Tumor type
Markers
Reference
Bladder
ABCG2high SP (side population, Hoechst dye)
[12]
ALDH1high
[13]
Breast
CD44+CD24−/low
[10]
ALDH1high
[14]
Colon
CD133+
[15]
EpCAMhigh CD44+
[16]
ALDH1high
[17]
Gastric
CD44+
[18]
ALDH1high
[19]
Glioma
CD133+
[11]
A2B5+
[20]
CD15+
[21]
Head and neck
CD44+
[22]
ALDH1high
[23]
Leukemia
CD34++CD38−
[9]
CD44+
[24]
ALDH1high
[25]
Lung
CD133+
[26]
CD133+epithelial-specific antigen (ESA)+
[27]
ALDH1high
[28]
Liver
CD90+
[29]
CD133+
[30]
Melanoma
ABCB5+
[31]
CD133+ ABCG2+
[32]
Ovarian
CD44+ CD117+
[33]
ALDH1high
[34]
Pancreas
CD133+
[35]
CD44+CD24+ESA+
[36]
ALDH1high
[37]
Prostate
Side population, Hoechst dye
[38]
ALDH1high
[39]
Different markers, such as CD44, CD24, CD90, CD133, and ESA, have been used to identify cancer stem cells in different tumor types. In addition, in many tumor types cancer stem cells have been demonstrated to express increased levels of aldehyde dehydrogenase (ALDH) (Table 9.1). ALDH1, a detoxifying enzyme, is responsible for the oxidation of aldehydes to carboxylic acids and thus might serve to prevent cells from oxidative insult facilitating their survival. It has been shown that hematopoietic and neural stem and progenitor cells have high ALDH1 activity [25, 40–42]. Increased ALDH1 activity has been found in stem cell populations in many cancers including bladder, breast, colon, gastric, head and neck lung, pancreatic, prostate, and so on [13, 14, 17, 19, 23, 28, 37, 39], suggesting that ALDH1 may serve as a reliable cancer stem cell marker for many types of tumor.
9.3 Cancer Stem Cells Are Resistant to Conventional Tumor Therapies
Cancer stem cells share many properties of their normal tissue counterparts. It has been demonstrated that normal stem cells demonstrate relative resistance to drugs and toxins through the expression of ATP-binding cassette (ABC) transporters and an active DNA-repair capacity. It follows that cancer stem cells might also possess these resistance mechanisms [8]. Indeed, resistance has been seen in both in vitro and in vivo models of cancer stem cells in response to chemotherapy or radiation therapy [43].
Hermann et al. isolated the human pancreatic cancer stem cells defined by CD133 expression. They found that the CD133+ cells showed increased resistance to standard chemotherapy (gemcitabine) compared with the CD133− cells derived from the same tumor [35]. Dylla et al. described epithelial-specific antigen (ESA)+ CD44+ phenotypes in human colorectal cancer stem cells. When these cells were xenotransplanted into NOD/SCID mouse and treated with cyclophosphamide (CPA) or irinotecan, residual tumors were enriched for cells with the cancer stem cell phenotype [44]. They found that a large subpopulation of ESA+ CD44+ cells has high ALDH1 activity. Using shot hairpin RNA against ALDH1, they demonstrated that knockdown of ALDH1 expression sensitized these cells to CPA [44]. Since ALDH oxidizes and inactivates the bioactive metabolic by product of CPA, aldophosphamide/4-hydroxycyclophosphamide (4-HC), they speculated that ALDH1 may play a major role in CPA resistance. Therefore, ALDH1 may not only serve as a marker for cancer stem cells but also play a key role in cancer stem cell resistant to chemotherapy.
