Fig. 1
Cross talk of the epigenetic alterations in cancer. The three classes of epigenetic modifications, such as DNA methylation, histone modifications, and noncoding RNA-based mechanisms, cause changes in the gene expression and genome-wide epigenetic alterations in cancer. DNA methylation, histone modifications, and posttranscriptional gene regulation by noncoding RNAs are not isolated events, but coordinated in higher-order chromatin structure. Thus, epigenetic modifications should not be interpreted independently, but only in combination with other modifications and within a context, leading to downstream transcriptional activation or repression
These recent advances have initiated significant interest in the use of natural, biological, or synthetic agents to prevent or delay the occurrence of cancer or its progression to invasive disease. The potential mechanisms of chemoprevention are the inhibition of cancer initiation with “blocking” agents and the inhibition of tumor progression with “suppressing” chemopreventive agents (Steward and Brown2013). Both mechanisms of cancer chemoprevention can be modified through epigenetic modulating agents, either natural or synthetic. Natural dietary compounds, including green tea polyphenols, soy isoflavones, isothiocyanates derived from cruciferous vegetables, and garlic-derived organosulfur compounds, have the strong potential to affect the gene expression via the epigenetic mechanisms, without the adverse toxic effects associated with synthetic epigenetic inhibitors used in chemotherapy. Another key aspect of the chemopreventive action of polyphenols and other dietary factors is their potential ability to selectively target cancer cells, while maintaining negligible cytotoxicity to normal cells (Khan et al. 2012).
2 Major Epigenetic Mechanisms in Cancer
2.1 DNA Methylation
The key epigenetic modification in mammals is the DNA methylation of cytosine, located 5′ to guanosine in the CpG dinucleotide (Szyf 2005; Herman and Baylin 2003). The covalent addition of a methyl group to the carbon-5 position of cytosine in DNA is catalyzed by the enzyme family of DNMTs, with S-adenosyl-methionine (SAM) acting as a universal methyl donor (Szyf 2005). While DNMT1 is mainly involved in the maintenance of DNA methylation after replication, DNMT3A and DNMT3B interact with transcription machinery and mediate de novo methylation (Szyf 2005). A number of studies have shown changes of DNMT expression (mainly DNMT1 and DNMT3B overexpression) in several cancer types (Girault et al. 2003; Agarwal et al. 2013; Yu et al. 2014).
CpG nucleotides are non-randomly distributed throughout the genome. Whereas solitary CpG dinucleotides are infrequent, CpG dinucleotides are often clustered in CpG-rich regions of DNA (CpG islands), with lengths from 0.5 to 4 kb. In normal cells, CpG islands are not methylated and are frequently associated with the transcription start site (Szyf 2005). During the process of carcinogenesis, paradoxical changes in DNA methylation patterns were observed. The “methylation paradox” is characterized by global hypomethylation followed by regional hypermethylation changes of tumor suppressor gene promoters. Global DNA hypomethylation may activate or “unlock” repetitive elements that could affect genome stability or could lead to transcriptional activation of latent viruses or oncogenes, whereas regional hypermethylation could lead to transcriptional silencing of tumor suppressor genes, which causes their inactivation, leading to malignant transformation (Herman and Baylin 2003). Patterns of tumor suppressor gene hypermethylation show tumor-type specificity (Esteller 2007). DNA methylation in cancer cells is now believed to be one of the major causes of the transcriptional silencing of tumor suppressor genes in carcinogenesis. Accumulating evidence demonstrates aberrant DNA methylation in most human cancers, including colon, breast, lung, and oral carcinomas (Esteller 2007; Belinsky et al. 2002; Supic et al. 2009, 2011a, b). A number of recent studies have demonstrated that dietary phytochemicals could have an important role in cancer chemoprevention by affecting DNA methylation (Shukla et al. 2014).
2.2 Histone Posttranslational Modifications
Histone modifications are posttranslational covalent modifications of histone tails, which result in changes to chromatin structure and gene activity. Histone tails can be modified by acetylation, methylation, phosphorylation, poly-ADP ribosylation, sumoylation, ubiquitination, citrullination, and proline isomerization (Jenuwein and Allis 2001; Tollefsbol 2009). Histone posttranslational modifications are complex, dynamic, and highly coordinated in chromatin remodeling and gene regulation. Different combinations of histone modification and histone variants, often referred as “histone code,” determine the interactions of histones with DNA and regulate the chromatin structure and its accessibility to transcription factors (Margueron et al. 2005; Tollefsbol 2009).
Histone acetylation of lysine residues (K) by histone acetyltransferases (HATs) neutralizes the positive charge on lysine and releases the histone tails from the negatively charged DNA. Histone acetylation causes decondensation of chromatin, thus allowing its accessibility to transcriptional factors (Tollefsbol 2009). The reverse process is histone deacetylation, catalyzed by HDACs, which leads to chromatin condensation and the suppression of DNA transcription (Mahlknecht and Hoelzer 2000) (Fig. 2).
