Table 5.1 Basic Tissue and Tumor Characteristics on Contrast-Enhanced CT | |||||||||||||||||||||
---|---|---|---|---|---|---|---|---|---|---|---|---|---|---|---|---|---|---|---|---|---|
|
typically between -1,000 and +3,000 HU. Increased iodine content of a tissue also results in increased density of that tissue, which forms the basis of contrast-enhanced CT images.
Commonly used display windows are also usually preprogrammed using different function keys for easy and rapid access.
diagnostic quality of the examination. This results in increased propensity to motion artifact, particularly below the hard palate, where there can also be image degradation secondary to swallowing artifact. A more detailed comparison of the two techniques will be provided later.
to motion artifact, which can degrade image quality. Therefore, the MRI protocols are designed carefully and optimized to achieve the best result taking into account these variables. When detailed evaluation of a small area of interest, such as skull base foramina, is required, this is best performed as a targeted exam focusing on the area of interest, rather than an evaluation of the entire neck, if possible. An optimal examination is designed to achieve reasonable scan times that can be tolerated by the patient and also enable acquisition of high-quality images without significant motion degradation and image distortion.
Table 5.2 Basic MRI Sequences and Tissue Characteristics | |||||||||||||||||||||||||||||||||||
---|---|---|---|---|---|---|---|---|---|---|---|---|---|---|---|---|---|---|---|---|---|---|---|---|---|---|---|---|---|---|---|---|---|---|---|
|
MRI for cancers below the level of the hard palate. CT is also a safer environment for the evaluation of acutely ill patients or patients with respiratory difficulties who would have difficulty lying still in the supine position for a prolonged period of time.
![]() Figure 5.9. Tumor appearance on STIR images. STIR image is shown from the same case displayed in Figure 5.6. Note the bright signal of the tongue cancer (T) compared to adjacent tissues. Fluid, such as that of cerebrospinal fluid (CSF), is very bright on STIR. |
Table 5.3 Comparison of Relative Strengths of CT and MRI for Head and Neck Cancer Imaging | ||||||||||||
---|---|---|---|---|---|---|---|---|---|---|---|---|
|
which in turn can be detected and localized with a PET scanner. When integrated with an anatomic technique like CT, this provides a robust functional evaluation while enabling accurate anatomic localization, which is very important given the complex anatomy in the head and neck. Therefore, current standard practice is to perform a combined PET/CT for evaluation of head and neck cancer.13,14 Most PET/CTs for head and neck cancer are performed using the radiopharmaceutical 2-18F-fluoro-2-deoxy-D-glucose (FDG), a glucose analog that is taken up by cells but is not metabolized.13,14 The rationale for FDG-PET cancer imaging is that in general, cancer cells have greater uptake on PET than do normal tissues (known as Warburg effect) (Fig. 5.10). However, one must take into account that increased FDG uptake is not specific to cancer cells and may be seen in context of inflammation/infection including biopsy sites, some benign neoplasms, or increased muscular activity under certain circumstances. This needs to be taken into account when interpreting PET scans; to be discussed later.
performed in 199319 and has been used by some for evaluation of head and neck cancer.20 Therefore, although it is useful as a reference for potential pathology, there is no clear evidence that this threshold can be extrapolated to lymph nodes or tissues and lesions outside the thorax. For example, others have used an SUV of 3.5 to 4 as threshold for evaluation of lymphadenopathy,21 and one study showed that the greatest specificity for determination of metastatic nodal disease in squamous cell carcinoma (SCC) was achieved when a threshold of 5 was used.22 As discussed by Escott,13 another pitfall of using strict SUV criteria for determination of lymphadenopathy is that small pathologic lymph nodes may have an SUV value below an accepted threshold and thus be visually difficult to call abnormal. This highlights the importance of using the SUV as a guide, rather than absolute determining value, and carefully correlating with findings on the contrastenhanced CT for determination of pathologic lesions and lymphadenopathy.
when necessary help with biopsy planning. Imaging can also be helpful in clinically misleading presentations, and sometimes the radiologist is the first to suggest the presence and site of a head and neck cancer (Fig. 5.14). However, frequently, at the time of initial evaluation, the diagnosis has already been made, and the main role of imaging is to stage the tumor. The added value of imaging in that scenario is typically to upstage the clinical assessment by identifying involvement of critical structures, lymph nodes, or distant metastases that are not reliably identified clinically. Imaging can also guide biopsy when there are potentially important equivocal findings. Furthermore, imaging plays a key role in follow-up and surveillance of cancers to evaluate response to treatment, progression of disease, and tumor recurrence.
