IgG
IgA
IgM
IgD
IgE
Heavy chain
Classes
Gamma (γ)
Alpha (α)
Mu (μ)
Delta (δ)
Epsilon (ε)
Subclasses
IgG1, IgG2, IgG3, IgG4
IgA1, IgA2
None
None
None
Light chain types
Kappa, κ
κ
κ
κ
κ
Lambda, λ
λ
Λ
λ
λ
Molecular formula
γ2κ2
α2κ2a
(μ2κ2)5
δ2κ2
ε2κ2
γ2λ2
α2λ2a
(μ2λ2)5
δ2λ2
ε2λ2
Designation
IgG-κ
IgA-κ
IgM-κ
IgD-κ
IgE-κ
IgG-λ
IgA-λ
IgM-λ
IgD-λ
IgE-λ
A brief review of the history, structure, properties, and functions of immunoglobulins is useful for an understanding of the monoclonal proteins.
In 1937, Tiselius [2] used electrophoretic techniques to separate serum globulins into three components, which he designated α, β, and γ. Interestingly, this paper, which led to his Nobel Prize and later to the presidency of the Nobel Foundation, was rejected initially by The Biochemical Journal [3]. Electrophoresis was applied to the study of multiple myeloma by Longsworth et al. [4], who demonstrated the tall, narrow-based “church-spire” peak. The method was cumbersome and difficult, so electrophoresis was not readily available until the early 1950s, when filter paper was introduced as a supporting medium (zone electrophoresis). Cellulose acetate then supplanted filter paper [5] and now electrophoresis is routinely performed on agarose gel or in solution within capillary tubes.
Before 1960, the term γ-globulin was used to designate those proteins that migrated in the γ-mobility region (toward the cathode) of the electrophoretic pattern. The γ-globulin level was increased by immunization and decreased when absorbed by exposure to the specific antigen. Later, it was recognized that some antibodies migrate in the fast γ-region and others in the slow γ-gamma and that some sediment in the ultracentrifuge as 7S and others as 19S molecules. It was not until late in the 1950s that the concept of a family of proteins with antibody activity was recognized. These proteins are now referred to as immunoglobulins; the five groups presently recognized and their properties are listed in Table 29.2 [6].
Table 29.2
Properties of immunoglobulins of normal human serum
IgG | IgA | IgM | IgD | IgE | |
---|---|---|---|---|---|
Electrophoretic mobility | γ–α2 | γ–β | γ–β | γ–β | γ–β |
Sedimentation coefficient | 6.7 S | 7–15 S a | 19 S | 7 S | 8 S |
Molecular weight | 150,000 | 160,000–400,000 a | 900,000 | 180,000 | 200,000 |
Carbohydrate (percent) | 2.6 | 5–10 | 10 | 10–12 | 11 |
T 1/2 (days) | 23 | 5.8 | 5.1 | 2.8 | 2.3 |
Serum concentration (mg/dl) | 700–1,500 | 60–400 | 60–300 | 0–14 | 0.05 |
Percentage of total serum Immunoglobulin | 74 | 21 | 5 | 0.2 | 0.002 |
Percentage of total body pool in intravascular space | 45 | 42 | 76 | 75 | 51 |
Percentage of intravascular pool catabolized per day (normal) | 6.7 | 25 | 18 | 37 | 89 |
Normal synthetic rate (mg/kg per day) | 33 | 24 | 6.7 | 0.4 | 0.02 |
Fixes complement | IgG1 and IgG3; IgG2 variable | Alternate pathway | Yes | No | No |
Crosses placenta | Yes | No | No | No | No |
Immunoglobulin G
In normal serum, three-fourths of the immunoglobulin is IgG. This class has a molecular weight of 150,000 and a sedimentation coefficient of 6.7S. Its electrophoretic mobility is predominantly in the γ fraction but can range from slow γ (cathodal region) to α2. Many antibodies to both bacteria and viruses are of the IgG class.
A reducing agent such as mercaptoethanol will disrupt the disulfide bonds linking the four polypeptide chains of the IgG molecule, but the protein will retain its tetrapeptide structure. If the reduced protein is alkylated with iodoacetamide and is fractionated in the presence of a denaturing solvent (e.g., guanidine or urea) that is capable of dissociating noncovalent bonds, two kinds of immunoglobulin polypeptide chains can be recovered: heavy (H) and light (L) (Fig. 29.1).
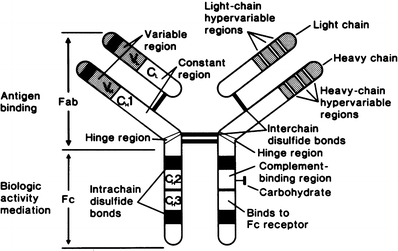
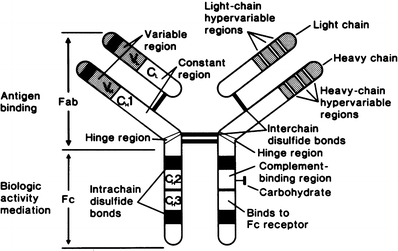
Fig. 29.1
Schematic representation of immunoglobulin G (IgG) molecule, with cleavage by papain into Fab and Fc fragments (Modified with permission from Terhorst C, David J. Antigens, antibodies, and T cell receptors. In Rubenstein E, Federman DD (eds.): Scientific American Medicine: Immunology 6. Vol. 2. New York: Scientific American;1990)
Heavy Chains
Both heavy chains of the IgG molecule are of the γ-class and are identical to each other. The two light chains are also identical to each other—either κ or λ. Each heavy chain has a molecular weight of approximately 55,000 and consists of 440–450 amino acids. Each has a variable (VH) region in which many amino acid substitutions provide each γ chain with binding specificity, and a constant (CH) region which defines the specific class and subclass and imparts the biologic activity of the antibody molecule. The variable region of the γ-chain consists of approximately 110 amino acid residues, beginning at the amino (NH2) terminal of the chain. The constant region of the γ-chain consists of 310–330 amino acids and contains three homology regions or domains, designated Cγ1, Cγ2, and Cγ3. Homology region Cγ1 is in the Fab fragment (Fig. 29.1) and extends from about amino acid 110 to amino acid 220; Cγ2 and Cγ3 are in the Fc fragment, Cγ2 extending from approximately amino acid 220 to amino acid 330 and Cγ3 from there to the COOH terminus.
Immunological analysis of myeloma proteins has disclosed four distinct subclasses of γ-heavy chains, designated IgG1, IgG2, IgG3, and IgG4 (Table 29.3). The average biological half-life of IgG1, IgG2, and IgG4 myeloma proteins injected into patients with malignancies is 21 days, whereas IgG3 has a half-life of 7–8 days. This shorter time is probably due to its fourfold longer hinge region, which appears to be easily accessible to proteolytic enzymes. In addition, IgG3 contains a large number of disulfide bonds. A wide angle of 45°–180° in IgG3 indicates a mobile molecule. Although the hinge region in the IgG3 molecule is four times longer than that in IgG1, it exists in a compact state. In the extended confirmation, the hinge region is 7–9 nm and the length of the intact molecule is only 25 nm [7]. IgG3 does not bind staphylococcal protein A except in rare cases [8]. IgG1 and IgG3 fix complement readily via the classic pathway, but IgG2 does so less well.
