© Springer-Verlag Berlin Heidelberg 2015
Nima Rezaei (ed.)Cancer Immunology10.1007/978-3-662-46410-6_2323. Immunology and Immunotherapy of Breast Cancer
(1)
Centre de Recherche du Centre Hospitalier de l’Université de Montréal, 900 St-Denis Street Montreal, Montreal, QC, H2X 0A9, Canada
(2)
Division of Cancer Medicine, Group Leader, Translational Breast Cancer Genomics Lab, Cancer Therapeutics Program, Division of Research, Peter MacCallum Cancer Centre, East Melbourne, VIC, 3002, Australia
23.1 Introduction
23.2 CD8+ T Cells
23.5 B Cells in BC
23.10.1 MUC-1 Vaccines
23.10.2 HER2 Vaccines
23.10.3 MAGE-A3 Vaccines
23.10.4 Targeting Immune Checkpoints
23.10.5 Anti-CTLA-4
23.10.6 Anti-PD-1
23.10.9 Blocking the Immunosuppressors
23.10.10 Adoptive T Cell Therapy
23.11 Concluding Remarks
Keywords
Breast cancerImmunologyPrognostic markersPredictive marksPD-1Adenosine23.1 Introduction
Breast cancer has not traditionally been considered an “immunogenic” tumor type. While immunosurveillance mechanisms do not seem to have an effect on the growth of primary breast tumors (transplant patients receiving immunosuppressants do not have an increased incidence of breast cancer), immunosuppression does seem to adversely affect metastases growth [1].
Immune cells can exhibit pro- or antitumor effects and affect therapeutic resistance. Avoiding “immune destruction” is now considered an emerging hallmark of cancer [2]. It is now generally accepted that lymphocytic infiltrates, especially consisting of CD8+ T cells, are associated with a better cancer prognosis [3]. The analysis of location, density, and type of tumor-infiltrating immune cells – termed the “immune contexture” – offers the hope of prognostic information and the identification of patients most likely to respond to immunotherapies [4]. As well as this, standard therapies in breast cancer, for example, anthracyclines and trastuzumab, have been shown to modulate immunity as part of their mechanism of action, though not conventionally considered “immunotherapy.” In this review, the role of different immune cell populations in the progression of breast cancer and how these could be harnessed or inhibited in the treatment of breast cancer would be discussed.
23.2 CD8+ T Cells
Over the past recent years, it has become increasingly evident that tumor-infiltrating lymphocytes (TIL) can control the clinical progression of epithelial cancers [5]. In breast cancer, two large series in newly diagnosed or early-stage breast cancer reported a significant correlation between increased lymphocytic infiltration and better clinical outcomes. Denkert et al. [6] analyzed tumor-infiltrating lymphocytes (TILs: using hematoxylin/eosin sections and immunohistochemistry [IHC]) of pretherapeutic core biopsies from over 1,000 breast cancer patients. Mahmoud et al. [7] analyzed tumor-infiltrating CD8+ T cells (by IHC) in 1,334 breast cancer patients. In both studies, high lymphocytic infiltrates or CD8+ T cell counts were associated with improved patient outcome, independent of standard prognostic and predictive factors. In 2009 high risk, newly diagnosed breast cancer patients, TILs were also evaluated using the same method as Denkert et al. but this time on full-face H&E sections taken from surgical removal of the primary tumor [8]. In this study it was found that the prognostic benefit of TILs was largely restricted to the triple-negative breast cancer subtype (TNBC: negative for expression of estrogen receptor [ER], progesterone receptor [PR], and Human epidermal growth factor receptor 2 [HER2]). While TNBC patients usually have a worse, women with TNBC and high TILs had a numerically better disease-free outcome than the women with ER+/HER2− negative tumors who usually have the best prognoses. These data suggest that some patients have preexisting antitumor immunity at diagnosis and this is associated with improved long-term clinical outcomes. The reasons why some breast cancers are associated with TILs and others are not are unclear.