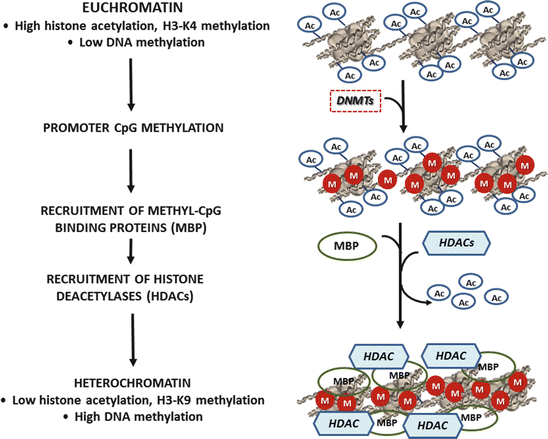
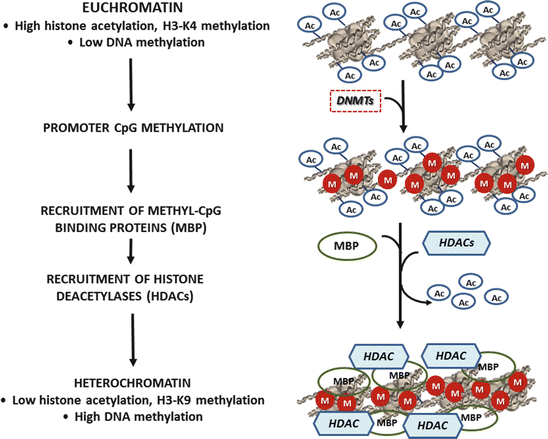
Fig. 2
The Epigenetic Control of Chromatin Organization. Euchromatin is characterized by low level of DNA methylation, high level of hyperacetylation of histones, and H3-K4 methylation. Promoter CpG methylation by DNMTs leads to the binding of MBPs that recruit histone deacetylases (HDACs) and histone methyltransferases (HMT). In contrast, heterochromatin shows hypermethylation of DNA, hypoacetylation of histones, and H3-K9 methylation that leads to the chromatin condensation and suppression of the DNA transcription
Histone methylation of lysine (K) and arginine (R) residues of histones H3 and H4 has been associated with both the activation and repression of transcription, depending on the modification target, type of amino acid, and its position in the histone tail (Jenuwein and Allis 2001; Tollefsbol 2009). While methylation of the residue of the fourth lysine on histone 3 (H3K4) leads to transcriptional activation, methylation of the ninth lysine on histone 3 (H3K9) leads to transcriptional repression. Histone methylation does not alter the charge of the histone tails but influences the chemical characteristics of histones and their affinity to transcription factors and other regulatory proteins. Histone methylation is catalyzed by a family of enzymes named histone methyltransferases (HMTs), whereas the removal of methyl groups is catalyzed by the histone demethylases (HDMs) (Margueron et al. 2005; Miremadi et al. 2007).
Histone phosphorylation is involved in diverse biological processes including mitosis, gene transcription, DNA repair, and cell cycle progression (Banerjee and Chakravarti 2011). The core histones and histone H1 undergo phosphorylation of serine and threonine residues by H1 and H3 kinases. Phosphorylation of histones during mitosis and meiosis is involved in chromosome condensation, whereas the same modification during interphase weakens H1 binding to DNA, thus promoting free access of transcriptional factors to the DNA and stimulating the gene expression. H3 phosphorylation at serine-10 has been associated with the transcriptional activation of the early response genes c-fos and c-jun (Kouzarides 2007).
Histone ubiquitination at lysine residues of histones H2A and H2B has a role in gene transcription control. Mono-ubiquitination of histone H2B is required for methylation of histone H3 at K4 and K79, where histone ubiquitination seems to promote methylation by recruiting proteasomal ATPases using ubiquitin-modified H2B (Jenuwein and Allis 2001; Tollefsbol 2009). The global disruption of the histone acetylation and methylation, commonly found in cancer, has been associated with malignant transformation through transcriptional repression of the tumor suppressor genes (Jenuwein and Allis 2001; Tollefsbol 2009). Aberrant expression of histone-modifying enzymes (HDAC, HAT, HMT, and HDM) has also been observed in cancer cells (Mahlknecht and Hoelzer 2000; Tollefsbol 2009). A large number of dietary bioactive factors have been shown to have modifying effects on various histone-modifying enzymes (Tortorella et al. 2014).
