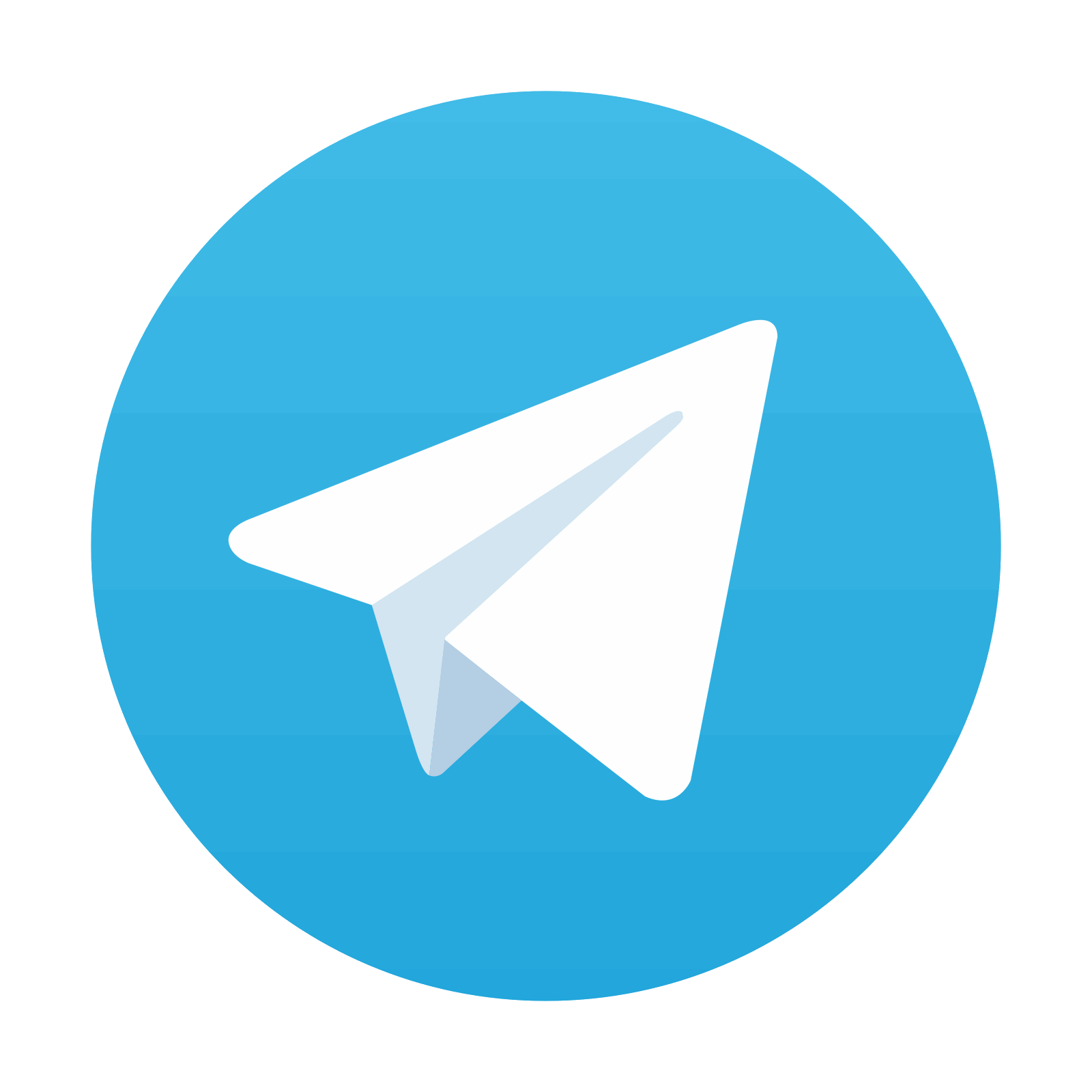
Stay updated, free articles. Join our Telegram channel

Full access? Get Clinical Tree
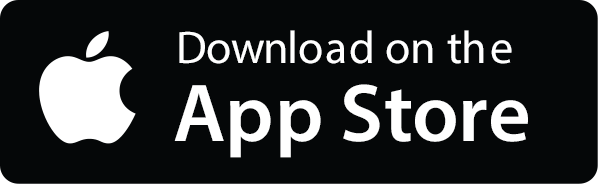
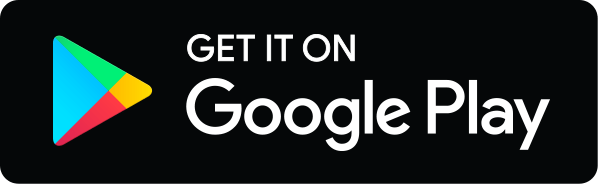