Table 29.3
Biologic characteristics of IgG subclasses
IgG1 | IgG2 | IgG3 | IgG4 | |
---|---|---|---|---|
Percent of total IgG | 70 | 20 | 6 | 4 |
Biologic half-life (days) | 21 | 21 | 7 | 21 |
Human monocyte receptors | Yes | No | Yes | No |
Fixation of complement | Yes | Variable | Yes | No |
Reaction with staphylococcal A protein | Yes | Yes | No | Yes |
Restricted antibody toward: isoagglutinins, Rh, diphtheria or tetanus toxoid, viruses (HIV, CMV) | Yes | No | No | No |
Tetanus toxoid, teichoic acid, polysaccharides, lipopolysaccharides | No | Yes | No | No |
Rh, viral neutralizing, HIV, CMV | No | No | Yes | No |
Circulating anticoagulants, factors, VIII and IX, phospholipase A | No | No | No | Yes |
Although many antigens evoke an antibody response within the IgG subclasses proportional to their distribution in normal serum, others produce antibodies mainly within one subclass. For example, almost all antibodies to factor VIII (found in hemophilic persons, postpartum women, and elderly patients) are of the IgG4 subclass, while without predilection only 3–4 % of the antibodies would be of this subclass [9]. Most antibodies to dextran, levan, and teichoic acid are of the IgG2 subclass. Antibodies developed against polysaccharide antigens are also primarily found in IgG2 [10].
Although it has been reported that the serum antiplatelet antibody was restricted to the IgG3 subclass in idiopathic thrombocytopenic purpura (ITP), all four IgG subclasses were bound to platelets of ITP patients in the same distribution as in normal serum [11]. Presumably, these differences represent nonspecific binding to platelets.
Although specific IgG subclass deficiencies have been associated with increased infections, the clinical impact of selective subclass deficiencies is unclear. Among a group of patients with selective IgA deficiency, those with reduction of IgG2 had frequent respiratory infections, but those with normal IgG2 levels did not [12]. In 139 patients with isolated IgA deficiency and an increased susceptibility to infection, IgG4 deficiency was more common than in controls. Contrary to the prior study, there was no significant association between IgG subclass deficiency and increased susceptibility to infection [13]. The IgG2 subclass is thought to be most important in protecting against bacterial sepsis [14]. In a series of 22 patients with ataxia–telangiectasia, very low or borderline-low serum IgG2 levels were found in all, and IgG4 was undetectable in 19 of the 22 patients. Nine of the patients with an undetectable or very low IgG2 level had chronic lung disease or chronic sinusitis, or both [15]. In another series, 4 of 422 unselected patients were found to have an isolated absence of IgG4, as determined by a sensitive radioimmunoassay. All had severe recurrent sinopulmonary infections [16]. Others have suggested that deficiency of IgG3 is becoming more common and is more frequently associated with mild infections [17]. In a group of 89 patients with bronchiectasis, the IgG1, IgG2, and IgG3 levels were increased compared with controls. There was an overall incidence of deficiency of 1 % for subclasses IgG1, IgG2, and IgG3 and 5 % for IgG4. The authors concluded that IgG subclass deficiency was comparatively rare in bronchiectasis [18]. Extensive reviews of IgG subclass deficiency have been published [19–21].
We have found that the distribution of IgG subclasses did not differ among patients with multiple myeloma and those with monoclonal gammopathy of undetermined significant (MGUS; benign monoclonal gammopathy) [22]. There was also no relationship between clinical and laboratory features in multiple myeloma of different subclass types. However, patients with a monoclonal protein of different IgG subclasses have greatly different patterns of suppression. For example, patients with IgG2 monoclonal proteins are more likely to have repressed residual IgG than are patients with IgG3, IgG1, or IgG4 [23].
Radial immunodiffusion in agar with appropriate antisera can be used for semiquantitative concentrations of IgG subclasses in sera, but nephelometry is now commonly used for quantitation of IgG subclasses. These procedures are similar to those used for IgG, IgA, and IgM assays, and they are rapid and reliable.
Light Chains
Historically, the light chains were encountered in 1845, when Macintyre [24] and Henry Bence Jones [25] noted that the urine of a patient with multiple myeloma precipitated when heated, cleared when boiled, and reprecipitated when cooled. In 1962, Edelman and Gally [26] demonstrated that the light chains prepared from a serum IgG myeloma protein and the Bence Jones protein from the same patient’s urine not only had the same thermal solubility but also had similar spectrofluorometric behavior, the same molecular weight, identical appearance on chromatography with carboxymethylcellulose and on starch gel electrophoresis after reduction and alkylation, the same ultracentrifugal pattern, and the same amino acid sequence. Thus, it was found that the urinary proteins that evoked such interest from Macintyre and Bence Jones were the light chain components of the immunoglobulin molecule.
Two distinct groups of Bence Jones proteins (group I and group II) were recognized by Bayne-Jones and Wilson [27] in 1922. In 1956, two major classes of Bence Jones proteins were described by Korngold and Lipari [28], and these have been designated κ and λ. Approximately two-thirds of serum IgG monoclonal proteins are of the κ-type and the remainder are λ. In patients with Waldenström’s macroglobulinemia, approximately 80 % are κ, but the opposite occurs in IgD myeloma, in which 60 % are λ [29].
Immunoglobulin light chains have a molecular weight of 22,500 and contain 210–220 amino acids. Analysis of amino acid sequence in individual light chains has disclosed a constant and a variable domain. The region of the chain from amino acid 107 to the COOH terminus at position 210–220 is very similar in chains of the same type (κ or λ) and has been designated as the constant domain (CL)—although amino acid substitutions have been found at a dozen different positions in the constant region of human λ chains [30]. The region from the NH2 terminus (position 1) to approximately position 107 has been different in every light chain thus far analyzed, and it is called the variable domain (VL). The variable half of the light chain contains the unique thermal solubility characteristics of Bence Jones proteins [31].
Amino acid sequence studies have revealed four basic groups of κ-light chains, designated κI, κII, κIII, and κIV [32]. Their frequencies of occurrence are approximately 60 %, 10 %, 28 %, and 2 %, respectively, and as expected, these figures reflect the proportion of κI, κII, κIII, and κIV light chains in immunoglobulins of normal serum [33]. In a series of 23 patients with acquired Fanconi syndrome, 96 % had a monoclonal κ-protein [34]. In another series, eight of nine patients with acquired Fanconi syndrome had κ1-subtype [35]. In light chain deposition disease, the light chain is κ in 90 % of patients, but the κIV subclass is overrepresented [36, 37]. Six subclasses of λ have been reported with the following distribution: λI, 26 %; λII/λV, 38 %; λIII, 22 %; λIV, 3 %; and λVI, 11 % [38]. There is a preferential association of λVI subclass with primary systemic amyloidosis (AL) [39]. In fact, with rare exceptions, all patients with monoclonal λVI subclass have amyloidosis.
Ordinarily free κ-chains (unbound to heavy chains) are in the monomeric form (molecular weight 22,500), but they may exist as noncovalently linked dimers or as a mixture of monomers and dimers, whereas free λ-chains occur as dimers (molecular weight 45,000) linked covalently via their penultimate cysteinyl residues [33]. In addition, κ-light chains precipitate maximally over a narrower range of pH than do λ-light chains [40]. Recognition of light chains as κ or λ by monospecific antisera depends on the variable and constant domains as well as the interdomain “switch” region between the variable and the constant regions [41].
Bence Jones proteins are not degradation products of the complete immunoglobulin in the serum but are synthesized by plasma cells in molar excess compared to heavy chain synthesis. Putnam and Hardy [42] showed that 13 C-labeled glycine is incorporated promptly into Bence Jones protein and cannot be the result of breakdown of serum or other tissue protein. Light chains clearly are catabolized by the kidney. After injection into mice, 131I-labeled Bence Jones proteins of humans were cleared rapidly from the circulation. Ligating and severing the ureters lengthened the disappearance time only slightly, but removal of the kidneys greatly prolonged the survival of light chains, a finding indicating the major role of renal catabolism. Human IgG was not cleared by the kidney in the same experiments [43].
IgG catabolism is increased at high protein concentrations. At low IgG concentrations, most of the IgG is phagocytized into vesicles, where it is protected from catabolism. When the IgG concentration is high, significant amounts of IgG molecules will not be bound by recycling receptors and will be degraded, the result of which is a high catabolic rate [44–46].