2.3 RNA-Associated Silencing RNA-Interference and MicroRNAs
Noncoding RNAs, including microRNAs and small-interfering RNAs, have a very important role in the posttranscriptional regulation of gene expression. Noncoding RNAs act either by complete complementary base pairing, which results in mRNA degradation, or by a partial base pairing, which leads to translational inhibition of the targeted mRNA. MicroRNAs regulate genes with important cellular functions such as cell proliferation, differentiation, and apoptosis. Changes in the expression of genes coding miRNAs are a common event in cancer. Depending on their target, subsets of miRNAs that are downregulated in cancer may act as putative tumor suppressor genes, whereas upregulation of other miRNAs suggests that many miRNAs may act as oncogenes (Shivdasani 2006; Negrini et al. 2007).
Accumulating evidence suggests that epigenetic modifications cannot be interpreted independently, but only in combination with other modifications and within a context that leads to downstream transcriptional activation or repression. DNA methylation, histone modifications, and RNAi are not isolated events but coordinated in higher-order chromatin structures. DNA methylation leads to the binding of methylcytosine-binding proteins (MBPs), which recruit HDACs and HMTs (Herman and Baylin 2003; Tollefsbol 2009) (Fig. 2). Furthermore, noncoding miRNAs can regulate the expression of DNMTs and trigger locus-specific histone modifications and DNA methylation, causing gene silencing (Zilberman et al. 2003). In addition, the genes for miRNAs can be downregulated as a result of hypermethylation (Lujambio et al. 2007). Aberrant changes of miRNA expression appear to be one of the key epigenetic mechanisms involved in regulating gene expression during carcinogenesis (Shivdasani 2006; Negrini et al. 2007). In addition, accumulating evidence demonstrates that dietary factors can alter miRNA expression in cancer cells. Thus, targeting specific miRNAs with dietary phytochemicals could be a novel approach for cancer chemoprevention (Shah et al. 2012; Davis and Ross 2008).
3 Dietary Factors in Epigenetic Cancer Chemoprevention
Certain dietary factors, e.g., folate, tea polyphenols, phytoestrogens and isoflavones, curcumin, and resveratrol, are thought to have a chemoprotective effect on the development and the progression of cancer. Many bioactive phytochemicals could potentially alter the expression of key tumor suppressor genes or oncogenes through modulation of DNA methylation and chromatin modification in cancer (Figs. 3 and 4). Bioactive natural compounds appear to act through the decrease of the DNMTs or HDACs gene expression in cancer cell lines and in vivo. In addition, these bioactive dietary factors resulted in modulating tissue levels of miRNAs and their target genes (Shah et al. 2012). Most of the studies were performed in vitro using different cancer cell lines, or in animals, and few studies were intervention studies on human subjects.
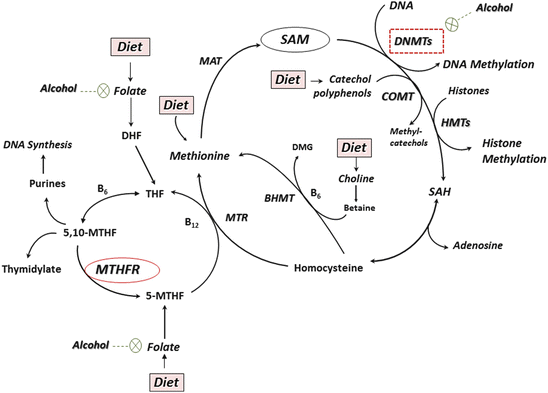
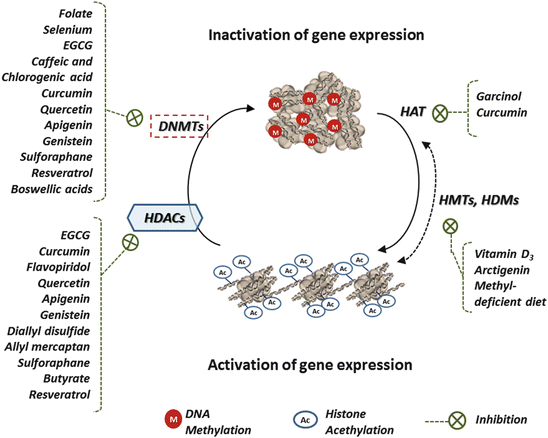
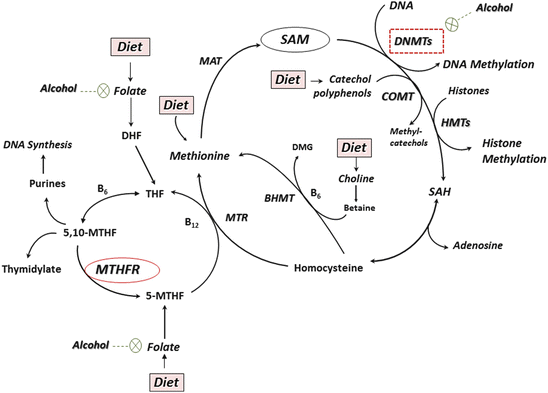
Fig. 3