Although the mechanisms underlying drug resistance are poorly understood, recent studies show that cancer stem cells resistance to chemotherapy and radiotherapy may also involve increased expression of drug efflux pumps, DNA repair, as well as interactions of cancer stem cells with their microenvironment. Cancer stem cells, like normal stem cells, express high levels of ABC transporters [8, 45]. ABC transporters are transmembrane proteins that utilize the energy of adenosine triphosphate (ATP) hydrolysis to transport a wide variety of substrates across extra- and intracellular membranes. These efflux transporters allow cancer stem cells to preserve genome integrity by pumping DNA damaging drugs out of the cell. Downregulating the expression of ABC transporters in cancer stem cells results in the increased death of cancer stem cells due to acute cytotoxic injury and the induction of apoptosis by chemotherapy agents in vitro [45]. In addition, cancer stem cells are able to rapidly repair DNA damage caused by DNA-targeting agents and radiation therapy [46]. Checkpoint kinases 1/2 (Chk1/2 kinases), which become activated after genotoxic stress induces cell cycle arrest allowing for DNA repair, may be one of the potential modulators of cancer stem cell resistance to DNA-targeting agents. Chk1/2 inhibitors partially reverse the resistance of glioblastoma cancer stem cells to radiation-induced cell death [46, 47]. In another report, Woodward et al. found that side-population cells with stem cell characteristics enriched after radiation in human breast cancer cell line MCF-7. They demonstrated differential expression of activated β-catenin and γH2AX in mammospheres derived from the cancer stem cells vs. non-cancer stem cells. These results suggested an important role of Wnt/β-catenin signaling in mediating more effective DNA repair in cancer stem cells promoting their resistance to radiation [48]. Furthermore, interactions of cancer stem cells with their microenvironment (niche) may also contribute to drug resistance. For example, CXCR4, a receptor for stromal cell-derived factor 1 (SDF1, also known as CXCL12) is expressed on many cancer cells. SDF1 is a niche-derived chemoattractant for CXCR4+ cells, thereby enhancing their entry into the bone marrow [49]. CXCR4-SDF1 inhibition sensitized cancer stem cells to chemotherapy [50, 51]. Altogether, these results suggest that alternative therapeutic strategies are needed to specifically target cancer stem cells. To this end, cancer stem cell-targeted immunotherapy has shown promise.
9.4 Innate Immune Response to Cancer Stem Cells
The role of innate immune effector cells in tumor immunosurveillance remains controversial. On one hand, these cells allow for the recognition and destruction of malignant cells before they generate a tumor mass. On the other hand, innate cells may be suppressed and therefore mediate immune tolerance to tumors. Recent advances in understanding NK cell function in antitumor immune responses have revealed a complex dynamic interaction between NK cells and tumor cells. Decreased peripheral blood NK cell function has been reported in many cancer patients [52, 53]. Accumulating evidence also indicates that NK cell cytotoxicity and INF-γ secretion are suppressed, which facilitates a minor group of stem cells to survive in the tumor microenvironment [54, 55]. It was proposed that cytotoxic NK cells (CD16+CD56+/dimCD69−) can be conditioned to differentiate into noncytotoxic cells (CD16−/dimCD56−/dimCD69+) through the interaction of cancer stem cells or primary stem cells with monocytes in situ [56, 57]. These anergic CD16−/dimCD56−/dimCD69+ NK cells have increased ability to secrete inflammatory cytokines and growth factors. Supporting this concept, tumor associated macrophages (TAMs) have been shown to medicate cytokine-dependent inhibition of NK cell function [58].