Fab and Fc Fragments
Treatment of IgG with the proteolytic enzyme papain cleaves it into three pieces: two Fab fragments, so named because they possess antigen-binding activity, and an Fc fragment, because in certain species it can be crystallized (Fig. 29.1). Each Fab fragment (molecular weight 52,000) consists of the amino-terminal region of a γ heavy chain and a complete light chain. The hinge region, consisting of 15 amino acid residues between Cγ1 and Cγ2, is a flexible junction between Fab and Fc fragments. This hinge permits the angle between the Fab fragments to vary from a few degrees to almost 180°.
Three regions of “hypervariability” have been identified in VL: positions 24–34, 50–56, and 89–97 [47]. This hypervariability and the existence of similar hypervariable regions in the variable region of the heavy chain (VH) in positions 30–37, 51–68, 84–91, and 101–110 permit the formation of many different antigen-combining sites—each consisting of approximately 30 amino acid residues [48]. These hypervariable regions constitute the antigen-binding site, and the three segments are called complementarity-determining regions, or CDRs (CDR1, CDR2, and CDR3).
The Fc fragment (molecular weigh 48,000) consists of the C-terminal region of both heavy chains, which are linked to each other by disulfide bonds. Biological activities of the Fc fragment include fixation of complement; passive cutaneous anaphylaxis (PCA); binding with rheumatoid factors; binding with receptors on macrophages, monocytes, neutrophils, and lymphocytes; reaction with staphylococcal A protein; and transfer across the placenta. Isotypic specificity—specificity for γ, α, μ, δ, or ε—resides in the Fc fragment. The Fc piece is devoid of antigen-binding activity.
Immunoglobulin A
Two identical heavy α-chains and two identical light chains (either κ or λ) form IgA [49] (Fig. 29.2). IgA has a molecular weight of 160,000 and a sedimentation coefficient of 7S, but it also has a propensity to form polymers with sedimentation coefficients of 9S to 15S. Concentration of IgA polymers correlates directly with the serum viscosity [50]. Its catabolic rate of 5.8 days (T ½) is greater than that of IgG.
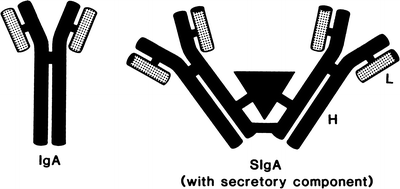
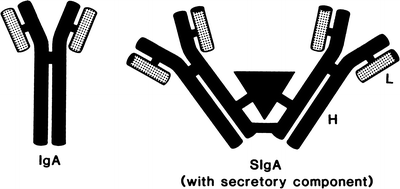
Fig. 29.2
Schematic representation of immunoglobulin A (IgA) and secretory IgA (SIgA) molecules (L, light chain; H, heavy chain) (Reprinted with permission from Kyle RA, Bieger RC, Gleich GJ. Diagnosis of syndromes associated with hyperglobulinemia. Med Clin North Am. 1970;54:917)
There are two subgroups of the α-chain, α1 and α2, and the consequence is IgA1 and IgA2 proteins. Approximately 90 % of polyclonal IgA proteins are of the IgA1 subclass. IgA2 molecules are unique among the immunoglobulins in that the light chains are bound to the α-chains by noncovalent forces instead of disulfide bonds. Almost 90 % of all patients with IgA monoclonal proteins have IgA1. Thus, the distribution of IgA subclasses is the same in both malignant and benign monoclonal gammopathies [51]. The IgA1 subclass is also predominant in French and Japanese patients—94.8 % of the French and 91 % of the Japanese were IgA1 in one study [52].
IgA2 has been found in the glomerular deposits of primary IgA nephropathy, in glomerulonephritis accompanying alcoholic cirrhosis, and in nephritis from Henoch–Schönlein purpura, whereas a preponderance of IgA1 is associated with lupus nephritis [53]. In contrast, as recently reported, oversialylation of polymeric IgA1 may play a role in the pathogenesis of IgA nephropathy [54]. IgA deficiency occurs in two-thirds of patients with ataxia–telangiectasia [55]. Patients with IgA monoclonal gammopathies may produce false-positive reactions of IgA anticardiolipin and anti-β-glycoprotein I antibodies [56].
The daily production of IgA is approximately that of IgG, but the serum concentration is lower because of the greater catabolism of IgA [57]. Monomeric serum IgA may protect endogenous antigens expressed on various cells and tissues by preventing their interaction with humoral and cellular immune mechanisms that may lead to tissue damage [58]. A major source of monomeric IgA is the bone marrow. Spleen cells also produce predominantly monomeric IgA. Production of IgA begins at 4 weeks after birth and approaches the adult level at 1 year. No significant changes in concentration occur during adulthood. Cunningham-Rundles [59] provided an extensive review of the genetic aspects of IgA deficiency.
Secretory Immunoglobulin A
Secretory IgA (SIgA) (Fig. 29.2) is found in high concentration in the secretions of many glands lining the respiratory and gastrointestinal tracts and in tears, colostrum, and urine. SIgA has a sedimentation coefficient of 11S and a molecular weight of 390,000. It is composed of two IgA molecules attached by disulfide bonds to a glycoprotein (molecular weight 60,000–80,000) called the secretory component. Secretory component increases the resistance of the molecules against digestion by trypsin and pepsin. The secretory component is synthesized in mucosal epithelial cells. In one unusual case, cancer cells from bronchogenic adenocarcinoma produced large amounts of secretory component, resulting in high serum levels of SIgA [60]. Serum IgA does not make a significant contribution to IgA in gastrointestinal fluids [61]. In both the gastrointestinal and respiratory tracts, dimeric IgA with J chain (described in the next section) is synthesized in plasma cells and secreted, after which it combines with secretory component on the epithelial cell surface. SIgA is then transported through the cytoplasm in endocytic vesicles to the luminal surface [62].
SIgA exhibits antibacterial and antiviral activity, and evidence indicates the SIgA antibodies are stimulated preferentially by upper respiratory tract infections. SIgA has a neutralizing effect on viral replication [63].
In secretions, IgA prevents microorganisms and foreign proteins from adhering to and penetrating the mucosal surfaces [61]. SIgA provides the most potent immunoglobulin signal for the degranulation of eosinophils. It may be the principal immunoglobulin that mediates eosinophil effector function at mucosal surfaces [64].
In contrast to that in serum IgA, approximately 50 % of SIgA is of the IgA2 subclass. Approximately 95 % of IgA in secretions is polymeric, whereas 90 % of serum IgA is monomeric. A patient with no secretory component in the saliva who presented with chronic intestinal candidiasis has been described [65].
Joining Chains
The joining (J) chain is a non-immunoglobulin protein with a molecular weight of approximately 15,000 [68]. It is bound by disulfide bridges to the Fc portion of the heavy chain and thus forms the dimers or polymers of IgA and the pentamer structure of IgM. It is never found in IgG or in monomers of IgA proteins, and yet J chain is found in cells secreting IgG. Only one J chain is present in each polymer of IgA or pentamer of IgM. The J chain of IgM is identical to that of IgA. The lack of J chain reduces the ability of IgA1 to bind secretory component and interferes with the epithelial transport of polymeric IgA [69].
Immunoglobulin M
A third immunoglobulin, IgM, is composed of subunits linked by disulfide bonds [70, 71] (Fig. 29.3). The heavy chain (μ) of IgM has a molecular weight of approximately 70,000. Two such heavy chains and two light chains form an IgM subunit, which has a molecular weight of approximately 180,000–190,000. Since the molecular weight of IgM is approximately 900,000, it evidently comprises five subunits. The pentamer is assembled in the rough endoplasmic reticulum of plasma cells [72]. The μ-chain consists of one variable (VH) domain and four constant (Cμ1, 2, 3, 4) domains. In contrast to IgG, IgM does not have a hinge. VH and Cμ1 are in the Fab piece, Cμ2 is in the “hinge” region, and Cμ3 and Cμ4 are in the Fc fragment [73]. Although IgM has a sedimentation coefficient of 19S, more rapidly sedimenting molecules of 22S (composed of dimers of the 19S molecule) and 35S may be seen. Low-molecular-weight IgM (sedimentation coefficient 7S) has been found in patients with various pathologic conditions, including lupus erythematosus, macroglobulinemia, other lymphoproliferative processes, and cirrhosis.