A schematic representation of the one-carbon metabolism, transmethylation reactions, and steps that are inhibited by alcohol. Dietary folate is converted to 5 methyl tetrahydrofolate (5-MTHF) by the enzyme methylene tetrahydrofolate reductase (MTHFR). The transfer of methyl group from 5-MTHF to homocysteine results in the methionine synthesis. Methionine is the precursor for S-adenosylmethionine (SAM), the primary methyl donor for numerous reactions, including protein, RNA, DNA, and histone methylation. Methyl groups from SAM are transferred to DNA by DNA methyltransferases (DNMTs) and to histones by histone methyltransferases (HMTs). DNA methylation of CpG dinucleotides and histone methylation lead to the altered chromatin remodeling and gene expression. The MTHFR 677C → T polymorphism reduces enzyme activity and could play a key role in interactions of one-carbon metabolism with alcohol, which could affect DNA methylation levels. COMT catechol-O-methyltransferase, BHMT betaine homocysteine methyltransferase, DHF dihydrofolate, DHFR dihydrofolate reductase, MAT methionine adenosyl transferase, MT methyltransferase, MTR methionine synthase, SAH S-adenosylhomocysteine, THF tetrahydrofolate, TS thymidylate synthase
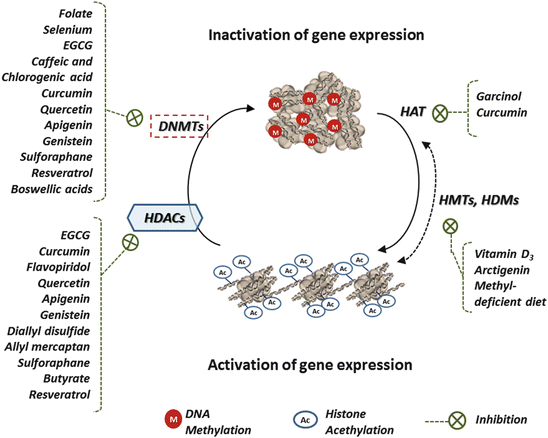
Fig. 4
The chemopreventive effects of dietary factors on specific epigenetic alterations. Dietary chemopreventive agents can affect DNMTs activity and the DNA promoter methylation status, as well as the HDAC activity and the pattern of histone modifications, thereby restoring the epigenetically silenced genes in cancer cells that regulate the cell cycle and apoptosis
The first study indicating this phenomenon showed that maternal diet supplemented with methyl donors, including folate, affected agouti gene expression in Avy/a mice, as a result of retrotransposon hypermethylation, and caused an altered coat phenotype (from a normal yellow to brown) in the genetically identical offspring (Wolff et al. 1998; Waterland and Jirtle 2003). Prenatal famine in humans influences phenotypic changes in offspring caused by DNA methylation changes that persist in the next generation offspring (Tobi et al. 2009; Heijmans et al. 2008). Individuals exposed to severe famine during childhood and adolescence, during the “Dutch Hunger Winter” of 1944–1945, had a decreased risk of developing colorectal cancer and a decreased methylation of a panel of cancer-related genes (Hughes et al. 2009). A reduced DNA methylation of the imprinted insulin-like growth factor 2 (IGF2), persisted six decades later, compared to their unexposed, same-sex siblings (Heijmans et al. 2008). A recent study showed that prenatal dietary supplementation with polyunsaturated fatty acid was associated with higher methylation levels in infants at IGF2/H19 imprinted regions. In addition, in the same study DNA methylation states in infants were associated with maternal body mass index (Lee et al. 2014). The data from these studies indicate that peri-conceptional, prenatal, and early-life conditions can cause epigenetic changes in humans that persist throughout life and could cause cancer.
Here we summarize the major phytochemicals that exert cancer-protective effects through modulation of epigenetic mechanisms, classified as essential micronutrients (folate and other nutrients of one-carbon metabolism, vitamin D, vitamin A, and selenium), polyphenol catechins (tea polyphenols—epigallocatechin gallate and epicatechin gallate, and coffee polyphenols—caffeic acid and chlorogenic acid), flavonoids (curcumin and difluorinated curcumin, garcinol, apigenin, quercetin, flavopiridol), isoflavonoids (genistein), phytoestrogen lignans (arctigenin), myco-strogens (zearalenone), organosulfur compounds(diallyl disulfide, S-allyl-mercaptocysteine, allyl mercaptan), isothiocyanates (sulforaphane and phenethyl-isothiocyanate), stilbenoids (resveratrol and pterostilbene), cyclic terpenoids (boswellic acids), plant alkaloids (mahanine, berberine), and short-chain fatty acids (butyrate).