![]() Figure 5.12. Pitfalls and false positives in PET: lymphoid tissues of the Waldeyer ring. Axial contrast-enhanced CT scan (A) and fused PET image (B) are shown from a 52-year-old with biopsy-proven squamous cell carcinoma of the right base of the tongue. Similar to the case in Figure 5.11, there is diffuse uptake at the base of the tongue without clear focally increased uptake at the site of tumor on the right (long arrow). On CT, there is asymmetric enlargement of the right base of tongue tissues (long arrow). Although by itself this is insufficient for a confident diagnosis, it is useful for directing the biopsy, which demonstrated cancer at that site. Note the large, partly necrotic, right level II pathologic nodal mass with diffusely increased uptake on PET (short arrows). |
of intrinsic contrast on both CT and MRI and is clearly distinguishable from soft tissue characteristics of most nonlipomatous tumors. Careful evaluation of infiltration and obliteration/asymmetry in the fat within and fat planes separating various structures and spaces in the neck will enable identification of small tumors and areas of tumor infiltration (Fig. 5.14). Familiarity with the detailed anatomy of the neck is an essential asset to help evaluation. Disruption of normal tissue architecture is also important for evaluating tumor infiltration. For example, the preserved striated architecture of denervated muscle should enable
distinction from tumor invasion despite the abnormal signal (Fig. 5.8). By taking into account all the different characteristics, an optimal imaging evaluation and lesion characterization can be performed. Information from the PET scan complements the anatomic information provided on CT and MRI and can increase sensitivity for detection of tumor, lymphadenopathy, and recurrence in head and neck squamous cell carcinoma (HNSCC).13
![]() Figure 5.17. Superficial invasion of the mandibular cortex with reactive marrow edema on MRI. Axial STIR (A), coronal contrastenhanced fat-suppressed T1w (B), axial T1w (C), and coronal T2w (D) MRI images are shown from the same patient whose CT is shown in Figure 5.4. Corresponding to the small focal cortical break seen on CT, there is a potential defect (thin arrow), although the MRI is less convincing than the CT. There is also mildly increased signal within the marrow (thick arrow) on the STIR (A) and contrast-enhanced (B) images, demonstrating the increased sensitivity of MRI for detecting subtle marrow changes. However, in this case, the fat within the marrow is preserved on the T1w image (C; thick arrow) and the signal is normal on the T2w image (D; thick arrow). This suggests that the mild signal abnormality represents reactive marrow edema and not true marrow invasion. Pathology confirmed superficial cortical invasion without marrow invasion. |