Also in support of a role for tumor-infiltrating CD8+ T cells in controlling breast cancer progression, unsupervised gene expression profiling of breast cancer-associated stroma has revealed an immune gene signature enriched for CD8+ T cell genes associated with good prognosis [9]. Interestingly, Mahmoud et al. [7] reported a positive correlation between CD8+ T cells and high histological grade, younger patient age, and negative estrogen receptor (ER) status. Two other studies found a similar correlation between intratumoral CD8+ T cell counts and negative ER status [10, 11]. The underlying mechanisms for this observation are unclear (and discussed below).
23.3 CD4+ and FOXP3+ T Cells
In contrast to intratumoral CD8+ T cells, analysis of CD4+ T cells on clinical outcomes in breast cancer has resulted in inconsistent results. In general, the presence of CD4+ T cells in tumors has been associated with worse prognosis. For instance, IHC analysis of tissue microarrays derived from 179 treatment-naïve breast tumors revealed that high levels of CD4+ T cells and macrophages were correlated with reduced overall survival (OS). In contrast, high levels of CD8+ T cells combined with low levels of CD4+ T cells were correlated with improved OS [12].
The association between CD4+ T cell infiltrates and poor prognosis is generally attributed to CD4+ FOXP3+ T regulatory cells (Tregs). Tregs were first described as a population of T cells capable of suppressing immune responses in mice and were originally defined by the surface markers CD4 and CD25 [13]. Further investigation revealed that Tregs express and functionally depend on the transcription factor FOXP3 [14–16]. Given its essential role in Treg development, FOXP3 has become a popular marker of Tregs in cancer studies. Intriguingly, studies of the prognostic value of FOXP3+ T cells in cancer patients have led to discrepant findings. Tissue microarray analysis of FOXP3+ cells in 1,445 cases of primary invasive breast cancer revealed that the number of FOXP3+ cells was associated with a worse outcome, but was not an independent prognostic factor in multivariate analysis [17]. On the other hand, Liu et al. [18] reported that intratumoral FOXP3+ cells was an independent predictor of poor prognosis in 1,270 breast cancer cases. Other studies have assessed the impact of FOXP3+ cells on neoadjuvant breast cancer chemotherapy. One study reported that high infiltration of FOXP3+ cells after neoadjuvant chemotherapy was an unfavorable and independent predictor of relapse-free survival (RFS) and overall survival (OS) [19]. Likewise, Ladoire et al. [20] reported that a high CD8/FOXP3 ratio was an independent predictive factor of RFS and OS in HER2-overexpressing breast cancer following neoadjuvant chemotherapy. In contrast, Oda et al. [21] reported that a high level of FOXP3+ cells before neoadjuvant chemotherapy was an independent predictor of pathological complete responses. Others have also reported a positive correlation between high levels of FOXP3+ T cells and good prognosis [22, 23]. Taken together, the role of FOXP3+ cells in breast tumors still remains unclear and warrants further investigation.
23.4 CD4+ Follicular Helper T Cells
Interestingly, a recent publication reported a favorable role for CD4+ T cells [24]. For the first time, CD4+ follicular helper T (Tfh) cells were found in tumor-infiltrating T cell populations, and their presence was associated with better prognosis. Accordingly, human breast tumors with high TILs were found to have higher frequency of CXCL13-producing Tfh cells. Notably, Tfh cells were found associated with tertiary lymphoid structures. Tertiary lymphoid structures (TLS) had been previously identified in lung and colorectal cancers, and their presence linked with better prognosis. It is now clear that Tfh cells are an important constituent of TLS in human breast tumors.
23.5 B Cells in BC
Intratumoral B cells have been associated with a favorable prognosis in breast cancer patients. An early gene expression study of 200 consecutive lymph node-negative cases reported a B cell metagene primarily formed by immunoglobulin heavy- and light-chain genes that was associated with metastasis-free survival in highly proliferating breast tumors [25]. Interestingly, immunoglobulin κ C (IGKC) as a single marker has also been shown to have similar predictive and prognostic value compared to the entire B cell metagene [26]. Building up of these findings, Mahmoud et al. [27] recently analyzed the density and localization of B cells in 1,470 breast cancer cases using IHC. Consistent with previous studies, increased B cell infiltration was associated with improved survival. Notably, B cell infiltration correlated with hormone receptor negativity and basal phenotype.
