Fig. 35.1
Disease progression of prostate cancer from initial diagnosis until death. Local therapies such as surgery and radiation can control initial disease, and hormone-naïve tumors see clinical benefit from antiandrogen therapy. However, prognosis for advanced metastatic castrate-resistant prostate cancer is poor, and few options exist for treatment
In the past several years, immunotherapy has emerged as a promising part of the treatment armamentarium for a variety of solid tumors. In 2010, anti-CTLA-4 (ipilimumab, Bristol-Myers Squibb, Princeton, NJ) was approved by the US FDA for the treatment of late-stage melanoma after a pivotal randomized phase III trial showed that CTLA-4 blockade increased overall survival [6]. Anti-CTLA-4 treatment also showed activity in renal cell and non-small cell lung carcinoma, but it is not approved by regulatory agencies for either of those indications. More recently, agents that block the PD-1/PD-L1 axis were shown to prolong survival in patients with non-small cell lung cancer, melanoma, kidney cancer, head and neck cancer, and others—leading to a barrage of regulatory approvals [7]. Across multiple tumor types, objective responses from PD-1/PD-L1 blockade appear to be more durable than those associated with chemotherapy [8]. Additionally, there is one vaccine that is approved for use in a treatment setting for mCRPC—the active cellular therapy Sipuleucel-T (Dendreon, Seattle, WA) [9]. Another notable immunotherapy strategy in late-stage development is adoptive cellular therapy, most notably CAR T cells, which have shown remarkable activity in certain leukemias and lymphomas [10, 11]. T cells engineered to express tumor-specific T cell receptors (TCR) have shown clear activity in melanoma patients [12], although adoptive cellular therapy still faces many challenges in the field of solid tumors [13].
Despite these successes, immunotherapy for advanced prostate cancer still has many challenges to overcome, including lack of tumor immunogenicity as well as the major obstacle that prostate cancer is poorly infiltrated as compared to some of the more responsive tumor types for which agents have been approved (Fig. 35.2). These daunting properties of the prostate cancer tumor microenvironment (TME) may make it a difficult tumor to treat with immunotherapy, and treatments that show activity in other tumor types fail to elicit responses in mCRPC. In terms of anti-PD-1/PD-L1 directed therapies, one hypothesis for this lack of objective response in the clinic is the relative paucity of PD-L1 expression on prostate tumors [14], which may explain why blocking antibodies are generally ineffective as a monotherapy. One potential exception to this lack of immunogenicity is in the context of tumors with microsatellite instability (MSI). Across a wide variety of diseases, tumors with MSI exhibit a more robust response to immunotherapy [15]. Unfortunately, true MSI in mCRPC is quite rare, occurring in less than 5% of patients [16]. Combination immunotherapy with anti-CTLA-4 and anti-PD-1 is active in several tumor types [17, 18], and combination treatment was recently approved by the US FDA for the treatment of melanoma [19].