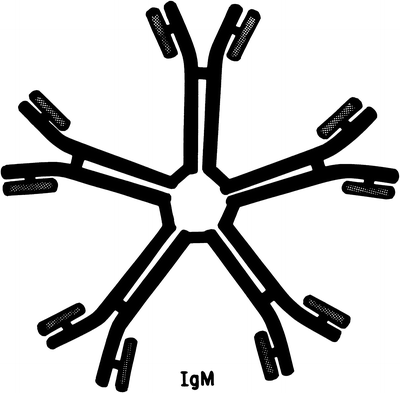
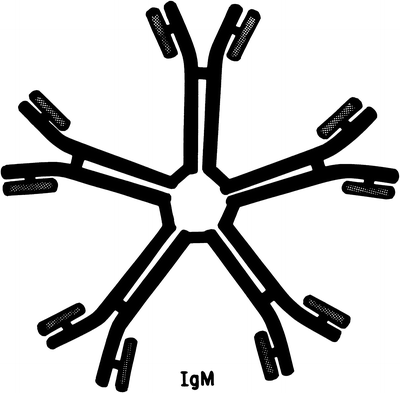
Fig. 29.3
Schematic representation of immunoglobulin M (IgM) molecule (Reprinted with permission Kyle RA, Bieger RC, Gleich GJ. Diagnosis of syndromes associated with hyperglobulinemia. Med Clin North Am. 1970;54:917)
J chains identical to those associated with IgA polymers have been isolated from IgM and aid in initiating or maintaining the tertiary structure of the molecule. The biologic function of secretory IgM has been reviewed [74]. Electron microscopy of IgM has shown figures resembling spiders, each with a central ring, where the Fc portions join together, and legs consisting of the Fab fragments. Subclasses of μ-chains have been reported from three laboratories, but they do not correspond, and specific subclasses of IgM are not recognized. IgM antibodies are the first produced in a primary immune response, and they avidly fix complement. Cold agglutinins, isoagglutinins, rheumatoid factor, and heterophil and Wasserman antibodies, as well as antibodies to various bacteria, are of the IgM class. Early IgM synthesis begins during the first few days of life, and adult serum levels are attained at 1 year. IgM concentrations have been reported to decrease by the sixth decade. Nephelometry frequently overestimates the amount of IgM, when compared with densitometry of serum protein electrophoretograms [75]. Riches et al. [76] reported that 30 % of IgM samples had a value with nephelometry double that calculated from the densitometer pattern. Due to the different quantitation of IgM by electrophoretic and nephelometric assays, the size of the IgM monoclonal protein should preferably be followed by densitometry and the methodology should be consistent [77].
Approximately 80 % of IgM monoclonal proteins are of the κ-type, in contrast to two-thirds of IgG and IgA monoclonal proteins. In spite of a higher percentage of IgM being in the intravascular space, plasmapheresis may not produce a greater loss of IgM than the lower molecular weight immunoglobulins IgA and IgG. In one report plasmapheresis consisting of 2–3 L was performed in 23 patients. Four patients had a monoclonal protein. The average percentage decrease of IgG was 52 %, that of IgA was 55 %, and that of IgM was 51 % [78].
Immunoglobulin D
In 1965, Rowe and Fahey [79] found a myeloma protein containing a heavy chain unlike those of the other immunoglobulins. This class, IgD, is present in a trimodal distribution, with modes at approximately 0.25, 5, and 35 IU/mL. The distribution suggests that levels of IgD in normal persons are strongly influenced by inheritance through a monogenic mechanism [80]. Two-dimensional gel electrophoresis has been reported to be of value in the recognition of IgD monoclonal proteins [81]. However, immunofixation is satisfactory for most cases. IgD is rapidly catabolized and has a serum half-life of 2.8 days. The molecules are relatively heat-labile and sensitive to proteases, and they are readily broken down to Fab and Fc fragments. δ-Heavy chains contain three constant domains and a long hinge consisting of 64 amino acid residues. Human IgD has been sequenced [82]. Antigen-combining activity associated with IgD has been reported with thyroglobulin, nuclear antigens, and insulin. Most circulating B lymphocytes bear IgD, together with IgM, on their surface as membrane-bound immunoglobulin. However, the function of serum IgD is not known [83].
About 75 % of IgD is intravascular, a distribution that may be the result of irregularities in the shape of the molecule. IgD is often found on the surface of lymphocytes [84].
Most patients with an IgD monoclonal protein will have multiple myeloma or primary amyloidosis. IgD accounts for only 2 % of patients with multiple myeloma. Incidences of extramedullary plasmacytomas (19 %), associated amyloidosis (19 %), frequency of Bence Jones proteinuria (96 %), and predominance of λ-light chains (60 %) are greater than those in myeloma associated with IgG or IgA proteins. By contrast, patients with IgD myeloma are more similar to those with light chain (Bence Jones) myeloma [29]. IgD monoclonal proteins have rarely been associated with benign monoclonal gammopathy [85].
Immunoglobulin E
The fifth class of immunoglobulins, IgE, has been purified from the serum of allergic patients [86] and subsequently identified in multiple myeloma [87, 88]. IgE myeloma is rare. Several reviews in the literature identified fewer than 40 cases of IgE myeloma [89–91]. There is a higher frequency of plasma cell leukemia and Bence Jones proteinuria. IgE has a molecular weight of 190,000 and a sedimentation coefficient of 8S. The ε-chain consists of one variable region (VH) and four constant domains but no hinge region. The combination of a short biologic half-life (2.3 days) and a very low rate of synthesis results in an extremely low serum concentration in normal persons.
IgE mediates the wheal-and-flare reaction associated with reaginic allergies and binds to IgE receptors on basophils or tissue mast cells. IgE is fixed on those cells, and histamine, tryptase, and other inflammatory mediators are released when the cell-fixed IgE reacts with the allergen. IgE is often increased in patients with extrinsic asthma and hay fever and in those with parasitic infections [92].
The discovery of IgE did not result in immediate changes in the treatment of allergic diseases [93], and the physiologic contribution of IgE to the maintenance of health remains speculative [87]. The quantitation of allergen-specific IgE, however, is useful in determining the probability that an individual will have an allergic response when exposed to food allergens. More recently, a humanized monoclonal antibody to IgE (omalizumab) has been used for the treatment of asthma associated with high IgE [94].
Immunoglobulin Gene Structure and Rearrangement
Molecular cloning of the genes for the immunoglobulin heavy and light chains has shown that their genomic sequences consist of noncontiguous variable, diversity (heavy chain only), joining, and constant region segments [95–107]. Figure 29.4 shows the basic structure of each gene complex and its chromosome location [95–101, 105]. During B-cell differentiation, DNA rearrangement occurs, and one of the several κ-variable regions (Vκ) combines with a specific joining region (Jκ) segment [96, 97, 102, 107] (Fig. 29.5). This rearranged allele that encodes Vκ, Jκ, and the single κ-constant region (Cκ) is now transcribed. The remaining intervening sequences are removed by RNA splicing, and the resultant messenger RNA is translated into the κ-light chain protein. The leader portion of the mRNA is responsible for the transport of the protein through the cell and is later removed. Both the rearranged variable and joining light chain gene segments encode the variable portion of the light chain protein [96, 97, 107].