arch. Level IV nodes are internal jugular chain nodes that extend from the level of the lower margin of the cricoid cartilage arch to the level of the top of the manubrium. Level V nodes are posteriorly located lymph nodes that are subdivided into levels VA and VB. Level VI nodes are the visceral nodes, and level VII nodes are those that lie caudal to the top of the manubrium, located between the medial margins of the left and right common carotid arteries in the substernal region45,46,47 (Fig. 5.19; Table 5.4). Please refer to Table 5.4 for a detailed description of the anatomic landmarks used for the imaging classification.
![]() Figure 5.18. Increased sensitivity of MRI for determination of invasion of the bone marrow compared to CT. Axial CT displayed in bone windows (A) and unenhanced T1w (B), T2w (C), and contrast-enhanced fat-suppressed T1w (D) MRI images are shown from a patient with an advanced squamous cell carcinoma of the retromolar trigone (T) with invasion of multiple adjacent spaces including the buccal space, masticator space, and oropharynx. On CT, the cortical invasion of the left mandible is subtly evident (arrowhead). However, the marrow invasion is not clearly demonstrated. On the MRI, however, there is clear invasion of the marrow with replacement of the normal marrow fat in the mandibular ramus with tumor (white arrow). Note the difference compared to the normal marrow of the contralateral mandibular ramus, particularly well seen on the unenhanced T1w image (B, black arrow). Unlike reactive marrow edema shown in Figure 5.17, the marrow signal abnormality follows the signal of large extraosseous tumor mass on all sequences, a key feature for differentiating the two on imaging. |
Table 5.4 Landmarks Used for Cervical Lymph Node Localization Using the Imaging-Based Classification | |||||||||||||||||||||||||||||||||||||||||||
---|---|---|---|---|---|---|---|---|---|---|---|---|---|---|---|---|---|---|---|---|---|---|---|---|---|---|---|---|---|---|---|---|---|---|---|---|---|---|---|---|---|---|---|
|
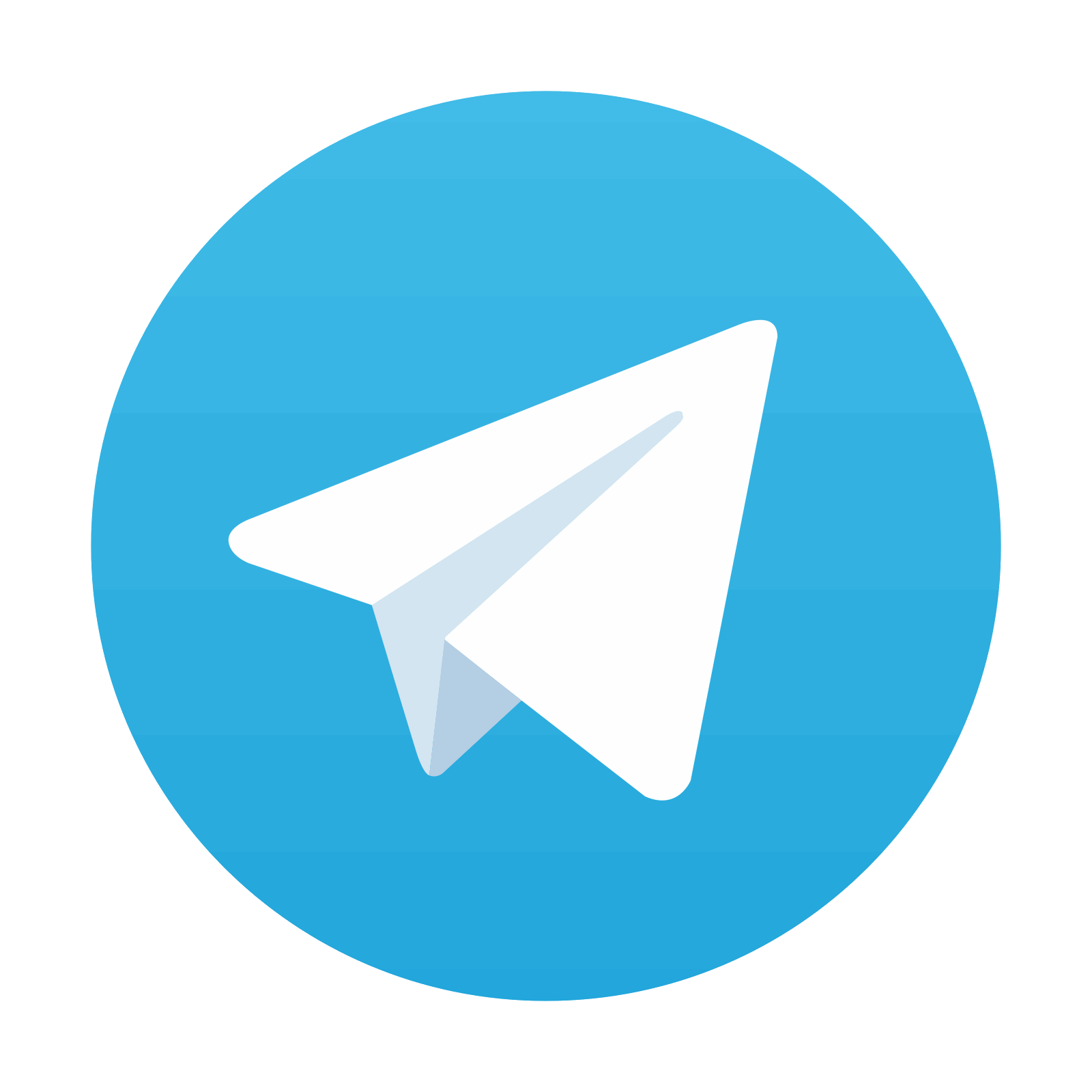
Stay updated, free articles. Join our Telegram channel

Full access? Get Clinical Tree
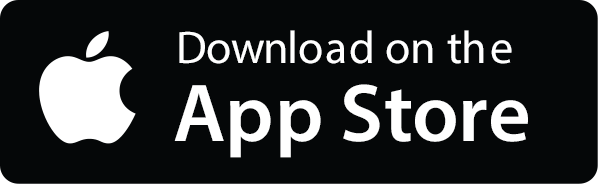
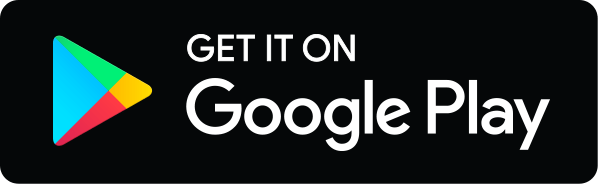
