and Winfried G. Rossmanith2
(1)
Lenzkirch, Germany
(2)
Ettlingen, Germany
6.1 Introduction
6.2.1 JH Synthesis
6.3.1 Introduction
6.3.3 Physiology
6.4 Steroids
6.5.2 3β-HSD
6.5.4 17β-HSD
6.5.5 5α-Reductase
6.5.6 CYP21
6.5.7 CYP11B1
6.5.9 CYP19
6.5.10 Concluding Remark
6.6 Progesterone
6.7 Sex Hormones
6.7.1 Gonadal Development:
6.8 Corticoids
6.8.1 Cortisol
6.8.2 Aldosterone
6.10.2 Steroids in Plants and Fungi
6.11.1 Synthesis of Vitamin D3
6.11.2 Vitamin D Uptake from Food
6.1 Introduction
With mevalonic acid as the common origin in vertebrates and invertebrates, a variety of hormones is synthesized from isoprenyl oligomers, squalene and cholesterol. Most relevant for vertebrates are steroid hormones based on the polycyclic sterane frame. In arthropods, however, noncyclic juvenile hormones are derived from farnesoate or farnesoic acid methyl esters and ecdysteroids are based on cholesterol.
All the metabolic steps involved depend on a variety of enzymes. These enzymes are not present in the same cells, in the same cellular compartment; steroidogenic enzymes are expressed in different cells and targeted individually to predetermined cellular compartments. This is exemplified in the hormone interplay of mother and fetus where some precursors have to cross the placental barrier in order to become fully metabolized.
The identification of steroid hormone in, for example, some mussel extract does not at all argue for a biosynthesis of this hormone by the mussel itself; it could have been taken up with the food. Likewise is the finding of modification by testosterone using mussel tissue no argument for testosterone formation by the mussel. This finding only says that steroids such as testosterone or related molecules might be modified by an enzyme from the mussel. For testosterone biosynthesis from cholesterol there are at least five enzymes necessary, at least in vertebrates, and none of these has ever been identified in molluscs. This said, there is no formal proof of testosterone or estradiol biosynthesis apart from vertebrates. The necessary enzymes are missing in invertebrates at least in those fully sequenced thus far and the isolation of biosynthetic intermediates has not been published.
To give the full image, we present the juvenile hormone and steroid-forming enzymes, their topological distribution, the hormone structures, and the actions of individual hormones. In addition ecdysones and some plant and fungal steroids are listed.
6.2 Pathways to Juvenile Hormone and Steroids
6.2.1 JH Synthesis
The starting molecule for juvenile hormone (JH) and steroid biosynthesis (Schooley and Baker 1985) is acetyl-coenzyme A (acetyl-CoA) which is converted by the acetoacetyl-CoA-thiolase into acetoacetyl-CoA and by the action of 3-hydroxy-3-methylglutaryl-CoA synthase into (HMG-S) hydroxymethylglutaryl-CoA. From this substance the 3-hydroxy-3-methylglutaryl-CoA reductase (HMG-R) generates mevalonate (1; Fig. 6.1). With the mevalonate kinase, the phosphomevalonate kinase and the diphosphomevalonate decarboxylase isopentenyl-pyrophosphate is generated (isopentenyl-PP; 4).
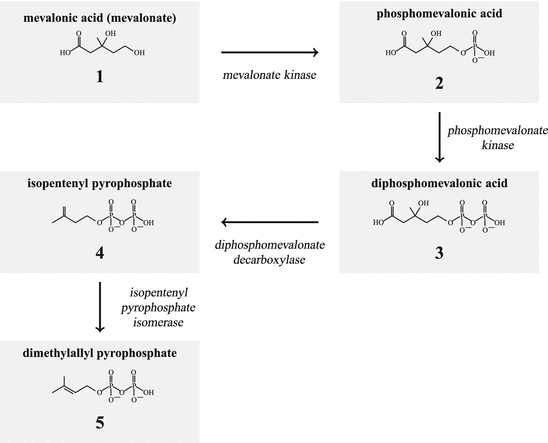
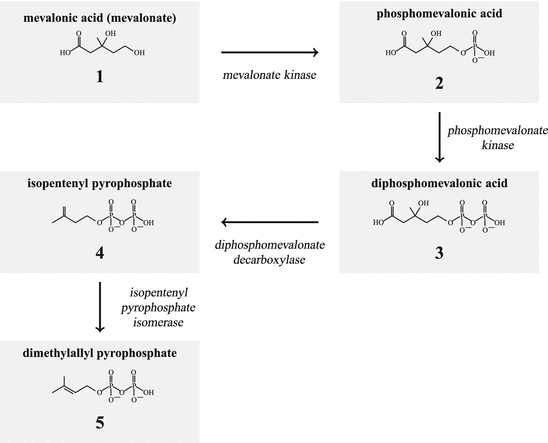
Fig. 6.1
Synthesis of isopentenyl pyrophosphate and dimethylallyl pyrophosphate
The isopentenyl-isomerase generates finally dimethylallyl-PP (5, Figs. 6.1 and 6.2). From dimethylallyl-PP plus isopentenyl-PP geranyl-PP (6) is derived. Using this geranyl-PP plus isopentenyl-PP the farnesyl synthase builds farnesyl-PP (7; Fig. 6.2 ).
