Fig. 24.1
Pathogenesis of graft-versus-host disease (GVHD) can be divided into three phases. In the first phase, the host is conditioned by myeloablative treatment. This leads to tissue damage with the release of proinflammatory cytokines and the danger signals LPS and ATP. The stem cell transplant induces phase II, when these molecules activate the transferred allogeneic donor cells. In phase III, donor NK cells, macrophages, and neutrophils directly attack host tissues by triggering apoptosis and releasing additional cytokines which leads to a cytokine storm. Both host and donor APC in their turn activate T cells which then exert their cytotoxic activity causing the typical symptoms of GVHD. All three phases are targeted by approaches to treat GVHD. Blocking antibodies against cytokines, co-stimulation molecules, and activation markers interfere with specific activation pathways of GVHD. Cell therapy with Treg cells, mesenchymal stem cells (MSC), and NK cells suppresses additional mediators
24.3.1 Phase I: Conditioning
The first phase of GVHD started already before the actual HSCT with the conditioning regime. This treatment depletes the patient’s own bone marrow cells to allow engraftment of the donor’s stem cells and prevent their rejection. It also aims at clearing any remaining pathological cells in the patient. The myeloablation is either done by total-body irradiation or high-dose chemotherapy. However, neither strategy is targeted and causes also injury to other tissues, particularly the gut mucosa, skin, and liver. The damaged cells in response secrete a massive amount of cytokines, especially interleukin (IL)-1, IL-6, interferon (IFN)-γ, and tumor necrosis factor (TNF)-α [11].
Several polymorphisms for genes of these cytokines have been associated with an increased risk for GVHD. While polymorphisms for TNF-α, IFN-γ, IL-6, IL-10, and a natural occurring IL-1 receptor antagonist were associated with severity of acute GVHD [12–15], IL-6 also correlates with the incidence of chronic GVHD [16–18].
Not only cytokines but also other danger signals contribute to the proinflammatory environment. One molecule that is released from dying cells and has been implicated in the pathogenesis of GVHD is ATP [19]. It can itself induce the secretion of the proinflammatory cytokines IFN-γ, TNF-α, and IL-6 and activate antigen-presenting cells (APC). Also the ATP receptor P2X7 is upregulated during GVHD, leaving cells more susceptible to the effects of ATP and creating a positive feedback loop. Thus, blocking of P2X7 as well as reduction of ATP concentration can improve GVHD symptoms. Even increased numbers of regulatory T (Treg) cells, which are known to control GVHD, are detected. Notably, Treg cells are also one of the main populations expressing the ATP-hydrolyzing enzyme CD39 on their surface [20] as they are particularly sensitive to ATP [21].
In the intestine, the tissue damage leads to the release of microbial lipopolysaccharides (LPS) which is recognized by Toll-like receptors (TLR) on APC. Polymorphisms in genes for several of these TLR, namely, TLR4, TLR7, and TLR9, were described to influence the incidence and severity of GVHD [22–24]. Furthermore, flagellin, an agonist for TLR5, was able to reduce GVHD in a mouse model [25]. Although the TLR/APC axis undoubtedly plays an important role in the onset of GVHD, it also develops without intact TLR signaling [26].
The presence this plethora of cytokines and danger signals present at these sites of acute inflammation leads to the activation of APC. These comprise dendritic cells (DC), monocytes, macrophages, and B cells which present antigens to T lymphocytes and activate these. APC and other local cells upregulate the MHC (major histocompatibility complex) and co-stimulatory and adhesion molecules [27] and thereby set the stage for the next phase of GVHD.
24.3.2 Phase II: Activation
The second phase is initiated when donor stem cells are transferred into this proinflammatory setting. They are attracted by the presence of cytokines, chemokines, and adhesion molecules at the sites of tissue injury. Here they come into contact with the activated host APC that present both MHC molecules and self-antigens. Any self-antigen derived from a protein that is different between donor and recipient can give rise to minor histocompatibility antigens (MiHA). Allogeneic donor cells recognize disparities in MHC for HLA mismatched donor/patient pairs or in MiHA with their T-cell receptor (TCR) [28]. In addition, also non-hematopoietic host tissue cells [29, 30] and donor APC [31, 32] can be involved in the presentation of host antigens. As all of these cell types are involved in the presentation of alloantigens, targeting only one cell type is not sufficient in abrogating GVHD [33, 34].