4 Essential Micronutrients
Folate and other nutrients of one-carbon metabolism, such as vitamin B2, B6, and B12, are the main source of methyl groups or act as coenzymes for the one-carbon metabolism that regulates methyl transfer and DNA synthesis (Anderson et al. 2012). One-carbon metabolism is essential for the synthesis of S-adenosyl-l-methionine (SAM), universal methyl donor for various cellular reactions including DNA methylation and histone methylation (Fig. 3). Extensive evidence has accumulated suggesting that folate deficiency plays a significant role in developing several tumors including cancers of the colorectum, lung, pancreas, esophagus, stomach, cervix, and breast, as well as neuroblastoma and leukemia (Kim 1999). Head and neck cancer patients with the high dietary intake of micronutrients involved in one-carbon metabolism and antioxidant activity (folate, vitamin B12, and vitamin A) and cruciferous vegetable, compared with those in the lowest intake, showed significantly less tumor suppressor promoter methylation, assessed using the Illumina Goldengate Methylation Cancer Panel (Colacino et al. 2012). Low consumption of folate, vitamin A, vitamin B1, potassium, and iron in colorectal cancer patients was associated with the increased promoter hypermethylation of p16, p14 (ARF), or hMLH1 genes (Mas et al. 2007).
Several studies have established a connection between cancer susceptibility and methyl-group metabolism genes, including methylene tetrahydrofolate reductase (MTHFR), a key enzyme involved in folate metabolism and DNA synthesis (Fig. 3). MTHFRC677T genotype was associated with RASSF1A promoter hypermethylation in bladder and oral cancer (Cai et al. 2009; Supic et al. 2011a). Carriers of the 677 T allele of MTHFR gene in patients with colorectal, breast, or lung tumors had global hypomethylation, while patients with methionine synthase 2756GG genotype showed promoter hypermethylation in a large panel of tumor suppressor genes, including p16, p14, hMLH1, MGMT, APC, DAPK, GSTP1, BRCA1, RAR-β 2, CDH1, and RASSF1 (Paz et al. 2002). An interaction between low dietary folate and high alcohol intake in colorectal cancer patients resulted in concordant DNA methylation of tumor suppressor genes (van England et al. 2003). In addition, a significant interaction between heavy drinking and MTHFR667TT genotype, observed in oral cancer patients, resulted in the multiple DNA methylation of tumor suppressor genes (Supic et al. 2011a). These findings indicated that polymorphisms in genes encoding enzymes involved in folate metabolism could be an alternative mechanism that modulates the epigenetic effects of environmental factors. Interaction of folate intake or one-carbon metabolism-related polymorphisms with environmental factors, such as alcohol, could cause changes in DNA methylation (Fig. 3). Future investigations might predict individual susceptibility to cancer and provide science-based recommendations for methyl donors intake, depending on the individual genetic profile exposure for cancer prevention.
Retinoic acid (RA), generated via oxidative conversion from β-carotene absorbed from fruits and vegetables, binds to nuclear retinoic acid receptors (RAR) and heterodimerize with retinoid X receptors (RXR). Subsequently, RA modulates transcription through its response elements in the promoters of target genes, including p21 and AP-1, and exerts the pro-apoptotic actions (Stefanska et al. 2012a). It has been demonstrated that the treatment of noninvasive MCF-7 cells with RA caused the decrease in promoter methylation, consequent increase in the RARβ2 and PTEN (phosphatase and tensin homologue) expression, and the inhibition of breast cancer cell growth (Stefanska et al. 2012b). In the combined treatment, butyrate and RA inhibited proliferation of MCF-7 cells (Andrade et al. 2012). Thus, retinoids have been suggested as a potential epigenetic strategy for the cancer treatment, especially in breast cancers in which RAR β is epigenetically altered.