23.6 Macrophages and MDSC in BC
Macrophage infiltrates in breast cancer often correlate with a worse clinical outcome. Tumor-associated macrophages have been associated with suppressed antitumor CD8+ T cells, increased angiogenesis, and increased metastasis. The presence of CD68+ macrophages is generally inversely correlated with CD8+ T infiltrates in human breast tumors [12]. Interestingly, tumor-associated macrophages were found significantly increased after chemotherapy with paclitaxel and in patients who had received neoadjuvant chemotherapy compared to surgery alone. Using transgenic mouse models of breast cancer, Coussens and colleagues also demonstrated that tumor-associated macrophages can directly promote breast cancer metastasis, via IL-4 producing CD4+ T cells [28]. Other myeloid cells composed of incompletely differentiated cells, termed myeloid-derived suppressor cells (MDSC), have also been shown to enhance breast tumor growth in mice [29]. Human equivalents of MDSC have been identified [30]. Recently, Sceneay et al. [31] demonstrated that tumor hypoxia, via MDSC recruitment, can alter the lung microenvironment to make them more permissive for metastasis, thereby driving the formation of a pre-metastatic niche. Furthermore, increase in circulating MDSC in human cancer has been associated with stage 4 disease [32].
23.7 Immune Infiltrates, Gene Signatures, and BC Subtypes
The correlation between lymphocytic infiltrates and clinical outcomes in breast cancer varies across molecular subtypes. In 2011, DeNardo et al. [12] performed a meta-analysis of CD68 and CD8 gene expression in 4,000 breast cancer cases and reported that a CD68high/CD8low immune gene signature correlated with reduced OS for basal or HER2+ breast cancer subtypes, but not for luminal breast cancers. Similarly, a metagene of STAT1 signaling – a surrogate of interferon response – was associated with better outcome in triple-negative breast cancer (TNBC) and HER2+ breast cancers, but not in luminal cases [33, 34]. Another independent group identified an immune response prognostic gene module in ER−, but not ER+ breast cancers [11].
Among ER− breast cancers, accumulating evidence suggests that basal or TNBC breast cancers are particularly associated with lymphocytic infiltration. In 2012, Liu et al. [35] performed a large-scale IHC analysis of CD8 expression on 3,400 breast cancer cases representing different subtypes and reported that only core basal TNBC demonstrated a significant correlation between intratumoral CD8 staining and favorable prognosis.
A B cell metagene has also been associated with good outcome in TNBC. In a gene expression analysis of 579 TNBC, Rody et al. [36] revealed that a ratio of high B cell and low IL-8 metagenes was identified in 32 % of TNBC patients with good prognosis. Taken together, these studies suggest that clinical outcomes in ER− breast cancers, especially TNBC, are particularly influenced by tumor immune responses.
Why immune infiltration appears more relevant for breast cancers which are ER negative or overexpress HER2 as opposed to other subtypes is unknown. We speculate that it could be due to the poorly differentiated nature and high genomic instability of these subtypes. In TNBC, the intrinsic nature of the tumor cells may help explain its propensity for inflammatory responses. For instance, signaling pathways able to downregulate ER and HER2 expression may be associated with increased pro-inflammatory activity. This is supported by the identification of lactoferrin as a repressor of hormone receptors and HER2 expression in breast cancer cells [37]. Lactoferrin concomitantly induces the production of cytokines and chemokines, including MIP-1, MIP-2, IL-10, and IL-4. Increased immune infiltration in TNBC may also be associated with increased genetic instability due to p53 loss of function and BRCA1/2 disruption, which are common features of this molecular subtype. In line with this model, a recent study in high-grade serous ovarian cancer revealed an important positive correlation between BRCA1 disruptions and high levels of TILs [38].