In addition to TCR engagement, alloreactive T cells require co-stimulatory signals for their activation; these are also provided by activated APC. The main pathway for this co-stimulation involves the engagement of CD28 on T lymphocytes by its B7 ligands CD80 and CD86 on APC [35]. Also CD40 and its ligand CD40L play a role in providing positive co-stimulation for T cells [36].
24.3.3 Phase III: Effector Phase
The clinical symptoms of acute GVHD are mainly caused by the action of T cells. It was shown that only the naïve pool of donor T cells is responsible for the development of GVHD but not transplanted effector and central memory T cells [39]. Other important cell subsets responsible for the tissue damage in the effector phase of GVHD are natural killer (NK) cells, macrophages, and neutrophils [40]. Also B cells were reported to play a role [41].
Alloreactive CD8+ T cells use two main mechanisms for their cytotoxic action, both of which induce the activation of the caspase cascade in target cells by direct cell contact. One pathway induces the release of perforin and granzymes which trigger cell lysis [42]. The other mechanism requires engagement of the death receptor, Fas, with the Fas ligand (FasL) leading to apoptosis [43].
Tissue damage is also induced indirectly by the cytokines present in abundance from the preconditioning (TNF-α, IFN-γ and IL-1).
These cytokines themselves trigger the release of a surge of additional cytokines from allogeneic donor cells in a self-amplifying cycle that can lead to a cytokine storm [44] with deleterious effects on the patient.
TNF-α is the key cytokine involved in all three phases of the pathogenesis of GVHD [45]. It was reported as an independent predictor of GVHD as it correlates with its severity, time of onset, and mortality when measured with the help of the surrogate marker TNF receptor 1 (TNFR1). TNFR1 binds TNF-α and is shed into the plasma where it can easily be measured [46–49]. Another member of the tumor necrosis factor receptor family, CD30, has been described as a marker for GVHD. Higher levels of CD30 were found both in plasma and on CD8+ T cells of patients with acute GVHD [50]. A more comprehensive combination of the four biomarkers IL-8, hepatocyte growth factor, TNFR1, and IL-2 receptor-α was suggested to predict the occurrence of GVHD [51].
24.4 Natural Control of GVHD
Natural suppression of GVHD is conferred by Treg cells. These cells regulate both innate and adaptive immune responses and confer peripheral tolerance by suppressing activated T cells [52, 53]. Their modes of action include a variety of mechanisms involving both soluble factors and direct cell-cell contact [54, 55]. In mouse models, depletion of Treg cells exacerbated GVHD, while infusion of these cells improved its outcome [56, 57]. However, in the overwhelming inflammation during GVHD, the balance of the immune system is grossly shifted toward the proinflammatory response and apparently leaves Treg cells insufficient. Nevertheless, Treg cells have been associated with the outcome of GVHD [57, 58]. Even specific markers in the transcriptome of Treg cells were able to predict both acute and chronic GVHD, among these were activation markers (PIP5Kγ, FAS, CD44, CD69), cyclins, and the transcription factor Eos which is important for the suppressive capacity of Treg cells [59].
24.5 Graft-Versus-Tumor Effect
The undesirable graft-versus-host syndrome is closely intertwined with the graft-versus-tumor (GVT) response, which is part of the curative effect of stem cell transplantation especially in patients with malignancies. T cells from the graft not only recognize alloantigens but also tumor antigens and are thus able to act against leukemia or tumor cells that escaped the conditioning regime. Also NK cells [60–62] and NKT cells were implicated in the GVT activity [63]. The greater the MHC mismatch between donor and patient, the stronger the GVT response is. This explains the advantage of allogeneic over autologous HSCT and the higher relapse rate of patients receiving HSCT from a related donor [1]. Especially after non-myeloablative pretreatment of the patient, GVT is indispensable to clear any remaining malignant cell. The strong general immunosuppression as well as T-cell depletion to prevent GVHD also inhibits GVT [64]. Thus, the challenge is to find a balance between the negative impact of GVHD and the positive effects of GVT. The incidence of GVHD is considered as an indication of an ongoing GVT activity and was shown to correlate with a reduced risk of relapse [65, 66]. An option to treat cancer relapse after HSCT is donor lymphocyte infusion which has a high risk of GVHD but also a highly effective GVT response [67].
