20 Hyperthermia
General Remarks
There are two types of heat application: active hyperthermia, which provokes fever episodes by means of pyrogen administration, and passive hyperthermia, which raises the temperature of tissues or body fluids by various physical means.
The classic, pyrogen-induced fever therapy with target temperatures between 39–40 °C results in enhanced migration of leukocytes and monocytes from the peripheral blood into the tissues and also improves chemotaxis and activates phagocytosis. In addition, it activates metabolic processes, enhances the blood supply, and potentiates the effects of some cytostatic agents.
Passive hyperthermia (Tables 20.1 and 20.2) has different results depending on the range of temperatures:
• Moderate hyperthermia has immunological effects similar to fever; it also increases membrane permeability and alters the blood supply within the tumor tissue. Synergistic effects with certain cytostatic agents in vitro and in vivo have been reported.
Table 20.1 Subdivision of hyperthermia according to temperature ranges
37.5–38.5 °C | Subclinical hyperthermia |
38.5–40.5 °C | Moderate hyperthermia |
40.5–41.5 °C | Intermediary hyperthermia |
41.5–43.0 °C | Extreme hyperthermia |
>43.0 °C | Thermotherapy |
Table 20.2 Mechanisms of action of hyperthermia
• Cytostatic/cytotoxic effects through metabolic changes and denaturation of molecular structures (e. g, enzymes)
• Induction of apoptosis through heat and through hyperacidity caused by metabolites that are not sufficiently eliminated by the blood stream
• Inhibition of cellular repair mechanisms
• Increased permeability of cell membranes for chemotherapeutic agents
• Synergistic effects with radiation therapy and chemotherapy (additive and potentiating)
• Nonspecific immunostimulation and, possibly, induction of a specific immune response mediated by HSP-TAA
• Alleviation of pain by blocking pain receptors
• Extreme hyperthermia has direct cytostatic and cytotoxic effects, especially within the tumor tissue, and produces synergistic effects with cytostatic agents.
In principle, hyperthermia can be applied to all solid malignant tumors. Temperatures above approximately 42 °C have cytostatic or cytotoxic effects on cells in general. In malignant tissues, however, heat seems to be cytotoxic already at lower temperatures because of the altered microenvironment. Damages to membranes of the endoplasmic reticulum, to the cytoskeleton, and to the mitotic spindle are induced already at temperatures in the range of 41–42 °C. In addition, hyperthermia causes the induction of apoptosis.
During passive hyperthermia, the tumor tissue is warmed up by means of external energy sources. The temperature range differs depending on the type of application:
– 39–40 °C for moderate whole body hyperthermia
– 41–42 °C for extreme whole body hyperthermia
– 42–43 °C for superficial or deep hyperthermia
– 46–58 °C for transurethral hyperthermia.
Hyperthermia must be distinguished from thermoablative methods. These procedures induce coagulation necrosis at temperatures of above 90 °C. They are usually performed with interstitial techniques, but also with ultrasound and alternating magnetic fields.
The thermosensitivity of tumors depends on internal cellular factors and on the cellular environment (e. g., energy deficit, or low pO2 and low pH). At low pH and low oxygen saturation, cells are more sensitive to higher temperatures. This might explain why malignant tissues are more sensitive to heat than healthy tissues with a normal blood supply. In addition to specific conditions of physical absorption and the altered microenvironment, the tumor’s blood vessels are probably less capable of thermoregulation. This creates higher temperatures within the tumor and stimulates some of the tumor cells to produce heat shock proteins (HSP). These proteins are then expressed on the cell membrane together with tumor-associated antigens (HSP-TAA), thus increasing the cell’s antigenicity and inducing additional local immuno-reactions that contribute to the destruction of the tumor cells (10, 14, 16). Depending on the temperature and duration of treatment, there may also be some regional overheating with increased blood flow in the healthy tissue surrounding the tumor, thus causing a reduction in the tumor’s blood supply.
Hyperthermia Combined with Radiation Therapy or Chemotherapy
The main problem with the sole application of high temperatures as cancer therapy lies in the technical area, i. e., in reaching sufficiently high and homogenous temperatures in tumors of well-vascularized organs or of deep location, and also in the prevention of so-called hot spots in healthy tissues.
In addition to having direct cytotoxic effects on the tumor tissue and making the tumor tissue more sensitive to chemotherapy or radiation therapy (e. g., by increasing membrane permeability), high temperatures also increase the cytotoxic effects of cytostatic agents and ionizing radiation. Hence, it makes sense to combine these methods. There are also clinical indications that the resistance to chemotherapy can be overcome by heat. The combined therapies may be performed simultaneously, or sequentially at defined alternating intervals.