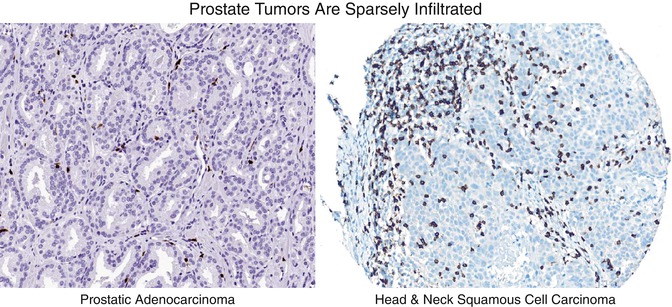
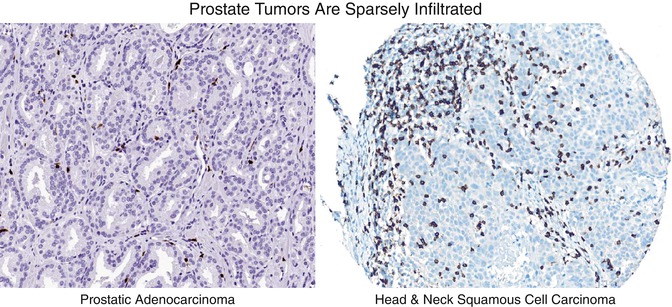
Fig. 35.2
CD8+ T cell infiltration patterns in prostate cancer tissue versus head and neck cancer. IHC staining for CD8 shows poor infiltration of prostate tumor by cytotoxic T Cells as compared to head and neck tumor
35.2 Immune Checkpoint Blockade
As highlighted above, immune checkpoint blockade showed impressive activity in several types of solid tumors. During a typical immune response, activated cytotoxic T cells upregulate PD-1, which binds to ligands such as PD-L1 and PD-L2 (Fig. 35.2) to inhibit T cell proliferation and effector function. This mechanism likely evolved to prevent uncontrolled systemic responses, which could lead to autoimmunity. However, many tumors have co-opted this pathway to evade immune recognition by upregulating immune checkpoint ligands, such as PD-L1 either on the tumor cells themselves or on the myeloid cells that infiltrate the tumor microenvironment. So, interfering with the PD-1/PD-L1 interaction can potentially enhance an antitumor response. One further consequence of this mechanism is that PD-L1 expression on tumor cells or on the immune cells infiltrating tumors could serve as a possible biomarker for tumors that would be more sensitive to PD-1/PD-L1 blockade. Extensive evaluation of this hypothesis across a number of trials resulted in sometimes conflicting results. For example, in the pivotal trial of PD-1 blockade in kidney cancer, the PD-L1 status of the tumor showed no association with response [20]. By contrast, the anti-PD-1 agent pembrolizumab (Merck, Kenilworth, NJ) is only US FDA approved for first-line therapy in non-small cell lung cancer but only in patients with PD-L1-positive tumors [21]. With a few exceptions [22], staining of mCRPC specimens has shown them to be mostly negative for PD-L1 [14, 23]. During the second (phase Ib) trial of anti-PD-1 (nivolumab, Bristol-Myers Squibb, Princeton, NJ), none of the 17 mCRPC patients enrolled showed an objective response, and neither of the two tumor specimens taken from that group was PD-L1 positive [23]. This is not particularly surprising, as prostate cancer is typically characterized by low levels of inflammation (Fig. 35.2), a lack of PD-L1 expression, and low mutational burden [24, 25], all of which may make it difficult to target with immunotherapy.
Similar to PD-1, CTLA-4 is another immune checkpoint molecule expressed by T cells upon activation, whose engagement inhibits proliferation and activity. It binds to its ligands, CD80/B7-1 and CD86/B7-2 (Fig. 35.3), with higher affinity than the T cell costimulatory molecule CD28 and thus is able to outcompete CD28 for binding. It is important for maintaining immune tolerance, as CTLA-4 knockout mice quickly succumb to lethal lymphocytic inflammation [26]. However, it also plays a role in immune evasion by tumors, and in early studies, tumor-bearing mice treated with anti-CTLA-4 showed a significant antitumor effect [27]. Two separate clinical trials in late-stage melanoma also showed positive results, with an increased median overall survival [28]. Interestingly, emerging data show that CTLA-4 is likely more highly expressed on tumor-infiltrating Treg than on CD8 T cells, suggesting the possibility that blockade exerts its antitumor effect through inhibiting the function of tumor-infiltrating T regulatory (Treg) [29], which serve to inhibit T cell responses.