Approaches to separate GVHD from GVT are difficult as both share similar mechanisms. However, attempts are being made to interfere with differences in the organ-specific proinflammatory environment, specific cells involved, and selective migration of effector cells to the target tissues [68].
24.6 Prevention of GVHD
Without any preventive treatment, acute GVHD would occur in practically every patient receiving allogeneic HSCT. Even with preemptive general immunosuppression, acute GVHD is still a major complication after HSCT. The standard treatment includes corticosteroids, cyclosporine A, and methotrexate (MTX). The combination of these has proven to be most effective [2]. Most novel approaches for the treatment of GVHD discussed below are also tested for its prevention.
A non-pharmacological approach to prevent the occurrence of GVHD is depletion of T cells, either ex vivo from the graft or in vivo. This depletion can be achieved by different methods, like anti-thymocyte globulin (ATG) or monoclonal antibodies (mAbs) directed against CD4, CD5, CD8, and/or CD52 (Campath/alemtuzumab) [69, 70]. Although depletion of lymphocytes was shown to reduce GVHD, it also increases the risk of graft rejection by the recipient’s T cells and of recurrence of the underlying disease as also GVT responses are diminished. It needs to be shown whether the attempts to selectively deplete alloreactive T cells can provide a tool to reduce the incidence and severity of GVHD.
24.7 Treatment of Acute GVHD
Even with these comprehensive measures of prevention, between 10 and 50 % of patients still develop forms of GVHD severe enough to require additional treatment. The first-line therapy remains administration of steroids, in particular methylprednisolone. However, the high doses necessary to abrogate the ongoing immune reaction lead to a generalized immunosuppression with the risk of opportunistic infections [2].
Other routine medications like the calcineurin inhibitor cyclosporine A inhibit T-cell activation and block IL-2 transcription [71]. The macrolide antibiotic tacrolimus or FK-506 also interferes with IL-2 production [72, 73] but appears to have less toxic side effects [74]. A different pathway is targeted by mycophenolate mofetil, which inhibits activation and proliferation of lymphocytes by blocking their purine synthesis [75]. These standard drugs, even in combination, are not sufficient for all patients and have serious side effects in addition to the immunosuppression [76–78]. Often renal and other toxicities limit their use.
Another approach is the depletion of T lymphocytes by the polyclonal anti-thymocyte globulin (ATG). A meta-analysis of six prospective randomized controlled trials found that ATG was able to lower incidence of grade II–IV GVHD with a stronger effect seen for rabbit compared to equine ATG but no impact on overall GVHD, survival, or relapse [79]. Higher doses of ATG can further decrease incidence of GVHD, but with a higher risk of serious infections [80]. A more recent study with a different ATG preparation could confirm the reduction in GVHD and reported a reduction in tumor relapse and infections [81]. Also a reduction of chronic GVHD was reported [82].
An interesting therapeutic modality is the extracorporeal photochemotherapy (ECP), a combination of a photosensitizing drug with exposure to UVA light. This has been demonstrated to inactivate lymphocytes although the mechanism still needs to be elucidated. However, in several clinical trials, symptoms of both acute and chronic GVHD were improved without negatively affecting GVT or relapse incidence [83, 84].
Also statins were shown to have an immunomodulatory effect by reducing cytokine secretion and T-cell activation [85]. In a mouse model, they reduced GVHD incidence [86]. A correlation study in patients could attribute a decreased risk for acute GVHD to the use of statins by the donor [87]. The risk for chronic GVHD was diminished if the recipient took statins [88].
A novel mechanism is targeted by the proteasome inhibitor bortezomib which is approved for treatment of myeloma. In a mouse model, early administration promoted the apoptosis of alloreactive T cells and thus prevented acute GVHD [89]. These results could be corroborated in a small clinical trial which also suggested an improvement of immune reconstitution [90]. However, timing is crucial as delayed and continued administration enhanced GVT but also aggravated GVHD symptoms [89].
24.8 Targeted Approaches
GVHD is well known as a multifactorial process with a variety of players involved, and most of these have been targeted in mouse models. However, not all could be transferred into clinical applications. Therefore, this chapter will focus on approaches for which clinical trials have been reported.