In contrast, other studies have demonstrated that cancer stem cells, e.g., glioblastoma stem cells and primary oral squamous carcinoma stem cells (OSCSCs), are significantly more susceptible to NK cell-mediated cytotoxicity than their differentiated counterparts [59, 60]. Castriconi et al. reported that human glioblastoma cells with stem cell-like properties display markers typical of neural stem cells [59]. These cells, despite their resistance to freshly isolated NK cells, are highly susceptible to lysis mediated by both allogeneic and autologous IL-2 (or IL-15)-activated NK cells. These stem cell-like glioblastoma cells do not express protective levels of HLA class I molecules, but express high levels of CD155 and CD112, the ligands of DNAM-1-activating NK receptor which trigger optimal NK cell cytotoxicity [59]. Increased NK cell cytotoxicity and augmented secretion of IFN-γ were also observed by Tseng et al. [60]. In their study, oral squamous cancer stem cells (OSCSCs) were identified by expression of CD133 and CD44bright markers. These OSCSCs release significantly lower levels of GM-CSF, IL-6, and IL-8; have decreased expression of phospho-Stat3, B7H1, and epidermal growth factor receptor (EGFR); and have much lower constitutive NF-κB activity than differentiated oral squamous carcinoma cells (OSCCs). When these OSCSCs were cocultured with IL-2-treated NK cells, the NK cells lysed OSCSCs significantly more than differentiated OSCCs. An increase in IFN-γ secretion and decrease in IL-6, GM-CSF, and IL-8 secretion were also detected in the supernatants of NK cells cocultured with OSCSCs compared with cytokine secretion in the supernatants of NK cells cocultured with differentiated OSCCs. In addition, the authors demonstrated that normal primary stem cells, like human embryonic stem cells (hESCs), mesenchymal stem cells (hMSCs), dental pulp stem cells (hDPSCs), and induced pluripotent stem cells (hiPSCs), are also susceptible to NK cell-mediated cytotoxicity. Collectively these studies suggest that undifferentiated cells are susceptible targets of NK cell cytotoxicity. Stem cells may become resistant to NK cell-mediated cytotoxicity once they differentiate.
Another kind of innate immune cell, γδ T cells represent a small part of the lymphocyte population that expresses a TCR complex where CD3 is associated with γ and δ chains. These lymphocytes were originally characterized as strong IFN-γ-producing cells which exhibit MHC-unrestricted lytic activity, thus making them potential anticancer stem cell mediators [61, 62]. The majority of γδ T cells in human peripheral blood are of the Vγ9Vδ2 phenotype and constitute 1–5 % of circulating lymphocytes [63, 64]. Many in vitro and in vivo studies have demonstrated antitumor activity of the Vγ9Vδ2 T cells. The possible mechanism for this γδ T cell-mediated cytotoxicity includes MHC nonrestricted direct killing of tumor cells, antibody-dependent cell-mediated cytotoxicity (ADCC), and activation of other immune effectors. Vγ9Vδ2 T cells have a unique capacity to recognize and be activated and expanded by non-peptide phosphoantigens, such as zoledronate and pamidronate. In 2009, Todaro et al. combined human Vγ9Vδ2 T cells with bisphosphonate zoledronate and found that Vγ9Vδ2 T cells efficiently killed human colon cancer stem cells [65]. In this setting, production of cytokines (TNF-α and IFN-γ) and cytotoxic and apoptotic molecules (TRAIL and granzymes) were induced after exposure of Vγ9Vδ2 T cells to sensitized human colon cancer stem cells. According to their report, γδ-TCR predominantly mediates cancer stem cell recognition and killing. The granule exocytosis pathway mediates Vγ9Vδ2 T cell cytotoxicity, which is highly dependent on isoprenoid production by tumor cells. In a clinical study, activated Vγ9Vδ2 T cells in combination with zoledronate show increased CD69 expression, indicating an activated phenotype. These Vγ9Vδ2 T cells displayed upregulated expression of peripheral tissue-homing chemokine receptors, CCR5 and CXCR3. In contrast, expression of lymphoid homing receptors, CCR7 and CXCR5, decrease [66]. More importantly, these zoledronate-activated Vγ9Vδ2 T cells are cytotoxic in vitro against tumor targets, and adoptively transferred Vγ9Vδ2 T cells traffic predominantly to the lungs, liver, and spleen and, in some patients, to metastatic tumor sites outside these organs, suggesting that therapy with activated Vγ9Vδ2 T cells is feasible [66]. Taken together, these results indicate that in vitro expansion of autologous γδT cells in combination with other antitumor agents, like zoledronate, pamidronate, and cytokines, may benefit cancer treatment via cancer stem cell elimination.