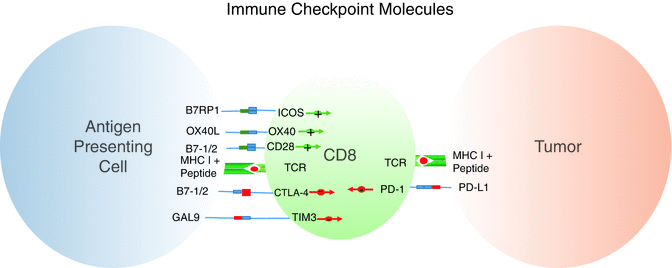
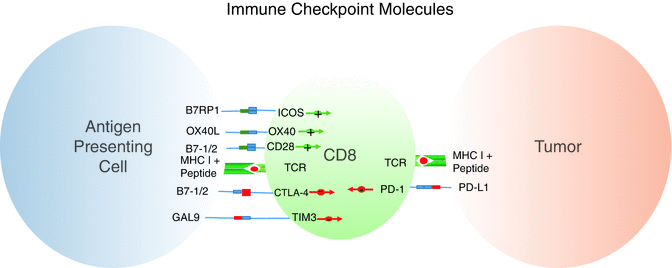
Fig. 35.3
Activating and inhibitory processes compete to mediate tumor response. Antigen-presenting cells can activate CD8 T cells and provide additional costimulation. Tumor cells upregulate inhibitory molecules to dampen CD8 activation and cytotoxic response. Chronic exposure to inhibitory ligands leads to CD8 exhaustion
Early studies of anti-CTLA-4 in mCRPC suggested some degree of activity, with approximately 10–15% of patients experiencing a drop in PSA, and a few objective responses [30]. These data, as well as the fact that few other therapies for advanced mCRPC were available at the time, provided enthusiasm for definitively testing anti-CTLA-4 in men with mCRPC. Two trials were launched, the first of which compared low-dose radiotherapy (RT) plus anti-CTLA-4 to placebo in men who progressed on or after docetaxel chemotherapy [31]. Although the trial failed to meet its primary endpoint of overall survival, there was a small increased in progression-free survival in the treatment arm. This trial was unique among prostate cancer immunotherapy trials, in that it did not exclude men with visceral metastases. This is important, because such patients have a poor overall prognosis [32]. Hypothesis-generating, retrospective analyses showed that patients with visceral metastases appeared to derive no benefit from immunotherapy, whereas men with no visceral metastases treated with anti-CTLA-4 had an OS approximately 4.1 months lower than those in the placebo arm (14.4 vs. 10.3 months) [31]. As above, visceral metastases have been correlated with a worse disease prognosis in mCRPC [32], although second-line hormonal therapies such as abiraterone are active in men with and without visceral metastases [33]. It is possible that metastasis to niches such as the liver fundamentally changes the antiapoptotic, angiogenic, and immunogenic properties of cells which make them more resistant to immunotherapies [33]. A second phase III trial enrolled men with earlier-stage mCRPC (pre-chemotherapy) and specifically excluded those with visceral metastases. Unfortunately, that trial also failed to reach its OS endpoint [34]. Taken together, the lack of responses in the late phase IB trial of anti-PD-1 and the two failed phase III trials of anti-CTLA-4 provides evidence that metastatic prostate cancer is a relatively nonimmunogenic tumor type and that either combination approaches or other agents may be required.
Two pieces of data suggest that later-stage mCRPC that progresses on enzalutamide might have unique immunological properties. The first of these was a case report of a patient progressing on enzalutamide who was treated with Sipuleucel-T and experienced an unusual complete response [35]. Perhaps more relevant are recent data showing that enzalutamide-resistant prostate cancer cell lines appear to upregulate PD-L1, suggesting that enzalutamide-resistant prostate cancer patients might respond to PD-1/PD-L1 blockade [36]. Based on those data, Graff et al. launched an interesting trial (NCT02312557) in which men with mCRPC who initially responded to enzalutamide and subsequently progressed were treated by adding the anti-PD-1 antibody pembrolizumab to ongoing enzalutamide. Early data on this trial were recently published and showed that three of the first ten patients had objective responses to pembrolizumab [37]. Additional observations included one patient with progression-free survival at up to 55 weeks, resolution of cancer-related pain, and serum PSA levels falling to <0.1 ng/mL. Interestingly, one of the responders also had microsatellite instability positive disease, an indication of defects in DNA mismatch repair (MMR). Previous findings showed that PD-1 blockade has widespread activity in patients with MMR deficiencies [38], possibly because of increased expression of neoantigens serving as targets for a wider repertoire of lymphocytes. Although the incidence of mismatch repair defects in prostate cancer is thought to be relatively low, one study found that in advanced prostate cancer cases, 7 out of 60 men had tumors with MMR defects and microsatellite instability [16]. Specifically, mutations in DNA repair genes MSH2 and MSH6 were found to be prevalent, possibly arising from gene rearrangements generated by androgen receptor itself [16]. Future clinical trials and treatment regimens will take into account the mutational status and nature of mutation of the patient’s tumor, with the notion that this could possibly serve as a predictive biomarker for PD-1/PD-L1 blockade-based immunotherapy.