24.8.1 Targeting Cytokines
One approach is targeting the cytokines involved in the pathogenesis of GVHD. Best studied is TNF-α, a cytokine implicated in all three stages of GVHD. Antibodies against TNF-α or other neutralizing reagents are used routinely to treat inflammatory autoimmune disease. One of them, etanercept, is a TNF-α receptor 2 fusion protein that competes for binding with TNF-α. In combination with steroids, it elicited good response rates in two studies without increased incidence of infections or relapse [91, 92]. The neutralizing TNF-α antibody, infliximab, showed efficacy in several studies, although this was accompanied by a high incidence of infections [93–97]. This can be attributed to the ability of infliximab to additionally deplete monocytes and macrophages that present membrane bound TNF-α [98]. However, it needs to be noted that none of the antibodies was superior to steroids alone [99].
Another critical cytokine in GVHD is IL-2. Its receptor α-chain, CD25, is upregulated on activated CD4+ T cells. IL-2 is indispensable for the survival and proliferation of these cells. Therefore, CD25 is an interesting molecule to specifically target effector T cells. A monoclonal CD25 antibody, daclizumab, proved to be efficient in first clinical trials [100, 101]. However, a larger multicenter trial was halted due to lower survival in the daclizumab arm [102]. This could be due to the fact that Treg cells constitutively express high CD25 levels and could be preferentially depleted by the antibody. Treg cells are able to control GVHD by conferring tolerance after HSCT [103], and the depletion of beneficial Treg cells might outweigh the positive effects of depletion of effector T cells.
The receptor for IL-6 has been targeted by the mAb tocilizumab and could improve GVHD by shifting the balance of the immune response from proinflammatory effector cells toward the suppressive Treg cells [104]. The first clinical studies in patients with steroid refractory GVHD have promising results [105].
24.8.2 Targeting Co-stimulation
Specificity for the allogeneic responses in GVHD can be achieved by interfering with the activation of effector cells. Apart from engagement of the T-cell receptor by alloantigens, co-stimulation is essential for T cells to become activated and to prevent anergy and apoptosis.
The main co-stimulatory molecules expressed on T cells are the activating CD28 and its inhibitory counterpart CTLA4 (cytotoxic T-lymphocyte antigen 4). Their B7 ligands, CD80 and CD86, are provided by APC. But blocking the CD28-B7 axis affects not only the co-stimulation but also the inhibition pathway affecting Treg cells and thus exacerbating GVHD. Instead, one trial targeted CTLA4-mediated inhibition in a small group of patients and showed low incidence of GVHD. However, the procedure requires coculturing of donor and patient cells in the presence of a CTLA4-immunoglobulin fusion protein to induce tolerance of alloreactive donor cells and thus is not feasible for a larger cohort of patients [106]. Abatacept, a CTLA4-immunoglobulin fusion protein that acts in vivo, has shown efficacy in autoimmune disease [107] but has yet to be tested in GVHD.
The second important co-stimulatory pathway CD40/CD40L can be blocked by an antibody directed against CD40L. This was able to reduce GVHD and has a beneficial effect on Treg cells [108]. Unfortunately, clinical studies with a CD40L antibody had to be halted due to serious thrombotic side effects [109].
24.8.3 Targeting Cell Subsets
24.8.3.1 B Cells
The role of B cells in the pathogenesis of GVHD is not yet fully understood. They play an important role not only in the humoral immune response but also act as antigen-presenting cells and produce cytokines, thereby modulating GVHD [41]. Treatment of B-cell malignancies, one indication for HSCT, includes depletion of B cells with the mAb rituximab. In one study, high doses of rituximab given before HSCT were correlated with a reduced incidence of acute GVHD [110]. However, these findings could not be confirmed by all studies [111–114]. Also the effect of rituximab on chronic GVHD is controversial as two studies showed a decrease in the incidence of chronic GVHD [111], while one group even reported an increase. However, this was blamed on additional donor lymphocytes given to a subset of these patients [112, 115]. In summary, it seems that B-cell depletion with high doses of rituximab prior to HSCT is effective and does not affect engraftment, although it delays B-cell recovery [41].
24.8.3.2 NK Cells
A novel strategy to treat GVHD is the adoptive transfer of immune cells. Natural killer (NK) cells are one of the main effectors of GVHD and GVT. Therefore, they were successfully used in clinical trials to treat patients suffering from cancer relapse [116–118]. Surprisingly, in addition to promoting GVT, they were also able to abrogate GVHD [119, 120] and improved engraftment [62] by killing recipient APC which are implicated in the induction of GVHD [121]. The beneficial effect of NK cells is most profound in an unrelated donor/recipient setting [122].