9.5 Cancer Stem Cell-Primed T Cells Specifically Targeting Cancer Stem Cells
Cell-mediated immunity plays a major role in the rejection of tumors and apparently has an advantage over innate immune cells. In conventional cancer immunotherapy, bulk tumors comprising heterogeneous cancer cell populations have been used as antigen either to prime pre-effector T cells or to generate DC-based vaccines. However, tumor-specific antigens may be selectively expressed on differentiated tumor cells and are not expressed on cancer stem cells [43, 67]. Cancer stem cells express cancer stem cell-specific antigens which may be different from either differentiated tumor cell antigens or normal stem cell antigens [68].
In support of this hypothesis, recent studies have demonstrated that cancer stem cell-specific CD8+ T cells can be generated in vitro for injection into NOD/SCID mice to mediate tumor regression [69–72]. In 1999, Bonnet et al. identified putative human acute myeloid leukemia (AML) stem cells and cancer stem cell-specific CD8+ T cells [71]. When NOD/SCID mice were transplanted with the mixture of human AML cells and a CTL clone specific for minor histocompatibility (H) antigens, these human leukemic cells were completely eliminated from the inocula. Based on the data, they proposed that AML stem cells can be eliminated by minor H antigen-specific CTL clones. Consistent with Bonnet’s report, Brown and colleagues identified tumor spheres (TSs), which were expanded from glioma explants, displayed consistently high CD133 expression, and exhibited stem cell-like characteristics [70]. These CD133+ TSs express significant and comparable levels of MHC I and ICAM-1/CD54, which are required for T-cell/tumor immunologic synapse formation. These brain tumor stem/initiating cells (BTSCs) were susceptible to perforin-dependent CTL-mediated cytolysis. To assess whether the protein processing machinery is sufficiently intact for the BTSCs population to process and present antigen for CD8+ CTL recognition, the authors engineered glioma TSs to endogenously express the cytomegalovirus (CMV) pp65 antigen by reconstructed pp65-lentiviral transduction. They found that CMV-specific CTLs mediate the CMV-transducted glioma TSs cytotoxicity. To test whether CTL can eliminate all tumor-initiating activity of the BTSCs in vivo, CMV pp65-expressing TSs and pp65-specific CTLs were co-injected into NOD/SCID mice. It was found that all pp65 antigen-positive tumor cells were ablated, while pp65− tumor cells were resistant to the pp65-specific CTL and efficiently engrafted. This result established that direct recognition of antigen-expressing TS cells by CTLs is required to ablate tumor initiation. In another study, Visus and colleagues reported that cancer stem cell-specific CD8+ T cells can be generated by using antigenic peptide from aldehyde dehydrogenase 1 family member A1 (ALDH1A1) expressed by ALDHA1bright cancer stem cells from squamous cell carcinoma of the head and neck (SCCHN) [69, 73]. They demonstrated that transfer of ALDH1A1-specific CD8+ T cells eliminated ALDHbright cells, inhibited tumor growth and metastases, and prolonged survival of xenograft-bearing immunodeficient mice [69]. CD133 is a common marker of cancer stem cells. Huang et al. generated an anti-CD3/anti-CD133 bispecific antibody (BsAb) and bound it to the cytokine-induced killer (CIK) cells as effector cells (BsAb-CIK) to target CD133high CSCs. The killing of CD133high pancreatic (SW1990) and hepatic (Hep3B) cancer cells by the BsAb-CIK cells was significantly (p < 0.05) higher than the killing by the parental CIK or by CIK cells bound with anti-CD3 (CD3-CIK) without CD133 targeting. In nude mice, the BsAb-CIK cells inhibited CD133high tumor growth significantly (p < 0.05) more than that by CIK or CD3-CIK cells or by the BsAb alone. Treatment with the BsAb-CIK cells significantly downregulated the expression of S100P and IL-18 bp, but upregulated STAT1. The findings may facilitate the development of novel immunotherapies for patients with cancer containing CD133high CSCs by selectively targeting this cell population [74]. Together, these results support the conclusion that potential cancer stem cell-specific T cells can be generated in vitro for subsequent adoptive transfer into tumor-bearing hosts to target cancer stem cells and lay the foundation for the development of new immunotherapeutic approaches to eradicate tumors in vivo.