Vitamin D3, synthesized upon sun exposure and from the diet, binds to vitamin D receptors (VDR). Upon ligand binding, VDR heterodimerize with RXR or RAR, and regulate the transcription of more than a hundred genes, involved not only in calcium and phosphate homeostasis but also in cell proliferation, differentiation, and apoptosis (Chung et al. 2009). Accumulating results revealed the association between vitamin D deficiency and vitamin D-related genes with different cancer types, including breast, prostate, colorectal, oral, lung cancer, melanoma and acute myeloid leukemia (Feldman et al. 2014; Davis and Milner 2011; Zeljic et al. 2012, 2014, Puccetti et al. 2002). In addition, the gene expression of VDR and CYP24A1 gene, coding for 24-hydroxylase, enzyme involved in the vitamin D catabolism, and CYP27B1, coding for 1α-hydroxylase, involved in anabolism, is mediated by epigenetic modifications (Hobaus et al. 2013; Fetahu et al. 2014). In highly invasive MDA-MB-231 cells, exposure to vitamin D3 resulted in hypomethylation and induction of p21, PTEN, and RARβ2 expression (Stefanska et al. 2012a, b). Vitamin D3-mediated promoter demethylation induced the expression of E-cadherin and differentiation of aggressive triple-negative breast cancer cells (Lopes et al. 2012). Dietary vitamin D intake was strongly negatively associated with the Wnt antagonist gene DKK1 methylation in a large cohort of Canadian colorectal carcinoma patients (Rawson et al. 2012). In vitro studies demonstrated that calcitriol [1 alpha,25 Dihydroxyvitamin D(3)], an active metabolite of vitamin D, synergized the pro-apoptotic effect of HDAC inhibitors sodium butyrate (NaB), a natural short-chain fatty acid, and trichostatin A (TSA), a synthetic HDAC inhibitor, in the prostate cancer cells LNCaP, PC-3, and DU-145 (Rashid et al. 2001). Calcitriol inhibited the expression of several HDMs and induced the expression of others (Pereira et al. 2011, 2012). In the colon cancer SW480-ADH cells, calcitriol increased the expression of the HDM Jumonji JMJD3, which upregulated the epithelial-to-mesenchymal transition inducers SNAIL1 and ZEB1, while it downregulated the epithelial proteins, including E-cadherin (Pereira et al. 2011). Novel data indicate that calcitriol exerts its regulatory role via Jumonji C (JmjC) and lysine-specific demethylase (LSD) families of the HDMs (Pereira et al. 2012). In addition, JMJD3 knockdown decreases the expression of miR-200b and miR-200c, two microRNAs targeting ZEB1 RNA (Pereira et al. 2012). In another study, calcitriol upregulation of the expression of miR-627 in the colorectal cancer cells HT-29 and in the mice tumor xenografts led to lower levels of KDM2A, one of the direct targets of miR-627 (Padi et al. 2013). These findings indicate that the antiproliferative effect of calcitriol on the expression of HDMs may well be indirect and could be mediated by microRNAs.
Selenium is an essential micro-element with antioxidant and pro-apoptotic effect (Davis et al. 2000; Davis and Uthus 2002). In the rat colon and liver, selenium deficiency causes global hypomethylation and promoter methylation of p53 and p16gene (Davis et al. 2000). Selenium inhibits DNMT directly interacting with enzyme and indirectly influencing plasma homocysteine level and the SAM:SAH (S-adenosyl-l-homocysteine) ratio in a rat model (Davis and Uthus 2002; Uthus and Ross 2007). In an azoxymethane-induced rat colon carcinogenesis model, combination of selenium and green tea revealed more effective suppression of colorectal oncogenesis than either agent alone. Rats fed the combination diet showed marked reduction of DNMT1 expression and induction of histone H3 acetylation, which were accompanied by restoration of SFRP5, reduced ß-catenin nuclear translocation, Cyclin D1 expression, and cell proliferation (Hu et al. 2013a). In human colon cancer, selenium plays a role in chemoprevention by inhibiting DNMTs, thus suppressing DNA methylation (Fiala et al. 1998). However, in another study treatment of colorectal cancer cells Caco-2 and HCT116 with different concentrations of selenium methylselenocysteine and selenite, either alone or in combination with sulforaphane and iberin, did not impact changes in gene-specific methylation of p16 and ESR1, global methylation of LINE-1 elements, or DNMT expression (Barrera et al. 2013).
5 Catechol-Structured Polyphenols
Epigallocatechin-3-gallate (EGCG), the major green tea polyphenol catechin, was associated with the lower incidence of a number of cancers (Yang et al. 2001; Tollefsbol 2009). Various findings indicate that EGCG is the potent inhibitor of catechol-O-methyltransferase (COMT) and DNMT activity, in a dose-dependent manner (Lu et al. 2003; Fang et al. 2003). Treatment of human esophageal cells, colon cancer cells, and prostate cancer cells with EGCG inhibits DNMT1 activity in a concentration- and time-dependent manner, leading to the hypomethylation and restored gene expression of p16, RAR β, MGMT, and hMLH1 (Fang et al. 2003). Decreased activity of human telomerase reverse transcriptase (hTERT) in cancer cells is associated with increased DNA methylation of its promoter (Berletch et al. 2008). EGCG treatment inhibits expression of DNMTs leading to the suppression of hTERT, the suppression of cell viability, and the induction of apoptosis in human breast cancer cells (Mittal et al. 2004; Meeran et al. 2011; Berletch et al. 2008). The treatment with natural compounds, EGCG, genistein, withaferin A, curcumin, resveratrol, and guggulsterone, resulted in the decrease of the DNMTs gene expression and the methyl-binding proteins MeCP2 in the breast cancer cell lines (Mirza et al. 2013). EGCG decreased the expression and the levels of DNMTs and HDAC, which resulted in the re-expression of silenced tumor suppressor genes, p16INK4a and p21 in human skin cancer cells, and increased levels of histones acetylation in human skin cancer cells A431 in a dose-dependent manner (Nandakumar et al. 2011).