24.8.3.3 Mesenchymal Stem Cells
The most straightforward approach of cell therapy is the adoptive transfer of inhibitory cells that are able to suppress the ongoing inflammatory process. One subset suggested for this strategy is mesenchymal stem cells (MSC). This immunomodulatory cell type can induce a tolerogenic phenotype in other immune cells [123, 124] and increase Treg numbers [125]. As they act in an MHC-independent manner and do not induce alloreactive responses, they were considered an ideal target for cell therapy [126]. MSC could indeed inhibit GVHD in one mouse model [127]. But although another model could confirm the suppressive function of MSC, no effect on GVHD was observed [128]. A canine GVHD model was also unable to detect any benefit of treatment with MSC [129]. Several small clinical trials confirmed that MSC can improve GVHD [130–132] with pediatric patients in general responding better to the treatment. However, a large phase III trial failed to show any benefit of MSC transfer in GVHD patients. Further studies need to demonstrate whether optimizing the dosage and timing of treatment with MSC can provide more reliable data [133].
24.8.3.4 Treg Cells
Treg cells are the main suppressors of the immune system and have been implicated in the control of GVHD. Transfer of Treg cells can rescue GVHD while maintaining the GVT effect [56, 134, 135]. The main problem for employing Treg cells for clinical application is that their only lineage-specific marker, the intracellular transcription factor FoxP3 [136], cannot be used for isolation of live cells. Thus, Treg cells have to be selected by surrogate surface markers. First studies used expression of CD25 for enrichment [137]. Although Treg cells express high levels of CD25, also activated T-effector cells upregulate CD25 and are thus indistinguishable from their regulatory counterparts. Nevertheless, the first phase I clinical trial was performed with cells from apheresis blood that were positively selected for CD25 after depletion of CD19+ B cells. This study with nine patients confirmed that adoptive transfer of these Treg cells is safe and feasible [138]. Another group used a similar approach to isolated Treg cells. These cells were administered as sole prevention measure against GVHD in 28 patients with high-risk malignancies who had received HLA-haploidentical HSCT. In these patients, a reduction in GVHD and CMV reactivation without loss of GVT was reported [139].
Another approach is the isolation of Treg cells not from adults but from umbilical cord blood. As those T cells are mainly in an antigen inexperienced naïve state, the majority remains CD25 negative [140]. Thus, selection of CD25-positive cells yields a relatively pure population of Treg cells. The drawback of the usage of cord blood is the low number of cells available in one unit. Therefore, the isolated cells were expanded to obtain sufficient numbers. Transfer of these cord blood-derived Treg cells into patients who had previously received a transplant of cord blood stem cells resulted in a reduction in GVHD incidence [141]. However, the low yield of Treg cells from umbilical cord blood and the need for cell expansion make the application for a larger patient cohort challenging.
The report that the IL-7 receptor, CD127, is expressed on all effector T cells but Treg cells lack its expression [142] suggested a different strategy for isolation. Depletion of CD127-expressing cells followed by positive selection of CD25 cells yields functionally suppressive Treg cells. In a case study, these cells were expanded and transferred into one patient with acute and one with chronic GVHD. In both, symptoms of GVHD improved at least transiently [143].
Combination with a second marker that is selectively absent on Treg cells, namely, CD49d, the α-chain of the integrin VLA-4, allows the isolation of untouched Treg cells. Single-step depletion of all CD127- and CD49d-expressing cells yields highly pure functional Treg cells [134]. A trial using these cells is in preparation.
Apart from adoptive transfer of Treg cells, several attempts have been made to target these cells in vivo. The cytokine IL-2 is indispensible for the function and survival of these cells, and they express high levels of its receptor CD25. This distinguishes them from CD4 effector cells which only after activation upregulate CD25. However, they require much higher concentrations of IL-2 than Treg cells. To exploit this difference, in two human trials, patients were treated with low-dose IL-2. Both studies detected increased numbers of Treg cells [144], and in one of them, symptoms of chronic GVHD were alleviated [145].
Treg and T-effector cells also use different signaling pathways. Sirolimus or rapamycin is an immunosuppressant that blocks the mammalian target of rapamycin (mTOR) and thereby cytokine secretion of T cells. Treg cells instead signal via STAT3 and STAT5 after activation and are spared from the suppressive effect of sirolimus. Some reports even find a preferential expansion of Treg cells in its presence [146, 147]. Clinical trials have confirmed the efficacy of sirolimus to prevent GVHD although toxicities limit its usage [148, 149].