9.6 Development of Cancer Stem Cell-Specific Vaccine in Immunocompetent Host
In contrast to the generation of cancer stem cell-specific T cells in vitro, for their in vivo use, the development of a cancer stem cell-specific vaccine depends on the integrated host cellular and humoral immunity. However, most cancer stem cell studies have been performed with human tumor-derived cancer stem cells in immunocompromised mice [69–72]. These xenotransplantation assays have shown a great deal of variability in the frequency of cells identified with tumorigenic potential, depending on the degree of host immunodeficiency [75]. Most importantly, due to the lack of cellular and humoral immunity in the NOD/SCID mice, these hosts are not suitable for the immunological evaluation of cancer stem cell vaccines. The efficacy of cancer stem cell-based vaccination against tumors needs to be assessed in immunocompetent hosts.
Given the fact that dendritic cell (DC) vaccines have significant potential in cancer immunotherapy, cancer stem cell-primed DC vaccines have been proposed. Glioblastoma-derived cancer stem cells express MHC I [72]. After coculturing human immature, autologous DCs with these irradiated brain tumor stem cells, the cancer stem cell-primed mature DCs express co-stimulatory molecules CD80, CD86, and CD40 and stimulate significant Th1 (IFN-γ) response in vitro [72]. Our group at the University of Michigan assessed the feasibility of cancer stem cell-primed DC vaccine in vivo and demonstrated that cancer stem cell-primed DC vaccination confers significant antitumor immunity in immunocompetent hosts [76]. In this study, the tumorigenicity of murine ALDH+/high cancer stem cells were characterized in two histologically different tumors (D5 melanoma and SCC7 squamous cell carcinoma) from two genetically distinct immunocompetent hosts (B6 and C3H mice). Using purified cancer stem cells as an antigen source to prime DCs, we evaluated the protective effects of cancer stem cell-primed DC vaccines in syngeneic mouse tumor models. The study demonstrated that cancer stem cell-primed DC vaccination significantly prevented lung metastasis formation in murine D5 melanoma model and subcutaneous (s.c.) tumor growth in murine SCC7 squamous cell carcinoma model compared with the positive control group using DCs pulsed with the unsorted heterogeneous tumor cells [76]. Mechanistically, this study observed high IgG production by splenocytes obtained from the host subjected to the cancer stem cell-DC vaccine, efficient binding of these antibodies to the cancer stem cells, and significant cancer stem cell lysis mediated by these antibodies in the presence of complements. In addition, CTLs generated from the PBMCs and splenocytes obtained from cancer stem cell-DC-vaccinated hosts selectively killed cancer stem cells. This study revealed direct targeting of cancer stem cells by cancer stem cell-primed antibody and CTLs. Collectively, these data indicate that enriched cancer stem cells are immunogenic and more effective as an antigen source than unselected tumor cells in inducing protective antitumor immunity [76]. In line with the above mentioned studies, Phuc and colleagues used cancer stem cell-primed DC vaccine in murine breast cancer models [77]. They found that breast cancer stem cell extract-loaded DCs migrate to the spleen, activate CD8+ and CD45+ T cells, and induce CTL responses [77].
The mechanisms responsible for mediating cancer stem cell-DC responses remain to be elucidated. Experimental evidence has demonstrated that cancer stem cell-DC vaccines confer animal host antitumor immunity by direct targeting of cancer stem cells by antibody and CTLs [77], but the molecule(s) responsible for such cancer stem cell-conferred antitumor activity remain unknown. In this regard, Duarte et al. used mass spectrometry to compare protein expression difference between cancer stem cells and non-cancer stem cells in colon carcinoma and identified four proteins specifically expressed in the cancer stem cells [78]. Among these proteins, two of them (heat shock protein 27-kDa and aldose reductase) are already known to be associated with treatment resistance and poor prognosis in colon cancer. They then inoculated rats with the cancer stem cell lysate (not purified antigens) as vaccine and found that the cancer stem cell-based vaccine reduced tumor volume and occurrence and inhibited experimental liver metastasis in half of the animals [78]. This report provides evidence for the existence of cancer stem cell-associated antigens in the lysate used to prime DCs.