By miRNA microarray analysis, EGCG treatment was found to modify the expressions of a number of the miRNAs in human hepatocellular carcinoma cell line, as well as miRNA-16 and its anti-apoptotic target Bcl-2, that lead to the induction of apoptosis (Tsang and Kwok 2010). These findings indicate that natural tea polyphenols and EGCG could have the strong potential to reverse the epigenetic changes, without the adverse toxic effects associated with synthetic DNMT or HDAC inhibitors used in chemotherapy.
Caffeic acid and Chlorogenic acid are catechol-structures polyphenols from coffee or black tea that inhibit the DNMTs activity in various human cancer cell lines. Catechol polyphenols may indirectly inhibit DNMT through the increased formation of SAH, during the COMT-mediated O-methylation of these dietary agents, thus changing the SAM:SAH ratio (Lee and Zhu 2006) (Fig. 3). Treatment of human breast cancer cells MCF-7 and MAD-MB-231 with caffeic acid or chlorogenic acid inhibited general DNA methylation in a concentration-dependent manner, and the regional methylation of the RAR β gene promoter (Lee and Zhu 2006).
6 Flavonoids
Curcumin (diferuloylmethane), flavanoid from the rhizome of the plant Curcuma longa, has anticancer activity both in vitro and in vivo (Kunnumakkara et al. 2008). Genome-wide methylation microarrays revealed that in contrast to the generalized, nonspecific global hypomethylation observed with 5-aza-2′-deoxycytidine, curcumin treatment of colorectal cancer cells HCT116, HT29, and RKO caused methylation changes in selected, partially methylated loci, instead of fully methylated CpG sites (Link et al. 2013).
Curcumin upregulates or downregulates histone acetylation, depending on the cell type. In brain cancer cells treated with curcumin, H4 histones are hypoacetylated (Kang et al. 2006), as opposite of prostate cancer cells treated with curcumin, where H3 and H4 histones are hyperacetylated and where curcumin induces apoptosis by the involvement of Bcl-2 family genes and p53 (Shankar and Srivastava 2007). Curcumin inhibited HDACs and HPV expression, upregulated p53, pRb, p21, and p27 in cervical cancer cells SiHa and SiHaR, and resulted in sensitization of cervical cancer cell toward cisplatin, lowering the dose of the chemotherapy (Royt and Mukherjee 2014) In addition, curcumin sensitized tumor cells to the chemotherapeutic drugs cyclophosphamide and paclitaxel through the modulation of PKC, telomerase, NF-kappa B, and HDAC in breast cancer (Royt et al. 2011)
Combined administration of curcumin and emodin, a bioactive factor isolated from the root and rhizome of Rheum palmatum, widely used in traditional Chinese medicine, synergistically inhibited proliferation, survival, and invasion of breast cancer cells through upregulation of miR-34a (Guo et al. 2013). Treatment of colon cancer cells with curcumin and synthetic analogues of curcumin induced apoptosis and reactive oxygen species (ROS) and decreased specificity protein (Sp) transcription factors and Sp-regulated genes, including the epidermal growth factor receptor (EGFR), survivin, bcl-2, cyclin D1, and NFκB, by targeting microRNAs (miR-27a, miR-20a, and miR-17-5p) (Gandhy et al. 2012). The potential effects of curcumin treatment on miRNA expression profiles in a pancreatic cancer cell line showed altered expression, with miRNA-22 upregulated, while miRNA-199a downregulated (Sun et al. 2008). In addition, curcumin reduced the expression of Bcl-2 by upregulating miRNA-15a and miRNA-16 in MCF-7 breast cancer cell line (Yang et al. 2010), thereby inducing apoptosis. Curcumin exerted inhibitory effects on proliferation, migration, and invasion in nasopharyngeal carcinoma by inhibiting the expression of miR-125a-5p, which led to the upregulation of p53 expression (Gao et al. 2014). Another miRNA, MicroRNA-200a/b, was overexpressed after curcumin treatment, and this was accompanied with the increase of apoptotic Bcl-2, Bax, and Bad levels in hepatocellular carcinoma cell lines (Liang et al. 2013). Difluorinated curcumin, a novel analogue of the curcumin, restores tumor suppressor PTEN expression in chemo-resistant colon cancer cells HCT116 and HT-29 through downregulation of key oncomir miR-21 (Roy et al. 2013), which suggests that this bioactive agent could be potentially used in chemotherapy-resistant colorectal cancer treatment.