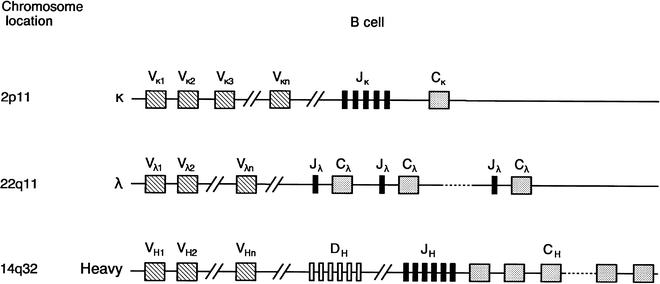
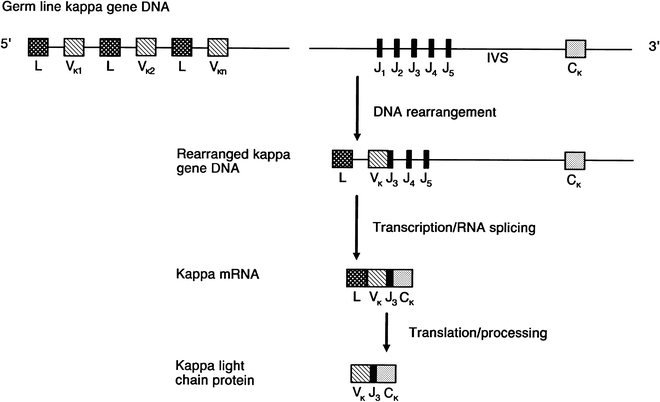
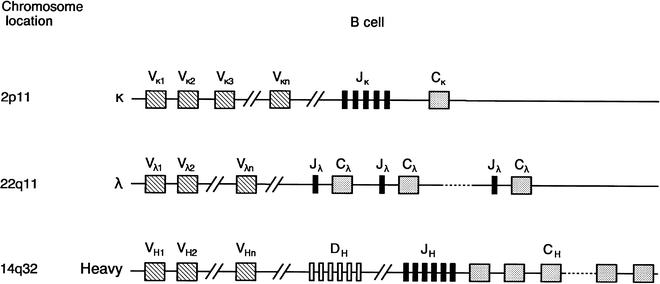
Fig. 29.4
Schematic representation of immunoglobulin heavy chain and κ- and λ-light chain loci. The chromosome location of each of the human loci is given at the left. Germline or unrearranged configuration for each gene complex is diagrammed. C constant, D diversity, J joining, V variable (Modified with permission from Cossman J, Uppenkamp M, Sundeen J et al. Molecular genetics and the diagnosis of lymphoma. Arch Pathol Lab Med. 1988; 112:117)
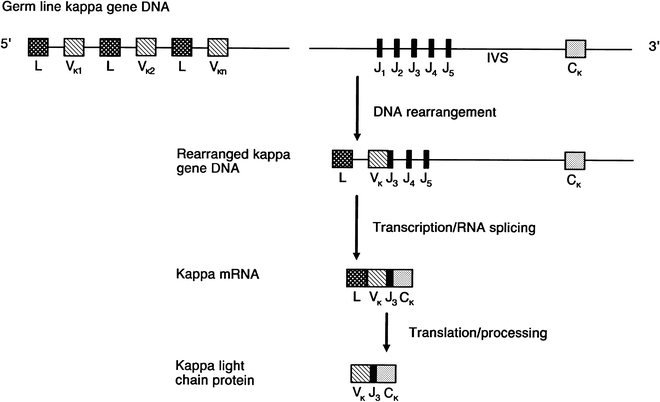
Fig. 29.5
Schematic representation of rearrangement of κ-gene locus. During B-cell differentiation, DNA rearrangement takes place and one of the several κ-variable regions (Vκ), each accompanied by a leader (L) sequence, combines with one of the five joining (Jκ) segments. This rearranged allele that encodes Vκ, Jκ, and a single κ-constant region (Cκ) is transcribed and ultimately translated into the κ-light chain protein. IVS intervening sequence (Modified from Korsmeyer SJ, Waldmann TA. Immunoglobulins. II: Gene organization and assembly. In Stites DP, Stobo JD, Fudenberg HH, Wells JV (eds.): Basic and Clinical Immunology. 5th Ed. Los Altos, CA: Lange Medical Publications;1984. With permission)
The κ-light chain gene complex consists of approximately 100 variable region segments, five joining segments, and one constant κ region [102, 103] (Fig. 29.4). The λ-gene complex is also composed of many variable region segments (Vλ) [105] but, in contrast to κ, the λ-gene complex exhibits multiple duplicated constant regions (Cλ), each associated with a separate J region (Jλ) [96, 106]. Furthermore, unlike κ, the human λ-constant gene locus often varies from one individual to another, as evidenced by different restriction fragment length polymorphisms on Southern analysis [106].
The organization of the heavy chain gene complex is different from that of the light chain genes in that three DNA segments, rather than two, join to code for the variable portion of the rearranged heavy gene [108–114]. Families of diversity segments (DH) and six joining segments (JH) are illustrated in Fig. 29.4 [111–114]. One heavy chain diversity segment combines with one joining segment that ultimately joins with one variable region segment (VH) during the process of heavy chain gene recombination [108, 109]. After the first DNA rearrangement that joins the VH, DH, and JH segments to the heavy chain constant region, a B cell can produce IgM and IgD simultaneously [115–118] (Fig. 29.6). Subsequently, a second DNA recombination occurs at one of the switch sites that precede each of the constant region segments and results in an isotype class switch. Figure 29.6 demonstrates that class switch rearrangement of Sμ and Sα and the loss of the intervening heavy chain constant region gene segments results in a class switch from IgM to IgA [115–118].
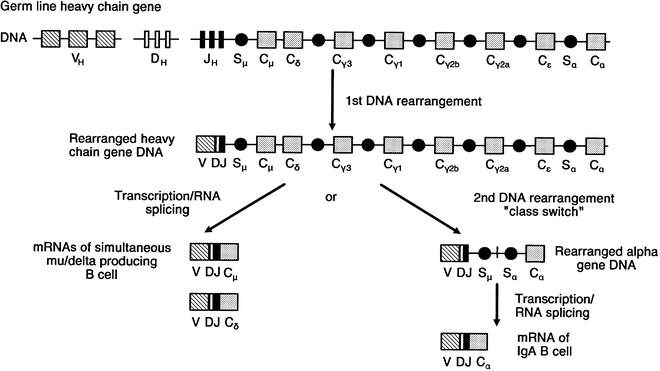
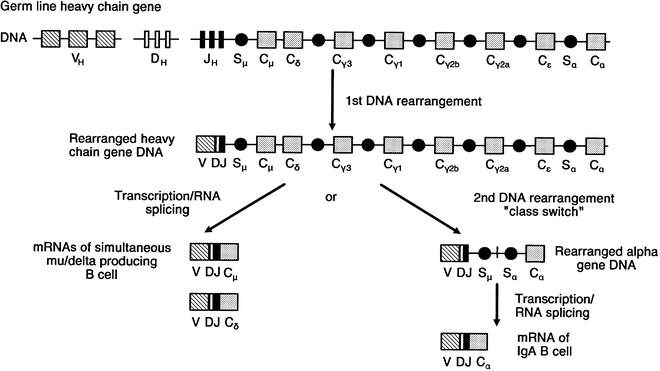
Fig. 29.6
Schematic representation of heavy chain gene locus, indicating order of the heavy chain constant region genes. After the initial DNA gene rearrangement, a B cell can simultaneously produce IgM and IgD using different sites of RNA splicing. Alternatively, a second DNA rearrangement may occur at switch sites (SμSα) in which a “class switch” takes place, resulting in production of a new isotype, such as IgA in this particular example. (Modified from Korsmeyer SJ, Waldmann TA. Immunoglobulins. II: Gene organization and assembly. In Stites DP, Stobo JD, Fudenberg HH, Wells JV (eds.). Basic and Clinical Immunology. 5th Ed. Los Altos, CA: Lange Medical Publications;1984. With permission)
In normal B-cell differentiation, immunoglobulin heavy chain genes rearrange at the pre-B stage before κ-genes [119]. The κ-genes rearrange or are deleted before λ-light chain gene rearrangement can occur [119]. The DH segment accounts for a large portion of the heavy chain’s third hypervariable region and is a major component of the resultant antibody’s idiotype [111, 112, 120]. Idiotype refers to the variable region segments of the antibody molecule and relates to the diversity at the antigen-binding site. Recent studies demonstrate that myeloma heavy chain VDJ genes contain somatic mutations but show little intraclonal variation. These findings suggest that clonal proliferation takes place in a cell type that has already passed through the phase of somatic hypermutation [121, 122].