Among TAA, cancer-testis (CT) antigens represent potential antitumor antigens since they are not shared with normal somatic cells. Interestingly, analysis of CT antigens in human breast cancer revealed higher expression in ER– vs. ER+ breast cancers and co-expression with basal cell markers [38]. Similar findings were reported by Curigliano et al. [39]. Consistent with these two studies, IHC analysis of eight CT antigens revealed significantly more frequent expression in ER− vs. ER+ human breast cancers [40]. In a study of genes differently expressed in TNBC, Karn et al. [41] further reported that among genes showing no correlation with known markers of TNBC, the most prominent group of genes encoded for CT antigens. Taken together, these studies suggest that CT antigen expression is a common feature of TNBC.
23.8 Effect of Chemotherapy on Tumor Immunity
In addition to being involved in the natural progression of cancer, immunity can affect the activity of various anticancer agents. Recent evidence suggests that some chemotherapeutic drugs, such as anthracyclines and oxaliplatin, rely on the induction of anticancer immune responses. In mouse models of cancer, chemotherapy with anthracyclines or oxaliplatin requires priming of IFN-γ-producing CD8+ T cells for optimal treatment response. In cancer patients, high levels of interferon (IFN)-gamma and CD8+ T cells are predictive of a good clinical response to anthracyclines. The immune-stimulating property of anthracyclines and oxaliplatin was shown to require preapoptotic translocation of calreticulin (CRT) on the tumor cell surface, post-apoptotic release of the chromatin-binding protein high-mobility group B1 (HMGB1), and extracellular release of adenosine triphosphate (ATP). CRT, HMGB1, and ATP act in concert to promote tumor antigen presentation by dendritic cells (DCs) via activation of CD91, TLR-4, and purinergic P2X7 receptors, respectively. It was recently demonstrated that chemotherapy-induced autophagy is essential for the release of ATP and subsequent anticancer immunity. Accordingly, autophagy-deficient tumor cells are unable to release ATP in response to anthracyclines or oxaliplatin and fail to elicit CD8+ anticancer T cells. This suggests that patients with autophagy-deficient tumor cells might benefit from therapeutic strategies designed to compensate this process in order to trigger immunogenic signaling.
Extracellular ATP appears as a central activator of chemotherapy-induced antitumor immunity. However, tumors can overexpress ecto-nucleotidases, which catabolize the hydrolysis of extracellular ATP into adenosine. Expression of these ecto-nucleotidases, such as CD39 and CD73, has two major consequences: decreasing the concentration of pro-inflammatory ATP and increasing the concentration of immunosuppressive adenosine. Notably, while the catabolism of ATP into adenosine monophosphate (AMP) is reversible, catalyzed by membrane-bound kinases, the conversion of AMP into adenosine by CD73 is irreversible. This places CD73 at a crucial checkpoint in the conversion of immune-activating ATP into immunosuppressive adenosine. Several groups have now demonstrated the importance of CD73 in the suppression of anticancer immunity. In breast cancer, CD73 is overexpressed in response to loss of ER expression [42]. Studies undertaken by the authors and confirmed by others revealed that targeted blockade of CD73 can effectively reduce tumorigenesis and metastasis of breast cancer in mice [43–47]. Furthermore, the authors recently demonstrated that CD73 expression is significantly associated with worse prognosis in TNBC patients and that the CD73-adenosinergic pathway promotes chemoresistance to anthracycline in mice [48]. Targeting CD73 therefore represents a novel approach with the potential to enhance the efficacy of chemotherapy for treatment of breast cancer.
23.9 Targeted Therapies (Including Trastuzumab and Tyrosine Kinase Inhibitors)
Antitumor immunity also plays a major role in the efficacy of targeted therapies. Tumor-targeted monoclonal antibodies (mAbs), such as trastuzumab, rely in part on immune-mediated killing [48]. While innate immune responses, in particular via Antibody-dependent cell-mediated cytotoxicity (ADCC), appear to be important for trastuzumab activity [49], recent studies suggest that trastuzumab also stimulates adaptive antitumor immunity. Two studies in mice showed that trastuzumab-like therapy requires adaptive CD8-dependent immune responses to mediate optimal activity [50, 51]. These studies support a model whereby trastuzumab activates MyD88-dependent Toll-like receptors – most likely via the release of high-mobility group box 1 (HMGB1) following ADCC – stimulates the release of type I interferons (IFNs), and primes adaptive IFN-γ-producing CD8+ T cells. These studies raise the possibility that combination strategies may be used to capitalize on the adaptive tumor-specific immunity generated by anti-ErbB2 mAbs. Consistent with this notion, Stagg et al. [51] demonstrated that anti-PD-1 and anti-CD137 mAbs can each synergize with anti-ErbB2 mAb therapy. This data advocates that enhancement of T cell antitumor immunity should be evaluated in the clinical setting in combination with trastuzumab.