The cytotoxic effects of hyperthermia are essentially based on molecular and metabolic changes in the cells. Due to the breakdown of molecular structures, the cells become more sensitive to heat not only during mitosis but also during S phase. This might explain the better response rate when radiation therapy and hyperthermia are combined—in addition to the changes in metabolic processes and blood supply–because cells in S phase and mitosis are certainly resistant to radiation. However, they are also especially thermosensitive. Another explanation for the potentiating effect of this combination therapy might be a thermal inhibition of the repair processes following radiation-induced DNA damage. In addition, hyperthermia is first of all effective in hypoxic areas, whereas radiation is more effective in oxygen-rich areas.
The sensitizing effect of hyperthermia on chemotherapy depends on the type of the cytostatic agent and on the temperature. The improved blood supply resulting from the initial hyperthermia in tumor and surrounding tissue may lead to an increased permeability of cell membranes, thus increasing the concentration of the cytostatic within the tumor. However, there are also known temperature-dependent changes in the intracellular biotransformation of various cytostatics (e. g., gemcitabine, capecitabine). Alkylating agents, platinum complexes, and anthracyclines (such as doxorubicin) are currently regarded as the ideal cytostatic agents in combination with hyperthermia (17). However, for most antimetabolites (such as vinca alkaloids and taxines), there are so far no indications of synergistic effects. Supplementary effects may also be expected from the fact that vascularized tumor regions are more accessible to cytostatic chemotherapy, whereas less vascularized hypoxic areas respond better to hyperthermia.
Generation of Hyperthermia
The best effects for cancer therapy are achieved at temperatures above 41.5 °C. In order to reach the temperature required in the target region, various technically expensive procedures have been developed in recent years (Table 20.3) It is only through these procedures that the clinical application of hyperthermia has become possible. Today, hyperthermia is mainly generated by infrared radiation, radio waves, microwaves, ultrasonic waves, or alternating magnetic fields (Table 20.4🙂
Table 20.3 Subdivision of hyperthermia according to size and location of the overheated body area
Hyperthermia procedure | Size and location of tumor |
Local hyperthermia (LHT) [or superficial hyperthermia (SHT)] | Superficial skin tumors or metastases |
Interstitial hyperthermia (IHT) | Direct overheating of tumors by implantation of applicators |
Regional hyperthermia (RHT) [or deep hyperthermia (DHT)] | Deep location of organ tumors or metastases |
Perfusion hyperthermia (PHT) | Hollow organs or extremities with disseminated metastases |
Whole body hyperthermia (WBHT) | Generalized metastases |
Interstitial thermotherapy (ITT) | Locally targeted tumor therapy (e. g., prostate cancer, ENT tumors, liver metastases) |
Table 20.4 Subdivision of hyperthermia according to energy source
Hyperthermia procedure | Energy source |
Superficial hyperthermia (SHT) | Infrared A1, short waves/microwaves |
Deep hyperthermia (DHT) | Short waves/decimetric waves, ultrasound |
Perfusion hyperthermia2(PHT) | Heat convection, extracorporal heat exchange |
Whole body hyperthermia (WBHT) | Infrared A, extracorporal heat exchange |
Interstitial thermotherapy (ITT) | Antennas, ferromagnetic particles |
Magnetic fluid hyperthermia (MFH) | Magnetite, magnetic alternating field |
1 Water-filtered irradiation with infrared A;
2 intracavitary perfusion or perfusion of extremities
• Infrared radiation Infrared A (IRA) radiation is generally used because it penetrates better into the tissue. In order to prevent skin burns resulting from intense absorption of some of the IRA radiation by water molecules in the uppermost layers of the skin, water-filtered infrared A (wIRA) radiation is sometimes applied. The most important fields of IRA application are superficial hyperthermia (e. g., Hydrosun irradiator) and whole body hyperthermia (e. g., IRATHERM 2000; Heckel hyperthermia bed).
• Radio waves Radio frequency hyperthermia (RF-HT) is usually used for tumors in deeper locations, because short waves penetrate better into the tissue (5–10 cm) than waves of higher frequencies. Depending on the frequency, energy transmission occurs by means of capacitive or radiative applicators. Radio waves that are used frequently for therapeutic purposes operate in the range of 8–27 MHz. By selecting certain frequencies and, thereby, determining the interactions between the electromagnetic field and cellular structures, it may be possible to achieve also direct cytostatic (nonthermal) effects. For example, the dielectric constant is approximately 10–30 times higher in tumors than in healthy tissues (6), and the electric conductivity in tumors is up to 100 times higher (11), which may be important especially when applying short waves.
• Decimetric waves Some devices for deep hyperthermia operate in the frequency range of 400–912 MHz. By using several radiative applicators, the temperature can be selectively increased to a predetermined range by phase shift. However, the higher the frequency, the lower the penetrating power, thus resulting in increased heterogeneity of temperature distribution (hot spots).
Clinical Application
Local Hyperthermia
The aim of local hyperthermia is to transmit thermal energy into the tumor while avoiding the healthy tissue as much as possible. Noninvasive approaches are only possible for superficial tumors by means of infrared radiation, laser light, or ultrasonic waves (Fig. 20.1) The combination of photosensitizing substances with point-source laser therapy or water-filtered infrared radiation is promising for small, superficial malignant skin alterations. Superficially spreading recurrences can be treated by methods using flexible applicators (e.g., spiral microstrip antennas). There are findings of phase I and phase II clinical trials on the treatment of superficial tumors by radiation therapy combined with hyperthermia (18).