Different signals induce distinct DC phenotypes (subsets) and yield distinct immune responses, e.g., Th1 response, Th2 response, Th17 response, or the generation of Treg cells. To circumvent potential unfavorable outcomes, it is important to understand how cancer stem cell antigens interact with distinct subsets of DCs. This will help elucidate the molecular pathways of DC maturation, cancer stem cell antigen presentation as well as the discovery of novel adjuvants for cancer stem cell-DC-based vaccines.
In protective studies, normal animals are inoculated with cancer stem cell-based vaccines before tumor cell injection. If a cancer stem cell vaccine is to be clinically relevant, it needs to be evaluated in a therapeutic setting. Although cancer stem cell-based vaccines have shown vigorous protective antitumor activity in several animal models, it is important to determine the therapeutic efficacy of cancer stem cell-based vaccines in established tumors, a more clinically relevant setting.
9.7 Targeting the Tumor Microenvironment as a Strategy to Enhance Immunological Targeting of Cancer Stem Cells
Normal stem cells reside in a distinct environment called the “stem cell niche.” The niche regulates stemness, proliferation, and apoptosis resistance of stem cells. Cancer stem cells also reside in a niche within the tumor. The local tissue environment contributes to the self-renewal and differentiation of cancer stem cells. Growth factors, cytokines, and diverse stromal cells, such as mesenchymal stem cells and immune cells in the cellular microenvironment, are essential for cell nutrition, intercellular communication, signal transduction, and cell fate [79]. Therefore, these components in cancer stem cell niche may provide additional therapeutic targets.
Inflammatory cytokines including IL-1, IL-6, and IL-8 in cancer stem cell niche are involved in driving cancer stem cell self-renewal [80]. These cytokines activate Stat3/NF-ĸB pathways in both tumor and stromal cells and in turn stimulate further cytokine production, generating positive feedback loops contributing to cancer stem cell self-renewal. Inhibitors of these cytokines and their receptors have been developed [80]. Using siRNA to knock down testicular nuclear receptor 4 (TR4) in the prostate cancer (PCa) stem/progenitor cells led to downregulation of octamer-binding transcription factor 4 (Oct4) expression, which, in turn, downregulated the IL-1 receptor antagonist (IL1Ra) expression. This approach resulted in increased drug sensitivity of cancer stem cells to the two commonly used chemotherapeutic drugs, docetaxel and etoposide [81]. In addition, blockade of the IL-8 receptor CXCR1 using antibody or repertaxin (a small-molecule CXCR1 inhibitor) selectively depleted the cancer stem cell population in human breast cancer cell lines in vitro, followed by the induction of massive apoptosis in the bulk tumor population via FASL/FAS signaling [82]. Another cytokine IL-6 has been shown to be a direct regulator for cancer stem cell self-renewal [83, 84]. Kim and colleagues observed that part of the non-stem cell population converted to cancer stem cell-like cells by promoting OCT–4 gene expression. Anti-IL-6 antibody inhibited the JAK1 and STAT3 activation as well as OCT–4 gene expression [85]. These studies indicate that IL-6 and its receptor may serve as attractive therapeutic targets in attempt to immunologically target cancer stem cells.