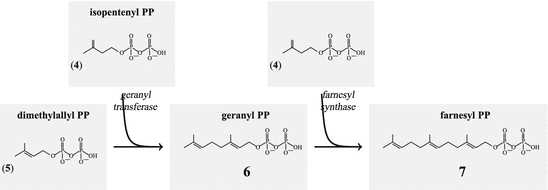
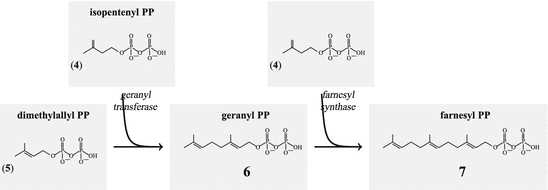
Fig. 6.2
Stepwise addition of isopentenylpyrophosphate generates farnesyl pyrophosphate
During the JH biosynthesis farnesyl-PP is hydrolyzed to farnesol (8; Fig. 6.2), which is then oxidized to farnesal (9) and finally to farnesoic (aka farnesenic) acid (10). By the JH-methyl transferase the methyl farnesoate (11) is made and by the JH-epoxidase oxidized to the juvenile hormone (13). Alternatively farnesoic acid can be oxidized to the JH III acid and later oxidized to juvenile hormone.
6.2.2 From Farnesyl Pyrophosphate via Squalene to Cholesterol
For steroid generation two farnesyl-PP are coupled by the squalene synthase to squalene (14; Fig. 6.4). After epoxidation this squalene is rearranged by the oxido-squalene synthase to lanosterol, which now has the four cyclic rings characteristic for steroids. Lanosterol is oxidized by several oxidation steps to cholesterol, the base of all steroids, or to another compound-like vitamin D3. Dimethylcholestatrienol (17), made in the ovarian follicle by the lanosterol-14
-demethylase (CYP51), is a paracrine stimulator of ovum maturation. From 7-dehydrocholesterol (18) branches off the biosynthesis of vitamin D3 and related compounds (Fig. 6.4).
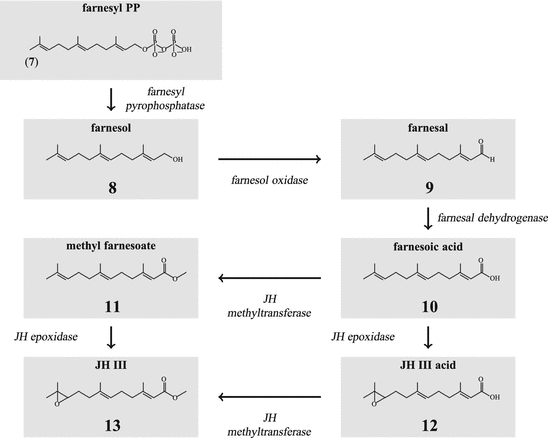
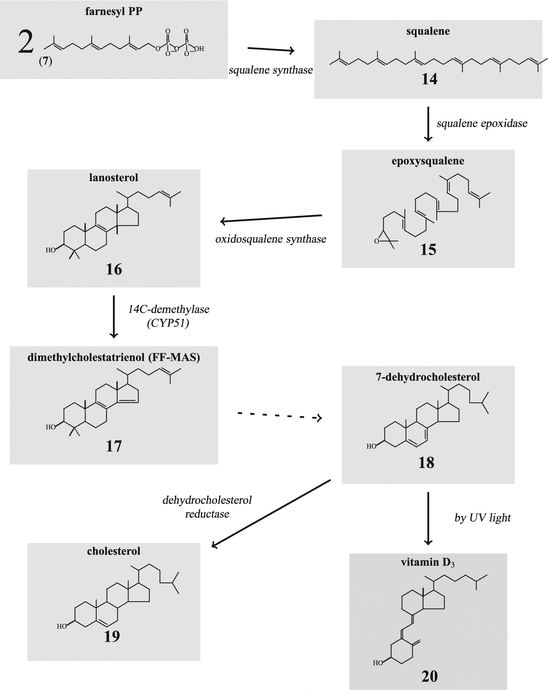

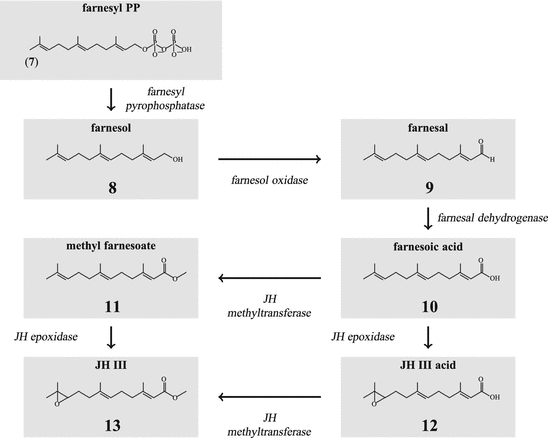
Fig. 6.3
Synthesis of juvenile hormone (JH) III from farnesyl pyrophosphate
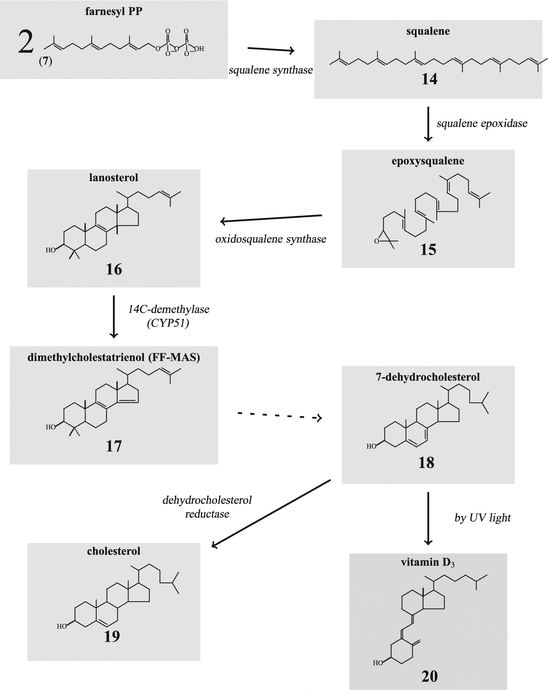
Fig. 6.4
Synthesis of cholesterol from squalene. FF-MAS follicular fluid meiosis-activating sterol
The 14
-demethylase is the cytochrome P450 enzyme (CYP51) which has been found with identical function in all living phyla: in bacteria, plants, and animals.

JH and steroids are terpenes: monoterpenes are derived from two isopentenyl building blocks, sesquiterpenes1 such as farnesol of 15 carbon atoms; squalene or lanosterol are triterpenes with 30 carbon atoms.
6.3 Juvenile Hormones
Fact sheet 6.1: Juvenile hormone
Synthesis and target:
JH is synthesized in the corpora allata from mevalonate. Its targets are all tissues.
Function:
In larvae JH ensures molting to an additional larval stage. In adults it supports vitellogenesis and the reproductive cycle.