A combination of sirolimus and IL-2 was reported as particularly efficient in promoting Treg cells by enhancing proliferation of natural Treg cells and conversion of induced Treg cells in a mouse model [150], but clinical data are still pending.
24.9 Concluding Remarks
Hematopoietic stem cell transplantation is often the last resort for patients with malignancies although the long-term outcome is still disappointing. One major complication is GVHD, a fulminant inflammatory response that is difficult to treat with conventional immunosuppression. New approaches target the different pathways involved in GVHD. Results of first clinical trials are promising, but no therapy is efficient for all patients. Heterogeneous patient cohorts and concomitant treatments make it difficult to compare results from different studies. The networks involved in the pathogenesis of GVHD are complimentary, and it is unlikely that targeting only one pathway will be sufficient. It is also possible that certain pathways are more important in one patient group than in others. In addition, genetic differences contribute to this heterogeneity and might be responsible for discrepancies observed in many trials. A more thorough selection of patients for specific therapies might give clearer results in the future. It might also prove helpful to identify relevant pathways to provide a more tailored therapy for individual patients which would not only improve efficacy of the therapy but also help to reduce side effects which are a huge problem with current medications. An additional challenge is to specifically prevent GVHD without affecting general immunity and the GVT effect. Eventually, combination of different therapeutic strategies and more individualized treatment of patients could improve the outcome and therefore boost the success story of HSCT.
References
1.
Pasquini MC, Wang Z. Current use and outcome of hematopoietic stem cell transplantation: CIBMTR Summary Slides. 2012. http://www.cibmtr.org.
2.
Goker H, Haznedaroglu IC, Chao NJ. Acute graft-vs-host disease: pathobiology and management. Exp Hematol. 2001;29(3):259–77.PubMed
4.
Inamoto Y, Flowers ME. Treatment of chronic graft-versus-host disease in 2011. Curr Opin Hematol. 2011;18(6):414–20.PubMedCentralPubMed
5.
Soderberg C, Larsson S, Rozell BL, Sumitran-Karuppan S, Ljungman P, Moller E. Cytomegalovirus-induced CD13-specific autoimmunity–a possible cause of chronic graft-vs-host disease. Transplantation. 1996;61(4):600–9.PubMed
6.
Patriarca F, Skert C, Sperotto A, Zaja F, Falleti E, Mestroni R, et al. The development of autoantibodies after allogeneic stem cell transplantation is related with chronic graft-vs-host disease and immune recovery. Exp Hematol. 2006;34(3):389–96.PubMed
7.
Lee SJ, Vogelsang G, Flowers ME. Chronic graft-versus-host disease. Biol Blood Marrow Transplant. 2003;9(4):215–33.PubMed
8.
Jacobsohn DA, Vogelsang GB. Acute graft versus host disease. Orphanet J Rare Dis. 2007;2:35.PubMedCentralPubMed
10.
Ferrara JL, Cooke KR, Pan L, Krenger W. The immunopathophysiology of acute graft-versus-host-disease. Stem Cells. 1996;14(5):473–89.PubMed
11.
Ferrara JL, Levy R, Chao NJ. Pathophysiologic mechanisms of acute graft-vs.-host disease. Biol Blood Marrow Transplant. 1999;5(6):347–56.PubMed
12.
Cavet J, Middleton PG, Segall M, Noreen H, Davies SM, Dickinson AM. Recipient tumor necrosis factor-alpha and interleukin-10 gene polymorphisms associate with early mortality and acute graft-versus-host disease severity in HLA-matched sibling bone marrow transplants. Blood. 1999;94(11):3941–6.PubMed
13.