The ability of an individual to generate a large immunoglobulin repertoire is now evident from an understanding of the mechanism of gene rearrangement. Antibody diversity may occur through (1) selection of one of the large number of different variable regions for the heavy and light chain gene complexes; (2) gene rearrangement in which specific variable, joining, and diversity segments recombine to encode a unique variable region domain; and (3) somatic mutation of V genes during B-cell ontogeny [123, 124]. Because each lymphocyte produces one type of immunoglobulin, the antigen will select and stimulate the B cells that produce high-affinity antibodies.
Monoclonal Proteins
Relationship to Normal Immunoglobulins
Although monoclonal proteins had long been considered abnormal, studies have indicated that they are excessive quantities of immunoglobulins that occur normally. The striking feature of monoclonal proteins that led investigators to consider them abnormal is their homogeneity. Whereas the population of normal IgG in human serum has diverse binding activity and is electrophoretically heterogeneous, IgG monoclonal proteins are localized sharply in their electrophoretic migration.
Kunkel [125] showed that each heavy chain subclass and light chain type in monoclonal proteins has its counterpart among normal immunoglobulins and also among antibodies. After the discovery of the two types of light chains (κ and λ) in myeloma proteins in a ratio of approximately 2:1, these same light chains were detected in essentially the same ratio among normal immunoglobulins. Similarly, the IgG and IgA subclasses and IgD class were discovered among myeloma proteins and then found as normal serum components. These identifications reversed the belief that the monoclonal proteins of multiple myeloma and macroglobulinemia are abnormal and have indicated that they represent the overproduction of a normal product by a clone of abnormal proliferating cells. However, many of the heavy chains found in the heavy chain diseases show significant deletions of amino acids: they are abnormal immunoglobulins.
Even the antigenic determinants (“idiotypic specificities” or “individual antigenic specificities”) that are associated with the binding sites were believed to be associated uniquely with myeloma proteins but have been shown to occur among antibodies [126]. Conversely, studies of highly purified antibodies have revealed a homogeneity approaching that seen in monoclonal proteins. In some instances, after primary immunization with a carbohydrate antigen, monoclonal immunoglobulins consisting of a single light chain type and single IgG heavy chain subclass have been seen [127].
The possibility expressed by Kunkel [125] that myeloma proteins are individual antibodies and are products of the individual plasma cells arising from a single clone of malignant cells has been supported by their antigen-combining activity [128]. Monoclonal antibody activity in humans has been associated with cold agglutinin disease as well as with a wide variety of bacterial antigens including streptolysin O, staphylococcal protein, Klebsiella polysaccharides, and Brucella [129]. It is almost certain that more human monoclonal proteins will be found to have antibody activity. Indeed, it has been postulated that all myeloma proteins may have such activity [130].
In this view, as illustrated in Fig. 29.7, the normal assortment of IgG molecules comprises minute amounts of homogeneous proteins from many diverse single clones of plasma cells and so is called polyclonal. If a single clone escapes the normal control mechanism, excessive proliferation occurs, leading to synthesis of excess protein with a single heavy chain class and subclass and light chain type that is now distinct from the normal immunoglobulins. More than 60 years ago, Gutman [131] used the term M component (or peak) in describing a monoclonal protein. This monoclonal protein is associated with abnormal plasma cell proliferation and may be associated with a neoplastic process. Support for the one-cell, one-immunoglobulin concept comes from the studies of the cellular localization of these proteins. Experiments performed with antisera to light chains have shown that nearly all individual plasma cells contain κ- or λ-light chains but not both.
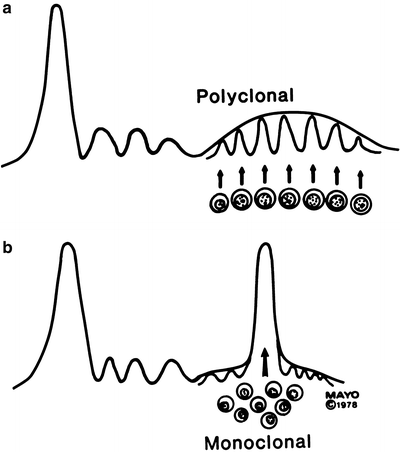
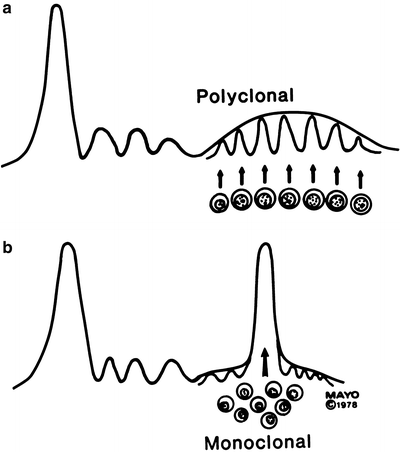
Fig. 29.7
Polyclonal and monoclonal electrophoretic patterns. (a) Broad outline comprising small peaks of many different homogeneous proteins (each represented here in normal amount, related by arrow to its peak), which have been produced by many different plasma cell clones (polyclonal). (b) Tall, narrow peak of homogeneous protein (single heavy chain class and subclass and single light chain type), which is excessive output of single clone (monoclonal) (Reprinted with permission from Kyle RA, Greipp PR. The laboratory investigation of monoclonal gammopathies. Mayo Clin Proc Med. 1978;53:719)
Patterns of Overproduction
Normally plasma cells produce heavy chains and a slight excess of light chains that spill over into the urine and are catabolized. In IgG myeloma, greater than three-fourths of patients have an excess of light chains that may be excreted into the urine (Bence Jones proteinuria) or catabolized [132]. A small excess of light chain production could be due to imbalance in translation or transcription within the clone or a possible suppression of heavy chain synthesis in the clone. In other instances, heavy chain is not produced by the plasma cell and only excessive quantities of light chains are detected (“light chain multiple myeloma”) [133]. Finally, a small proportion of myeloma cells do not secrete either heavy or light chains in detectable amounts, whether because of a simple failure of synthesis or a blocking of secretion, and these are called nonsecretory. In heavy chain disease, portions of the heavy chains of IgG, IgA, or IgM are present in serum or urine.
Laboratory Methods for Study of Monoclonal Proteins
Analysis of the serum or urine for monoclonal proteins should be performed with a sensitive, rapid, and dependable screening method to detect and quantitate the presence of a monoclonal protein and a specific assay to identify it according to its heavy chain class and light chain type [134–137]. Electrophoresis with high-resolution agarose gel is preferred for screening and should be done in conjunction with serum FLC quantification [138, 139]. After electrophoresis, immunofixation should be used to confirm the presence of a monoclonal protein and to distinguish the immunoglobulin heavy chain class and its light chain type. Due to their higher sensitivity for obscured abnormalities and monoclonal FLC, immunofixation and quantitative FLC also should be done if multiple myeloma, macroglobulinemia, primary amyloidosis, or a related disorder is suspected [137–139], even if the agarose gel electrophoresis does not show a localized band or spike.
Analysis of Serum for Monoclonal Proteins
Electrophoresis
Serum screening for monoclonal proteins should be done when multiple myeloma, macroglobulinemia, or amyloidosis is suspected. Which test(s) to perform depends on which disease process one is considering. For example, at a minimum, serum protein electrophoresis and serum immunoglobulin FLC is indicated when symptoms or signs of multiple myeloma are present, that is, unexplained weakness or fatigue, anemia, elevation of the erythrocyte sedimentation rate (ESR), back pain, osteoporosis or osteolytic lesions or fracture, immunoglobulin deficiency, hypercalcemia, Bence Jones proteinuria, renal insufficiency, or recurrent infections [136, 137, 139]. If one suspects immunoglobulin light chain amyloidosis, serum and urine protein electrophoresis with immunofixation and serum immunoglobulin FLC should also be performed in the setting of an adult with peripheral neuropathy, carpal tunnel syndrome, refractory congestive heart failure, nephrotic syndrome, orthostatic hypotension, or malabsorption.