Several phase II and III clinical trials of immune checkpoint blockade, either alone or in combinations, are currently underway (Table 35.1). One particularly notable trial is a phase III trial of the anti-PD-L1 antibody atezolizumab (Genentech, San Francisco, CA). In this trial, men progressing on first-line abiraterone acetate will be randomized 1:1 to treatment with the second-generation antiandrogen enzalutamide either alone or in combination with atezolizumab. The primary endpoint is overall survival. A second trial of note is a fairly large single-armed phase II trial of anti-PD-1 (pembrolizumab) in mCRPC patients previously treated with chemotherapy (Table 35.1), with a primary endpoint of safety and objective response rate. In a more exploratory approach, anti-PD-1 (pembrolizumab) is currently being combined with cryosurgery to induce the release of possible tumor antigens and activate a greater spectrum of CD8+ T cells in a phase II trial (Table 35.1).
Table 35.1
Immune checkpoint blockade trials in prostate cancer
Checkpoint inhibitor | Setting | In combination with… | Phase | NCT ID |
---|---|---|---|---|
Pembrolizumab | Enzalutamide progressors | Phase II | NCT02312557 | |
Pembrolizumab | Previous chemotherapy | Phase II | NCT02787005 | |
Pembrolizumab | Previous docetaxel, enzalutamide, or abiraterone | Olaparib, docetaxel + prednisone, or enzalutamide | Phase III | NCT02861573 |
Pembrolizumab | mCRPC | Cryosurgery | Phase II | NCT02489357 |
Pembrolizumab | mCRPC | ADXS31-142 (listeria-PSA) | Phase II | NCT02325557 |
Pembrolizumab | mCRPC | MVI-816 (PAP DNA Vac) | Phase II | NCT02499835 |
Pembrolizumab | Radiation and ADT | SD-101 (TLR9 agonist) | Phase II | NCT03007732 |
Atezolizumab | Androgen pathway inhibitor progressor | R-223-D | Phase I | NCT02814669 |
Atezolizumab | Docetaxel/cabazitaxel naïve | Sipuleucel-T | Phase I | NCT03024216 |
Atezolizumab | Abiraterone progressors, taxane ineligibility/progressors | Enzalutamide | Phase III | NCT03016312 |
Nivolumab | Mismatch repair-deficient tumors | Phase II | NCT03040791 | |
Nivolumab | AR-V7-expressing tumors | Ipilimumab | Phase II | NCT02601014 |
Nivolumab | mCRPC | Ipilimumab | Phase II | NCT02985957 |
Nivolumab | mCRPC | Prostvac and/or ipilimumab | Phase I/II | NCT02933255 |
Ipilimumab | Localized PC | Prostvac | Phase II | NCT02506114 |
As highlighted above, recent studies suggested the possibility that PD-1 and CTLA-4 might be expressed on different cell types in the tumor microenvironment, with CTLA-4 being predominantly expressed on Tregs and PD-1 being strongly expressed on CD8 T cells [39, 40]. Blockade of CTLA-4 inhibits suppressive tumor-infiltrating Treg function [41], while PD-1 blockade enhances CD8 functionality and may also prevent the induction of a tolerogenic program during antigen encounter [42]. These data support the concept that combined blockade of PD-1 and CTLA-4 could show synergistic efficacy in a tumor setting, as was the case in animal models [43, 44]. Clinical data on combined PD-1/CTLA-4 blockade were first reported in advanced melanoma [45] with 65% of patients exhibiting evidence of clinical activity, including a number of responses that occurred quite rapidly, i.e., within the first 8 weeks of treatment. The combination was eventually US FDA approved for melanoma and is currently being evaluated in the first-line setting in NSCLC [18] and in kidney cancer [46]. Interestingly, the phase III trial in melanoma showed that the combination therapy was effective even in patients with PD-L1-negative tumors [19], which is of particular interest in the context of prostate cancer, which typically expresses low levels of PD-L1 [14]. Based on these encouraging results, the Hopkins group recently completed enrollment of a small study of the combination in men with mCRPC who were biomarker selected for resistance to antiandrogens (Table 35.1). The primary endpoint of this trial is safety and tolerability, as well as the rate of objective responses. Additionally, checkpoint blockade can be combined with nonimmune agents. Some prostate tumors, especially those with mutations in BRCA1/BRCA2, show a dependence on poly-ADP-ribose polymerase (PARP) [47], and a phase I study is underway combining an anti-PD-L1 antibody (durvalumab, MedImmune, Gaithersburg, MD) with olaparib (AstraZeneca, Wilmington, DE), a PARP inhibitor (Table 35.1).