Middleton PG, Taylor PR, Jackson G, Proctor SJ, Dickinson AM. Cytokine gene polymorphisms associating with severe acute graft-versus-host disease in HLA-identical sibling transplants. Blood. 1998;92(10):3943–8.PubMed
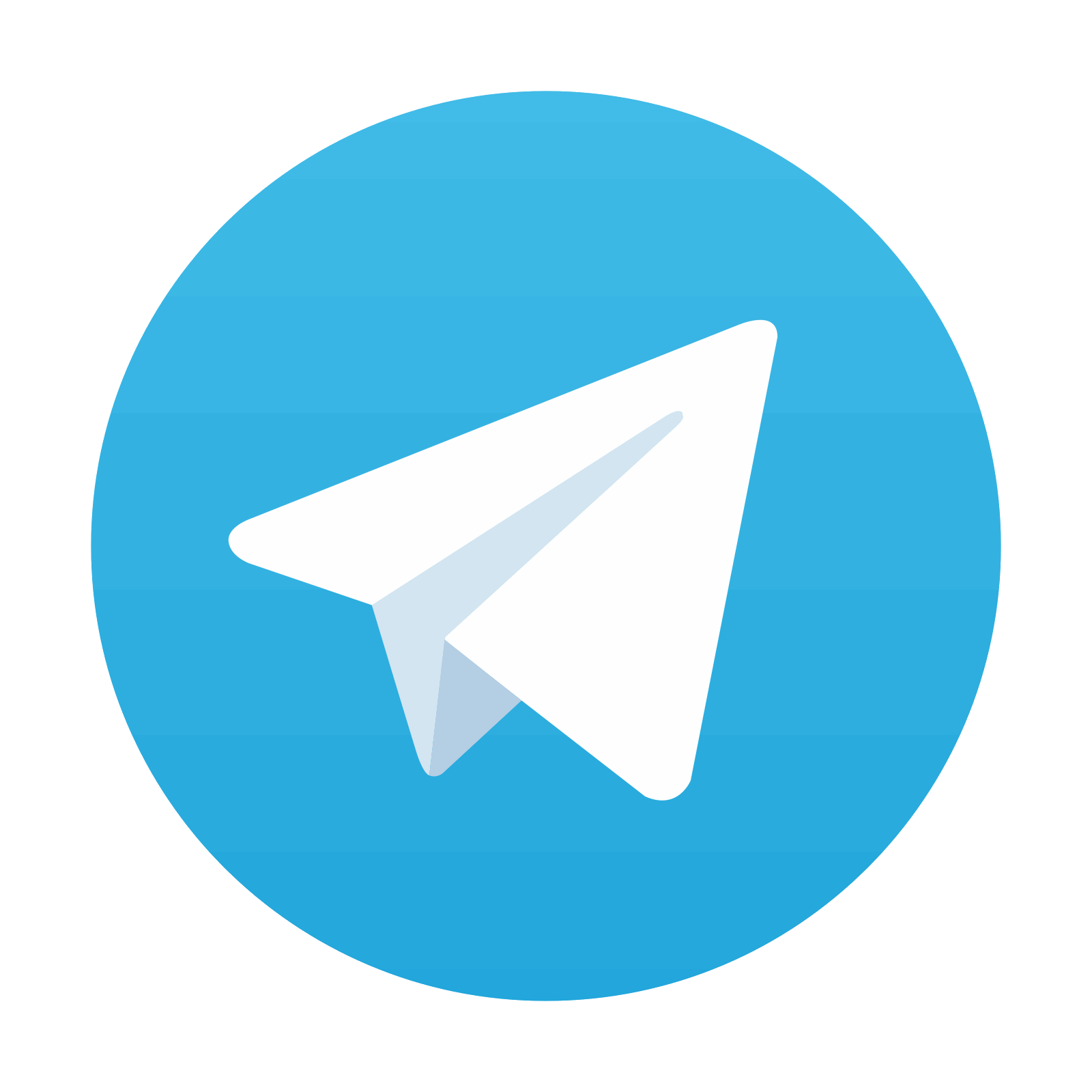
Stay updated, free articles. Join our Telegram channel

Full access? Get Clinical Tree
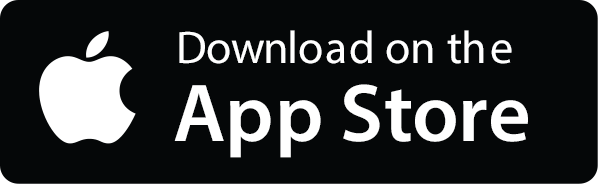
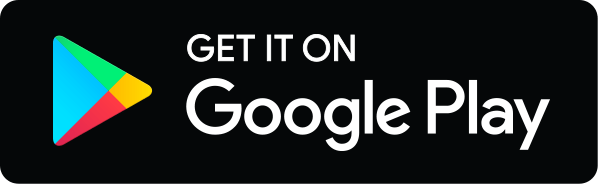