Fig. 20.1 Device for superficial hyperthermia.
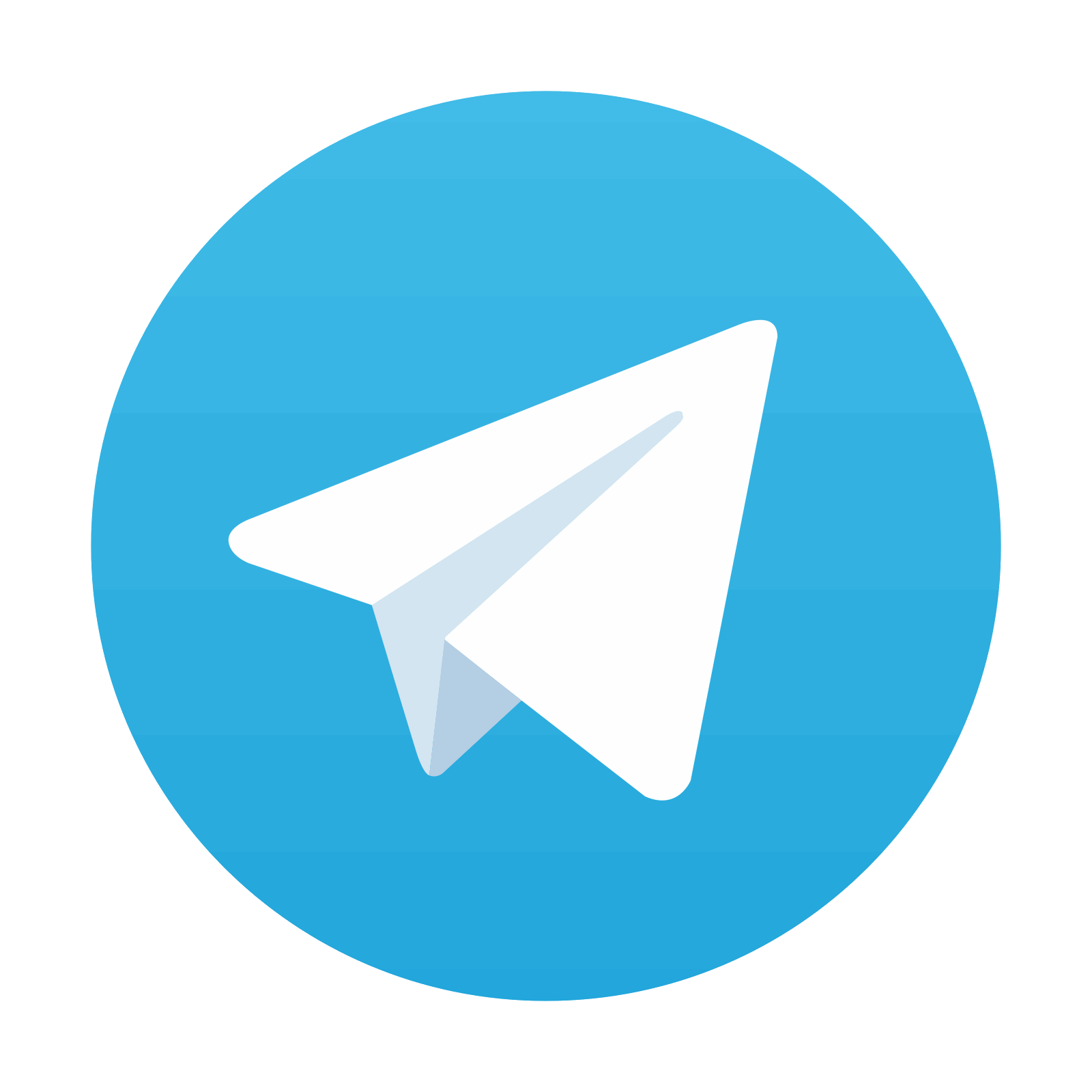
Stay updated, free articles. Join our Telegram channel

Full access? Get Clinical Tree
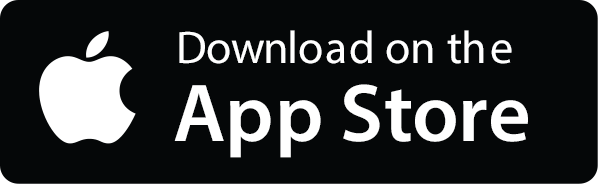
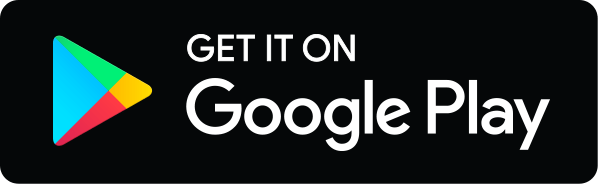