35.3 Cancer Vaccines
Unlike immune checkpoint blockade, which functions by down-modulating inhibitory immune signaling to augment an existing tumor response, vaccines seek to prime and induce an antitumor immune response. Vaccines function at the level of antigen-presenting cells, i.e., dendritic cells (DCs), which uptake and process antigens before presenting them to cytotoxic CD8 T cells along with costimulatory molecules that enhance the CD8 response. These T cell antigens are small peptide fragments presented in the context of MHC molecules, and when recognized by a cognate T cell receptor (TCR) on a CD8 T cell, activates the T cell. Activated CD8 T cells proliferate, traffic widely, and are capable of specifically lysing targets that express their cognate peptide/MHC ligand. Dendritic cells are the most potent antigen-presenting cells (APC) due to their ability not only to prime naïve T cells but also due to their ability to respond to innate immune signaling and thus orchestrate an immune response. So, in general a cancer vaccine consists of an adjuvant, designed to activate dendritic cells and prime them for presenting antigen and costimulation to T cells, as well as a tumor-specific peptide/protein against which the immune response is mounted [6]. Prostate cancer is an especially good candidate for vaccines due to its expression of fairly specific tumor antigens such as PAP, prostate-specific membrane antigen (PSMA), and prostate-specific antigen (PSA), all of which are expressed relatively more commonly in the prostate [48]. This restricted expression theoretically lowers the risk for unintended targeting of other tissues if T cells are robustly activated via vaccination. In addition, men with metastatic disease typically have undergone primary treatment with either radiation therapy or with surgery; thus the only source of prostate antigens in such men is likely to be the tumor itself.
The only US FDA-approved vaccine for the treatment of a solid tumor is Sipuleucel-T, a dendritic cell vaccine generated from patient-derived cells. To prepare this product, patient monocytes are isolated and co-cultured with a fusion protein consisting of PAP and GM-CSF, which induces the maturation of monocytes to dendritic cells, activates the dendritic cells, and provides PAP as a target antigen [49]. Upon reinfusion of these primed DCs into the patient, the antigen is presented on MHC I and II in order to potentially induce a cytotoxic response as well as aid in the development of a significant humoral effect. The Immunotherapy for Prostate Adenocarcinoma Treatment (IMPACT) study showed that Sipuleucel-T-treated patients derived a 4.1 month average OS benefit, with an OS of 25.8 months for treated patients versus 21.7 months for those receiving placebo. However, only 2.6% of treated patients had a PSA decrease of more than 50% [9]. In these patients, not only was there a significant increase in serum antibodies against the primary antigen, PAP, but also antibodies against “secondary antigens” such as LGALS3 and ECE1 which are known to be expressed in prostate tumors [50]. This phenomenon, known as “antigen spread,” likely occurs when immune-mediated attack of cancer cells leads to the release of additional antigens in a pro-immunogenic context [51].