Data also exists suggesting that preexisting immunity could be important for trastuzumab efficacy. Evaluation of TILs in baseline primary tumor specimens before treatment found that those patients with high levels of TILs derived more benefit from trastuzumab added to their cytotoxic chemotherapy [52]. This data suggested that though trastuzumab has been thought to act primarily through inhibition of cell signaling, it may also serve to relieve tumor-mediated immunosuppression through yet undefined mechanisms. This data also supports that some breast cancer subtypes may be more amenable to immunotherapeutic approaches.
23.10 Immunotherapy of Breast Cancer
23.10.1 MUC-1 Vaccines
A promising candidate antigen for breast cancer vaccination is the MUC-1 antigen. Both normal and cancerous breast cells express MUC-1. However, breast cancer cells often express an aberrantly glycosylated form of MUC-1 [53]. The presence of circulating antibodies against MUC-1 at the time of breast cancer diagnosis has been correlated with a favorable outcome [54]. In addition to stimulating humoral immune responses, aberrantly glycosylated MUC-1 can also stimulate CD8+ T cells [55]. While early MUC-1 vaccines failed to elicit effective antitumor immune responses in clinical trials, a glycosylated MUC-1-derived glycopeptide covalently linked to a Toll-like receptor (TLR) agonist has been recently shown to elicit potent humoral and cellular immune responses [56], highlighting the importance of maintaining conformational elements of MUC-1 to achieve successful vaccination.
23.10.2 HER2 Vaccines
Several clinical trials have investigated the use of immunogenic peptides derived from the HER2 protein in order to induce therapeutic vaccination against HER2+ breast cancer. Different trials were conducted with increasing doses of peptide (AE37 or E75 or GP2 peptide) and varying amounts of the immune adjuvant granulocyte-macrophage colony-stimulating factor (GM-CSF) injected intradermally. All three peptide vaccines have been well tolerated [57]. Interestingly, the combination of trastuzumab and HER2 vaccine has also been recently investigated [58]. In a phase I/II study, 22 patients with advanced metastatic HER2+ breast cancer already receiving trastuzumab were vaccinated with six inoculations of a HER2 peptide-based vaccine. The study revealed that preexisting immunity specific for HER2 (possibly as a result of previous trastuzumab therapy) could be significantly augmented with vaccination.
23.10.3 MAGE-A3 Vaccines
Vaccines targeting CT antigens, such as MAGE-A3, are also being tested in clinical studies. In a phase II trial of non-small cell lung cancer (NSCLC), it was reported that patients whose tumors had been removed by surgery experienced 25 % fewer recurrences following vaccination against MAGE-A3. A phase III trial involving 2,300 NSCLC patients positive for MAGE-A3 antigen is currently underway [59]. The outcome of this phase III clinical trial could be decisive in the development of tumor vaccines targeting MAGE-A3 or other CT antigens in breast cancer.
23.10.4 Targeting Immune Checkpoints
While correlative studies suggest that antitumor immunity can control breast cancer progression and patient outcome, tumors persist despite being infiltrated with tumor-specific CD8+ T cells. This apparent paradox is at least partly due to the exhausted nature of tumor-infiltrating T cells and the presence of immunosuppressive factors in the tumor microenvironment. One of the most important means of immune regulation is a process known as “T cell exhaustion,” which results from chronic exposure to antigens and is characterized by the upregulation of inhibitory receptors. Blocking of one or several of these inhibitory receptors, also known as “immune checkpoints,” with mAbs has been the mainstay of recent developments in cancer immunotherapy.