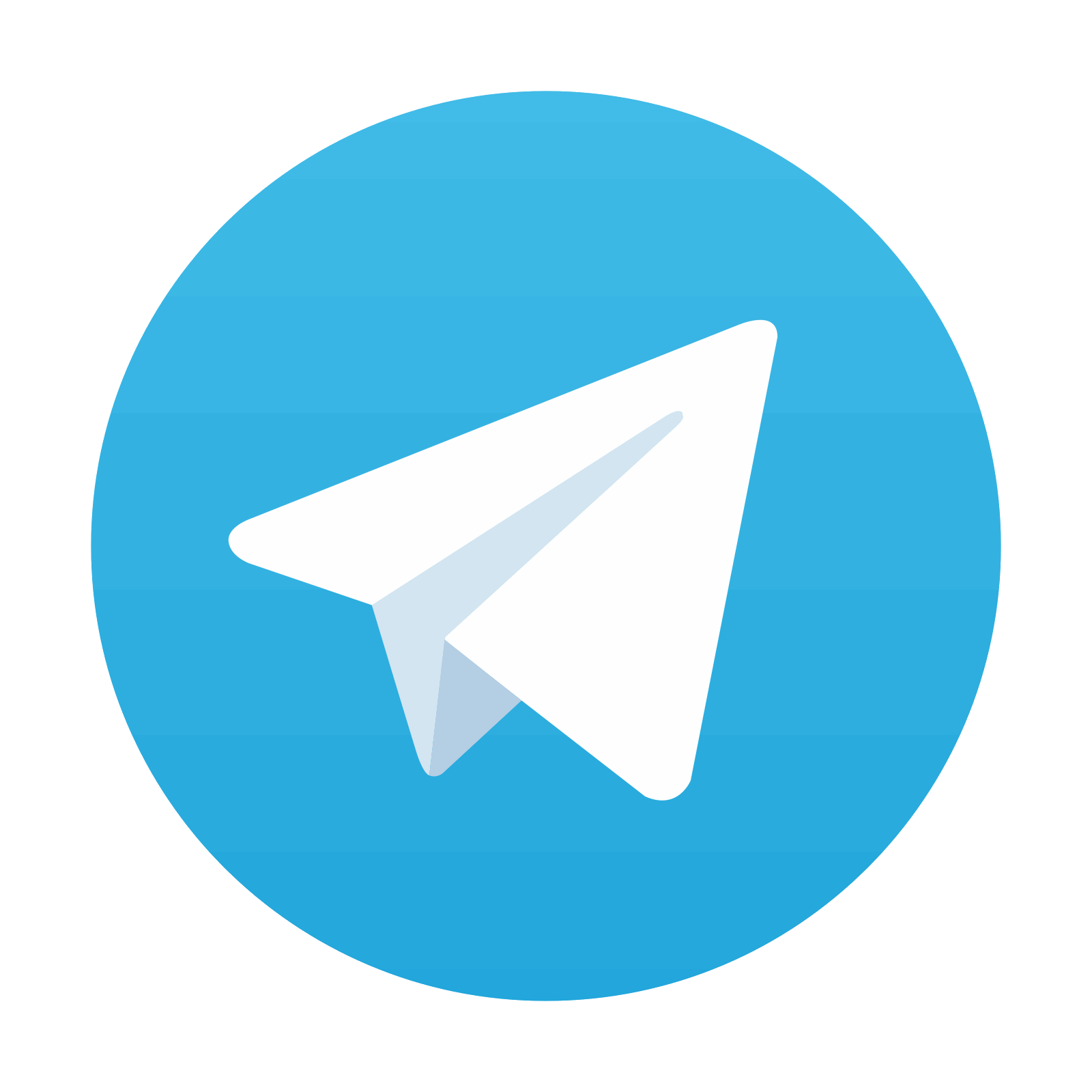
Stay updated, free articles. Join our Telegram channel

Full access? Get Clinical Tree
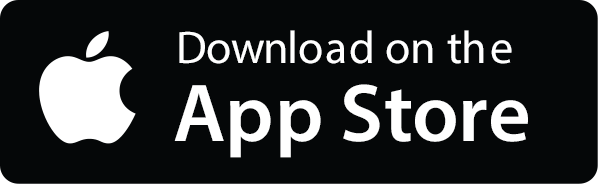
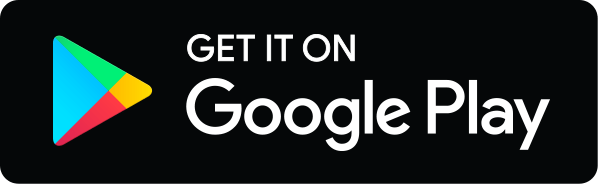