Although Sipuleucel-T was efficacious in phase III trials, the only other vaccine to complete phase III testing in prostate cancer was less encouraging. This reagent, GVAX prostate, is a whole-cell vaccine which consists of two tumor cell lines—PC3 and LnCaP—transduced with a recombinant viral vector to secrete GM-CSF. A randomized phase III trial, comparing GVAX prostate to chemotherapy in men with mCPRC, completed enrollment of approximately 600 patients in 2006. It should be noted that this trial, unlike the trials of Sipuleucel-T or the ongoing phase III trial of Prostvac-VF (see below), used an active comparator arm—docetaxel chemotherapy—which has clear clinical benefit in mCRPC [52]. The phase III trial for GVAX prostate in mCRPC was terminated early due to a futility analysis showing less than a 30% chance that the target endpoint would be met [53]. Nonetheless, GVAX is now being tested in combination with other treatments, including in a neoadjuvant setting in combination with hormonal therapy in phase II (Table 35.2).
Table 35.2
Selected prostate cancer vaccines in clinical trials
Name | Type | Antigen target(s) | Phase | NCT ID |
---|---|---|---|---|
Sipuleucel-T | Cell-based | PAP | FDA approved | |
Sipuleucel-T + radium-223 | Cell-based | PAP | Phase II | NCT02463799 |
Sipuleucel-T + early/late ipilimumab | Cell-based | PAP and CTLA-4 | Phase II | NCT01804465 |
GVAX prostate + cyclophosphamide | Cell-based | PC3 and LNCaP antigens | Phase II | NCT01696877 |
Prostvac | Viral | PSA | Phase III | NCT02649439 |
Prostvac + enzalutamide | Viral | PSA | Phase II | NCT01867333 |
ChAdOx1.5T4-MVA.5T4 | Viral/protein | 5T4 | Phase I | NCT02390063 |
MVI-816 | DNA | PAP | Phase II | NCT00849121 |
MVI-118 | DNA | Androgen receptor (AR) | Phase I | NCT02411786 |
INO-5150 | DNA | PSA + PSMA | Phase I | NCT02514213 |
ProstAtak | Viral | Oncolytic adenovirus | Phase III | NCT01436968 |
CureVac | mRNA | PSA, PSMA, PSCA, and STEAP | Phase II | NCT00831467 |
ADU-741 | Bacterial | PSMA, PAP, NKX3.1, and SSX-2 | Phase I | NCT02625857 |
ADXS-PSA | Bacterial | PSA | Phase I/II | NCT02325557 |
Another vaccine being developed for prostate cancer is Prostvac-VF (Bavarian Nordic, Morrisville, NC), a two-part viral vaccine consisting of a prime (Prostvac-V) and boost (Prostvac-F), targeting the antigen PSA (Table 35.2). In addition, the vaccine includes TRICOM™, which is a triad of costimulatory molecules (B7.1, ICAM-1, and LFA-3), designed to increase APC presentation of the target antigen. A phase II trial with Prostvac-VF did not meet the prespecified study endpoint of enhancing progression-free survival (PFS), but a post hoc analysis showed that the vaccine appeared to improve overall survival by 8.5 months, from 16.6 to 25.1 months, at a 3-year follow-up [54]. A phase III trial of Prostvac with GM-CSF in mCRPC patients is currently underway (Table 35.2). This survival trial randomized 1200 men with chemotherapy-naïve mCRPC 1:1:1 to either placebo, Prostvac-VF plus intradermal GM-CSF, or Prostvac-VF plus placebo (NCT01322490). Patients with visceral disease were excluded. Enrollment has completed, but final results are not yet available. A number of trials combining Prostvac with anti-CTLA-4 (as well as other agents) are currently ongoing, and preliminary results indicate a decline in PSA levels in 14 out of 30 patients [55].