23.10.5 Anti-CTLA-4
The first immune checkpoint inhibitor to be tested in clinical trial was ipilimumab (Yervoy, Bristol-Myers Squibb), an anti-CTLA-4 mAb. CTLA-4 belongs to the immunoglobulin superfamily of receptors, which also includes PD-1, B and T lymphocyte attenuator (BTLA), T cell immunoglobulin- and mucin domain-containing protein 3 (TIM-3), and V-domain immunoglobulin suppressor of T cell activation (VISTA). In 2011, the US Food and Drug Administration approved the use of ipilimumab for treatment of unresectable or metastatic melanoma, either as initial therapy or after relapse.
The mechanism of action of ipilimumab includes enhanced antitumor function of effector T cells, increased ratio of CD8+ T cells to Foxp3+ T regulatory cells (Tregs), and interference with the suppressive function of Tregs [60]. CTLA-4 blockade has been shown to expand a subpopulation of tumor-infiltrating CD4+ T cells that express high levels of ICOS and secrete IFN-γ [61]. These CD4+ ICOS+ T cells might play a role in the therapeutic activity of anti-CTLA-4 mAb therapy, as there frequency correlates with survival in treated melanoma patients. Nevertheless, it is still not clear whether the activity of ipilimumab is dependent on the blockade of CTLA-4-mediated negative regulatory signal in effector T cells or to its interference with Treg function. Another potential mechanism of action includes Antibody-dependent cell-mediated cytotoxicity (ADCC) of CTLA-4-expressing cells. The major drawback to anti-CTLA-4 mAb therapy is the generation of immune-related toxicities due to on-target effects. It has been reported that up to 25 % of patients treated with ipilimumab developed serious grade 3–4 adverse events [62], reflecting the importance of CTLA-4 in maintaining immune homeostasis. Unfortunately, toxicity is not always associated with therapeutic benefit. Thus, a major challenge in the use of anti-CTLA-4 mAb is to define favorable clinical settings that strike an optimum balance between tumor immunity and autoimmunity.
23.10.6 Anti-PD-1
PD-1 is another inhibitory co-receptor expressed on activated and exhausted T cells. Its ligand, PD-L1, is often found overexpressed in various types of cancer. Administration of mAbs blocking anti-PD-1/anti-PD-L1 enhances adaptive antitumor immune responses by preventing T cell exhaustion [63]. Anti-PD-1 mAb blocks interactions between PD-1 and its ligands, PD-L1 and PD-L2, whereas anti-PD-L1 mAb blocks interactions between PD-L1 and both PD-1 and CD80. PD-1 is expressed by activated CD4+ and CD8+ T cells, B cells, monocytes, and NKT cells. It has two ligands, PD-L1 and PD-L2, with distinct expression profiles. Expression of PD-L1 has been shown to be associated with poor prognosis in melanoma and hepatocellular carcinoma [64, 65]. Notably, cytotoxic chemotherapeutics such as paclitaxel, etoposide, and 5-fluorouracil have been shown to upregulate PD-L1 expression on breast cancer cells [66].
There are currently six agents blocking the PD-1/PD-L1 pathway in clinical evaluation: MDX-1106/BMS-936558/ONO-4538 (fully human IgG4 from BMS), CT-011 (humanized IgG1 from CureTech/Teva), MK-3475 (human IgG4 from Merck), MPDL3280A/RG7446 (from Genentech), BMS-936559 (fully humanized IgG4), and AMP-224 (a B7-DC/IgG1 fusion protein licensed to GSK) [www.clinicaltrials.gov]. Two phase I trials recently reported clinical responses with anti-PD-1 or anti-PD-L1 mAb in pretreated patients with diverse tumor types [67, 68]. Anti-PD-1 mAb therapy was associated with objective responses in 18 % of patients with NSCLC (14 of 76 patients), 28 % of patients with melanoma (26 of 94 patients), and 27 % of patients with renal cell cancer (9 of 33 patients) [68]. Anti-PD-L1 mAb therapy was associated with objective responses in 17 % of patients with melanoma (9 of 52), 12 % of patients with renal cell cancer (2 of 17), 10 % of patients with NSCLC (5 of 49), and 6 % of ovarian cancer patients (1 of 17) [67]. Notably, anti-PD-1 and anti-PD-L1 mAb therapy caused drug-related grade 3 or 4 adverse events in 14 and 9 % of patients, respectively. Strikingly, in the context of anti-PD-1 mAb therapy, objective responses occurred only in PD-L1+ tumors (36 % response rate) compared to no clinical responses in PD-L1-negative tumors.