A normal serum protein electrophoresis gel and its densitometric tracing (Fig. 29.8) illustrates the separation of serum proteins. The constituents of the major components of the serum electrophoretic pattern are listed in Table 29.4. Immunoglobulins (IgG, IgA, IgM, IgD, and IgE) make up the majority of the γ-component, but it must be emphasized that they are found in the β–γ and β-regions also and that IgG molecules may electrophorese to the α2-globulin region. Thus, an IgG monoclonal protein may be found to range from the slow γ to the α2-globulin region. In normal individuals, the gamma fraction will have a smooth, gaussian shape reflecting the sum of the many normal immunoglobulins.
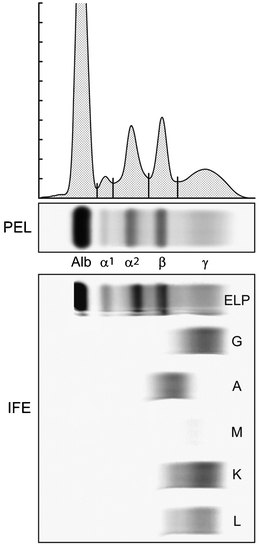
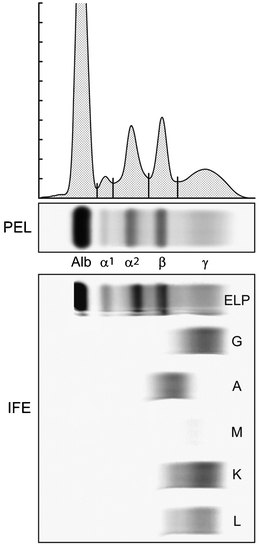
Fig. 29.8
The upper portion shows a normal serum protein electrophoresis agarose gel (PEL) as well as the densitometry tracing of the stained gel fractionated into five regions. The bottom portion shows immunofixation electrophoresis (IFE) of the same sample with antisera to γ, α, and μ heavy chains and κ and λ light chains. Note the smooth distribution of the immunoglobulins throughout the PEL gamma fraction
Table 29.4
Constituents of the major components of the serum electrophoretic patterna
+ (Anode) Albumin | α1-Globulin | α2-Globulin | β-Globulin | – (Cathode) γ-Globulin |
---|---|---|---|---|
Albumin | α1-Antitrypsin | α2-Macro-globulin | β-Lipoprotein | IgG |
α1-Lipoprotein | α2-Lipoprotein | Transferrin | IgA | |
α1-Acid glycoprotein | Haptoglobin | Plasminogen | IgM | |
(orosomucoid) | Ceruloplasmin | Complement | IgD | |
Erythropoietin | Hemopexin | IgE |
A decrease in albumin and increases in α1– and α2-globulins, and occasionally in γ-globulin, are nonspecific features of inflammatory processes (tissue inflammation and destruction) such as infection or metastatic malignancy. Rarely, two albumin bands (bisalbuminemia) may be found. This familial abnormality produces no symptoms [140].
Hypogammaglobulinemia (γ-globulin less than 0.6 g/dL) is characterized by a definite decrease in the gamma component, and the diagnosis should be confirmed by quantitative determination of the immunoglobulin levels. Hypogammaglobulinemia is seen in about 10 % of patients with multiple myeloma and most of these also have a large monoclonal protein (Bence Jones) in the urine. Approximately one-fifth of patients with primary systemic amyloidosis have hypogammaglobulinemia.
An absence of the α1-globulin band is usually attributable to a congenital deficiency of α1-antitrypsin and may be associated with recurrent pulmonary infections and chronic obstructive pulmonary disease. The absence of an α1 band should be confirmed with a quantitative assessment of the α1-antitrypsin concentration.
Upon electrophoresis, a monoclonal protein (having a single heavy chain class and single light chain type) is often seen as a narrow peak (like a church spire) in the γ-, β-, or α2-region of the densitometer tracing or as a dense, discrete band on agarose gel (Fig. 29.9). By contrast, an elevation of polyclonal immunoglobulins (having one or more heavy chain types with both κ- and λ-light chains) makes a broad-based peak or broad band (Fig. 29.10). In 4 % of sera with a monoclonal protein, there is an additional monoclonal protein of different immunoglobulin class, and this situation is designated biclonal gammopathy [141].
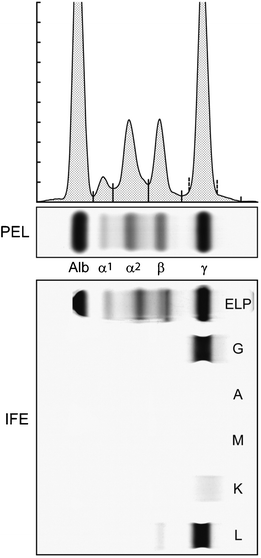
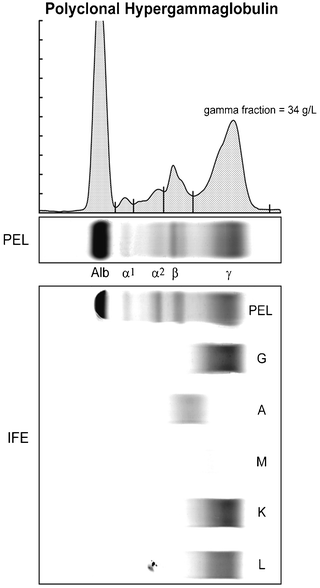
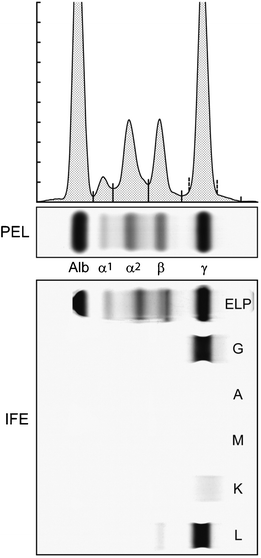
Fig. 29.9
The upper portion shows a multiple myeloma serum protein electrophoresis agarose gel (PEL) as well as the densitometry tracing of the stained gel. The bottom portion shows immunofixation electrophoresis (IFE) of the same sample with antisera to γ, α, and μ heavy chains and κ and λ light chains. Note the fractionated “M-spike” in the PEL gamma fraction and its identification as a monoclonal IgG lambda
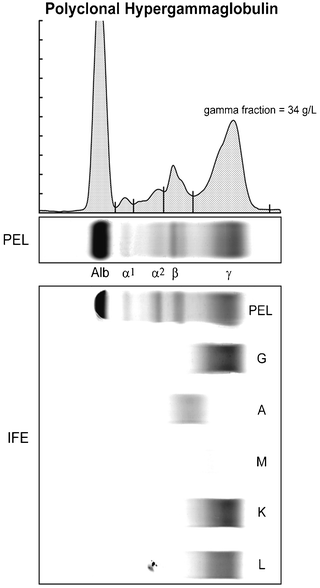
Fig. 29.10
The upper portion shows a polyclonal hypergammaglobulinemic serum protein electrophoresis agarose gel (PEL) as well as the densitometry tracing of the stained gel fractioned into five regions. The bottom portion shows immunofixation electrophoresis (IFE) of the same sample with antisera to γ, α, and μ heavy chains and κ and λ light chains. Note the elevated gamma fraction concentration and the smooth distribution of the immunoglobulins throughout the PEL gamma fraction
A tall, narrow homogeneous peak or a discrete band (monoclonal protein) is most suggestive of monoclonal gammopathy of undetermined significance, myeloma, or Waldenström’s macroglobulinemia, but monoclonal peaks may also occur in amyloidosis, cryoglobulinemia, and in lymphoma and in even rare conditions like POEMS syndrome, solitary plasmacytoma, scleromyxedema, and capillary leak syndrome.