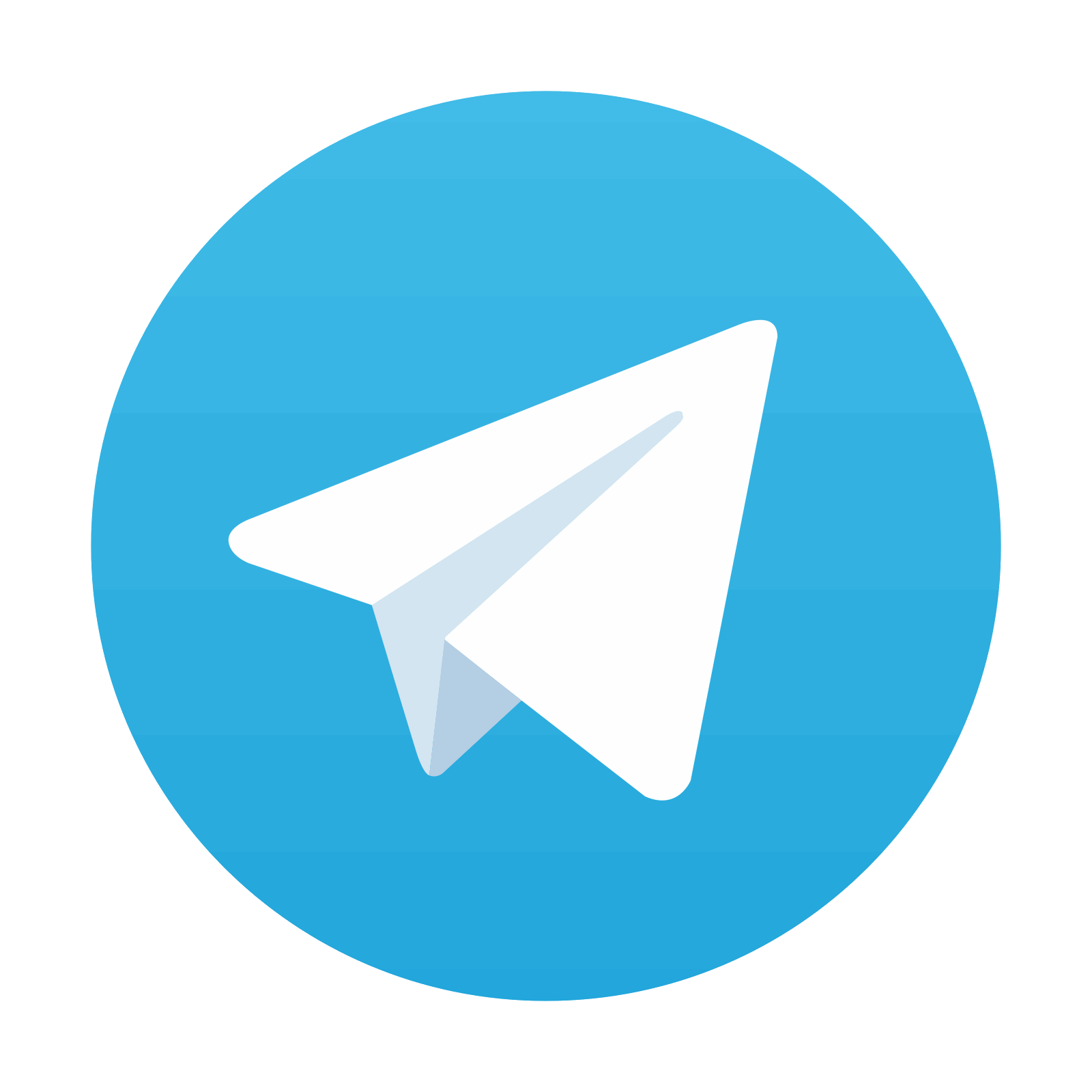
Stay updated, free articles. Join our Telegram channel

Full access? Get Clinical Tree
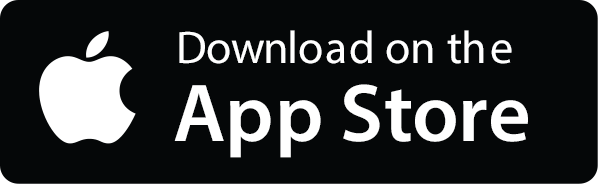
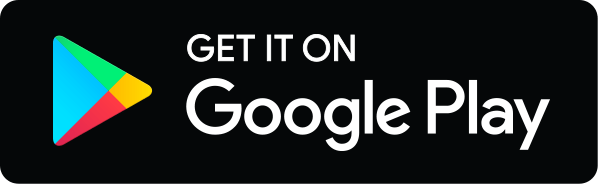