Recent safety and efficacy data from the phase I/II study using another anti-PD-1 antibody called lambrolizumab have been published [69]. A total of 135 patients with advanced melanoma were treated in a dose escalation followed by cohort expansion. Common adverse events attributed to treatment were fatigue, rash, pruritus, and diarrhea, all of which were low grade, with hypothyroidism, transaminitis, and pneumonitis also occurring (all grades, but <10 %). The confirmed response rate across all dose cohorts (RECIST 1.1) was 38 % (95 % confidence interval [CI] 25–44), with the highest response rate seen in the highest dose (10 mg/kg every 2 weeks). Responses were durable, with 81 % of patients who had a response (42 of 52) still receiving treatment (median progression-free survival was longer than 7 months). The majority of responses were seen at the time of first imaging at 12 weeks. Some patients with stable disease at first imaging also showed objective responses with further time, these being durable as well. Biopsies of regressing lesions revealed infiltration by CD8+ T lymphocytes, consistent with the mode of action of the drug.
Recently, Muenst et al. [70] investigated the prognostic value of PD-1 expression in human breast cancer TILs. Using IHC analysis of a tissue microarray of 660 breast cancer cases, the study revealed that the presence of PD-1 expression on breast cancer TILs was associated with significantly worse overall survival in luminal B and basal-like breast cancer, but not in luminal A or HER2+ breast cancer.
PD-L1 expression using IHC has also been examined in breast cancer. Expression was found in the tumor but also in the surrounding TILs. Tumoral expression was associated with high histologic grade and ER negativity, whereas PD-L1 expression in TILs was associated with larger tumor size, HER2 positivity, and high histologic grade [71]. Expression at the mRNA level is higher in breast cancer subtypes that are ER-negative and HER2-amplified compared with ER-positive tumors (unpublished data). PD-L1 in TILs in breast cancer may also present an exhausted T cell response resulting from chronic antigen exposure, suggesting that TILs represent previous activation of antitumor immunity with subsequent suppression. This suppression may also be enhanced by the tumor.
23.10.7 Combination of Checkpoint Inhibitors
While inhibition of a single immune checkpoint can prolong the survival of cancer patients, an important question that remains is whether combinatorial checkpoint blockade can be synergistic in promoting anticancer activity. The first combination of immune checkpoint inhibitors to be tested in mice was the combination of anti-CTLA-4 and anti-PD-1 mAbs. Curran et al. [70] demonstrated that blockade of CTLA-4 and PD-1 in mice allows CD8+ and CD4+ T cells to survive in the tumor microenvironment, proliferate, and carry out effector function. More recently, combination of anti-CTLA-4 and PD-1 in metastatic melanoma showed impressive tumor regressions and durable responses providing proof-of-concept of this hypothesis.