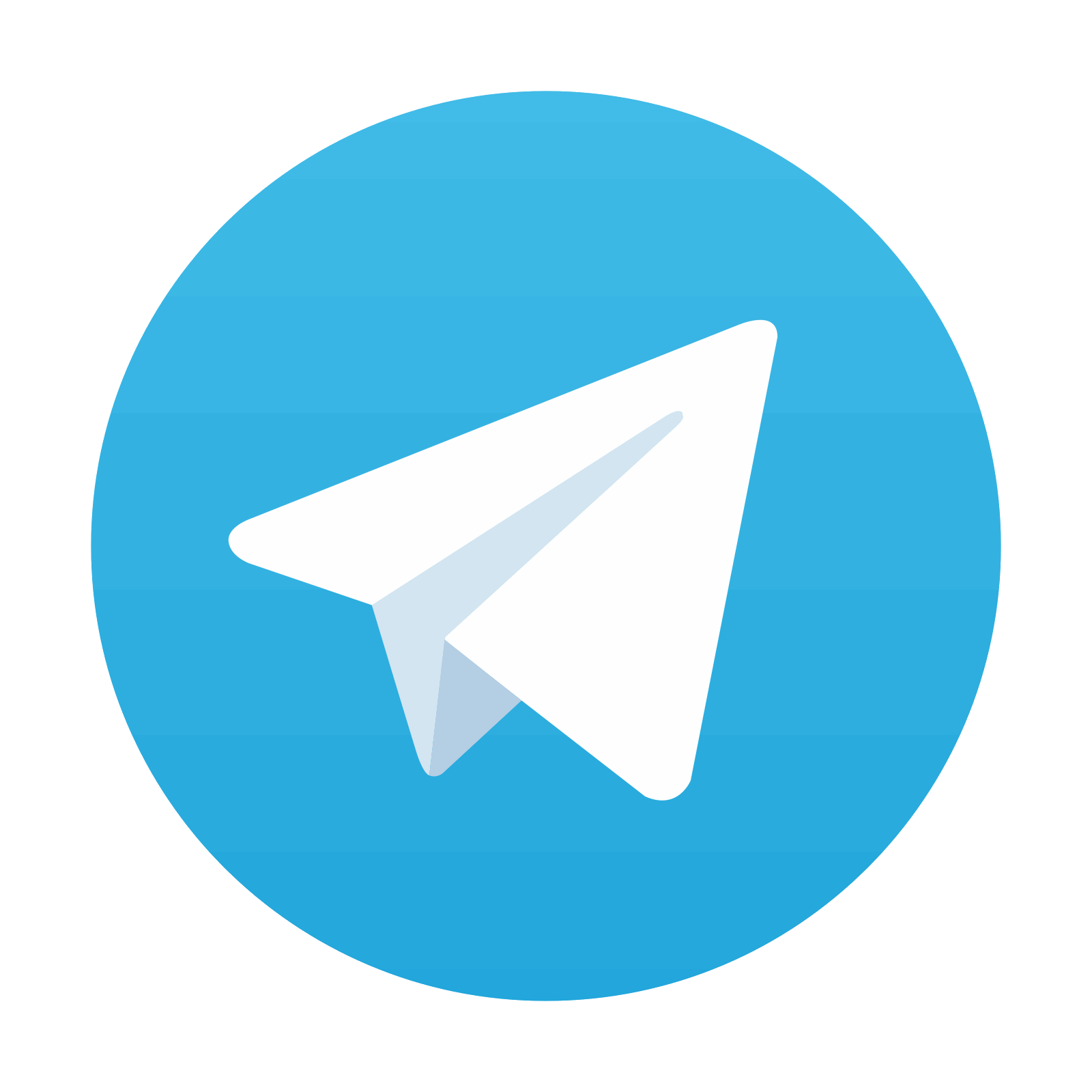
Stay updated, free articles. Join our Telegram channel

Full access? Get Clinical Tree
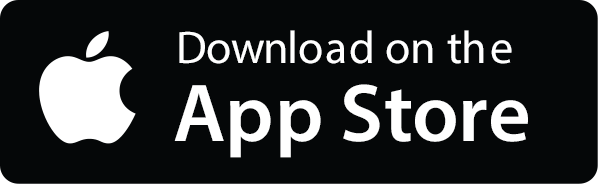
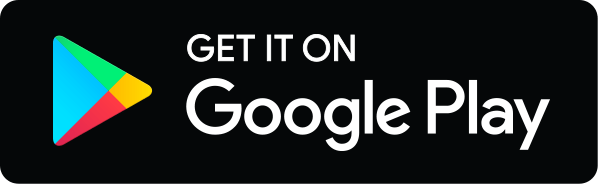