Other features may misleadingly suggest a monoclonal protein in the serum. For example, a large, broad peak in the α2-globulin region may represent free hemoglobin–haptoglobin complexes resulting from hemolysis. The serum is often pink and should suggest the possibility of hemolysis. Immunofixation electrophoresis (IFE) will be negative, and another serum sample should be obtained from the patient. Large amounts of transferrin in patients with iron-deficiency anemia may produce a localized band in the β-region. Fibrinogen (in plasma) is seen as a discrete band between the β- and the γ-regions. When a small β–γ band is seen, the specimen should be examined for the presence of a clot. If a clot is not seen, thrombin should be added to the sample; this produces a clot if fibrinogen is present. If the discrete band can no longer be detected when electrophoresis is repeated, the presence of fibrinogen is established.
Certain other conditions have distinctive electrophoretic patterns. The nephrotic syndrome produces a serum pattern featuring decreased albumin and γ-globulin and increased α2– and β-globulins. The increased α2 and β-globulin may look like a monoclonal peak of rapid mobility and might be mistaken for a myeloma protein. The urinary pattern seen in a patient with nephrotic syndrome consists mainly of albumin.
Chronic infections, connective tissue diseases, and chronic liver diseases may be characterized by a large, broad-based polyclonal pattern (Fig. 29.10). This pattern is common in chronic active hepatitis, where the γ-component may be 4 or 5 g/dL or greater [142]. This large, broad γ-band may be confused with that seen in multiple myeloma or macroglobulinemia. Occasionally, lymphoproliferative processes may have a large polyclonal increase in immunoglobulins. We have seen a number of patients in whom no underlying disease is apparent and in whom the polyclonal increase in immunoglobulins persists for years.
A monoclonal protein may be seen as a rather broad band on the agarose gel or a broad peak in the densitometer tracing and can be mistaken for a polyclonal increase in immunoglobulins. Presumably, this broad band or peak is the result of a complex of monoclonal protein with other plasma components, aggregates of IgG, polymers of IgA, or dimers of IgM. IFE is required for unambiguous identification as a monoclonal protein [143].
It must be emphasized that a patient can have a monoclonal protein when the total protein concentration, β- and γ-globulin levels, and quantitative immunoglobulin values are all within normal limits. A small monoclonal peak may be concealed among the β- or γ-components and thereby be missed by serum protein electrophoresis. A monoclonal light chain (Bence Jones proteinemia) is often not seen on serum protein electrophoresis. In a number of cases of IgD myeloma, the monoclonal protein appears small or is not evident at all. Often the monoclonal protein is not apparent in the heavy chain diseases. In fact, the serum protein electrophoretic pattern is normal in half the cases of α-heavy chain disease, and an unimpressive broad band in the α2– or β-region is the only electrophoretic abnormality among the rest. The characteristic sharp band or peak suggestive of a monoclonal protein is never seen. In μ-heavy chain disease, the electrophoretic pattern appears normal except for hypogammaglobulinemia, and a dense band is often not apparent. In γ-heavy chain disease, a localized band is usually seen in the β–γ region, but often it is broad, appears heterogeneous, and is more suggestive of a polyclonal than a monoclonal protein. Thus, a normal value for the components of the electrophoretic pattern or a normal-appearing or nonspecific pattern may still contain a monoclonal protein, and consequently IFE is critical.
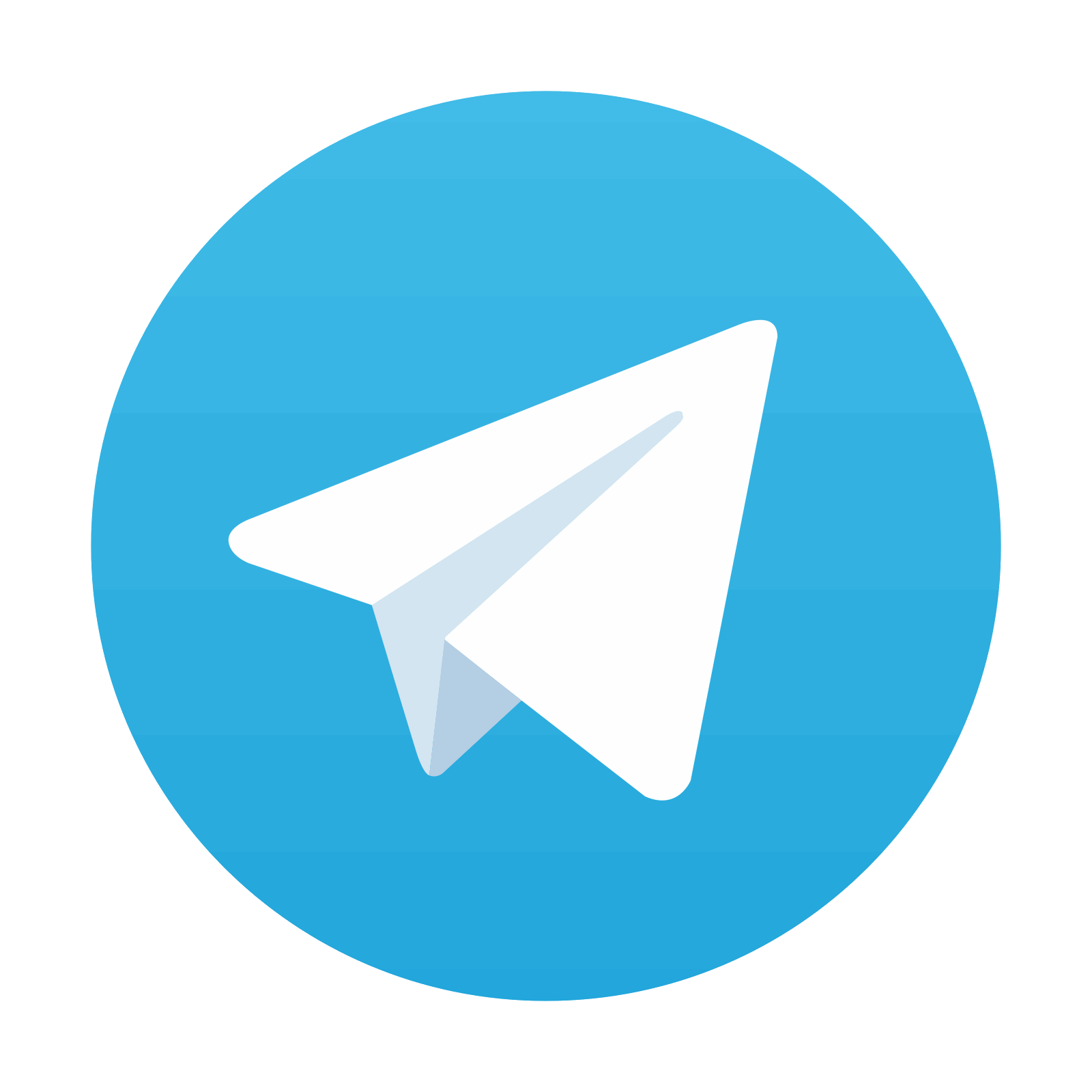
Stay updated, free articles. Join our Telegram channel

Full access? Get Clinical Tree
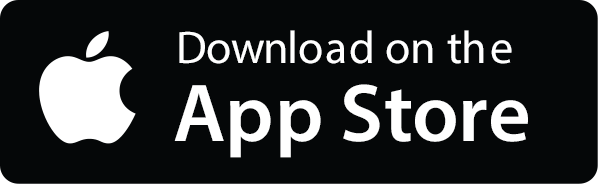
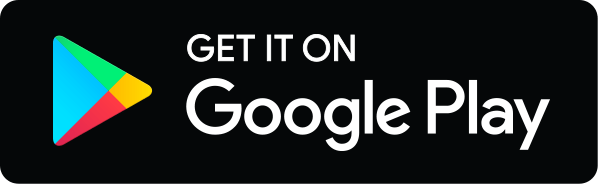