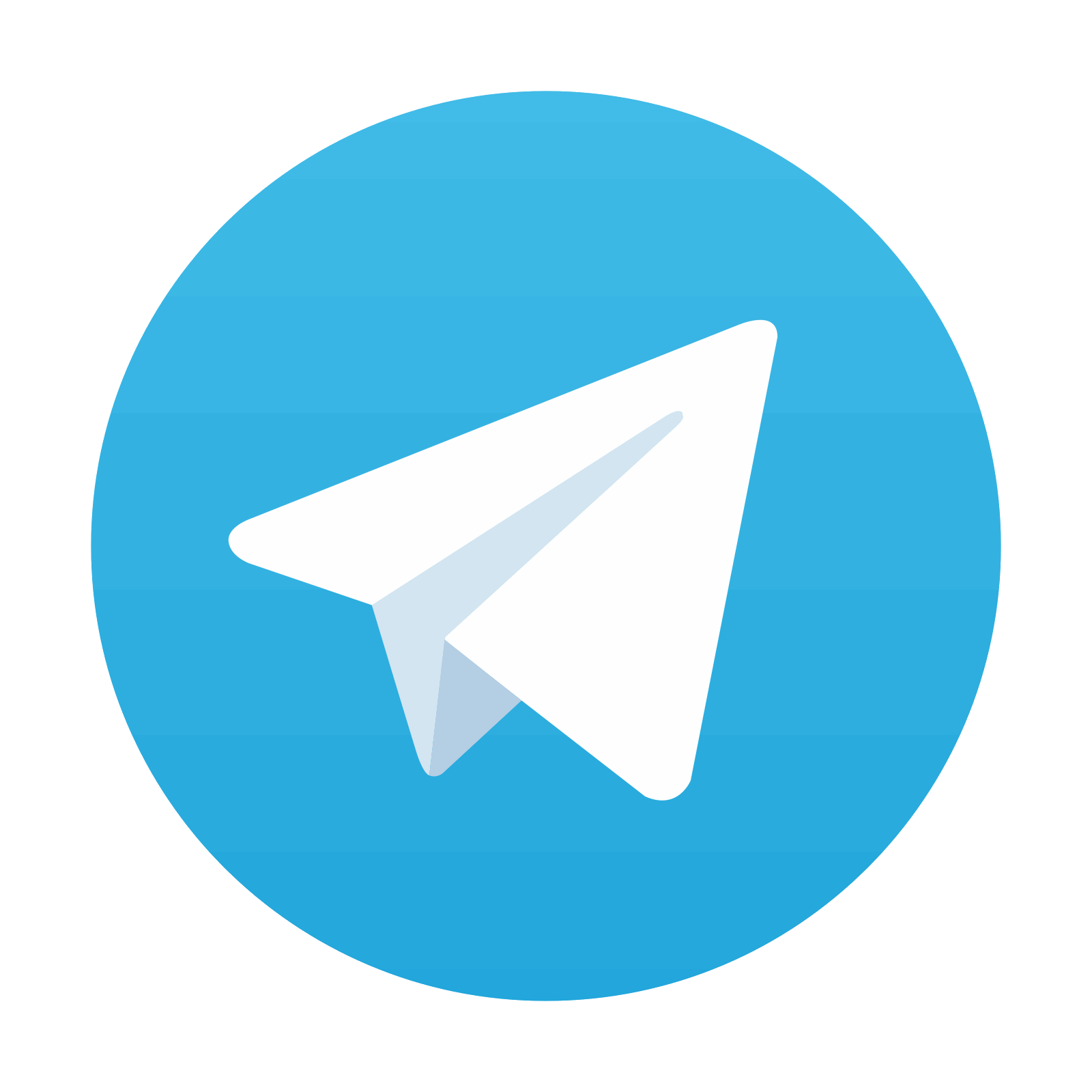
Stay updated, free articles. Join our Telegram channel

Full access? Get Clinical Tree
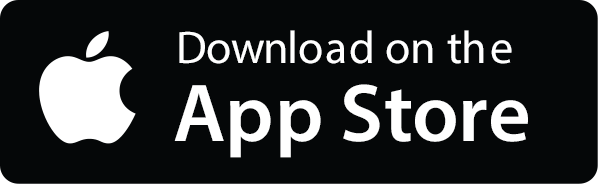
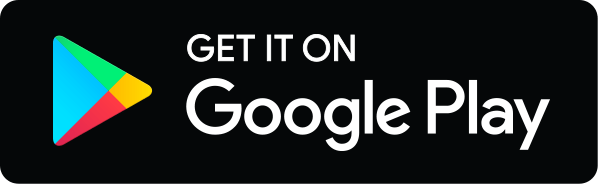