Receptor:
The transcription factor methoprene-tolerant has lately evolved as the JH receptor.
6.3.1 Introduction
Juvenile hormones (JH) were discovered almost 50 years ago (Williams 1956; Goodman and Granger 2005; Adams 2003). They are the product of the corpora allata . All ecdysozoan s2 have to molt in order to grow. In insects there are, however, two different molts: first the molt between two larval stages (instars) and then the molt to the imago which involves in holometabolic insects pupation. In order to arrive at a molt, the hemolymph ecdysone must increase to high levels. The parameter to decide whether a larval molt or a imaginal molt happens is the JH3: fifth instar larvae of Psacothea hilaris , an east Asian longicorn, may molt—given sufficiently long daylight and warm temperature—to become an imago. However, when treated with JH they have to pass two additional larval molts before becoming an imago (Munyiri and Ishikawa 2004).
6.3.2 Biochemistry and Structure
The general pathway of JH biosynthesis has already been shown (Fig. 6.3) (for review see: Schooley and Baker 1985). Thus JH III is made (13). Additional JH are JH 0 (26), JH I(25), and JH II(24). These are made only in lepidopterans (butterflies and moths); JH 0 has only been found in eggs of M. sexta . Differences between these three JH and JH III arise from the usage of propionyl-CoA in addition to that of acetyl-CoA. With propionyl-CoA a homomevalonic acid with one extended side chain is formed (21). It depends on a single, double, or triple usage of the derived ethylmethylallyl- and homoisopentenyl pyrophosphate whether JH II, JH I, or JH 0 are made.
As demonstrated in Fig. 6.5 dimethylallyl-PP and isopentenyl-pp (derived from mevalonate) and the derivatives of homomevalonate, ethylmethylallyl-PP (22) and isohexenyl-PP (homoisopentenyl-PP; 23), are species-specifically utilized: animals, which make JH I or JH II, can use both acetyl-CoA and propionyl-CoA. In those synthesizing JH I, the geranyl transferase adds isohexenyl-PP to ethylmethylallyl-PP, and during JH II synthesis, isoprenyl-PP is added to ethylmethylallyl-PP. These steps distinguish JH I and JH II synthesis.
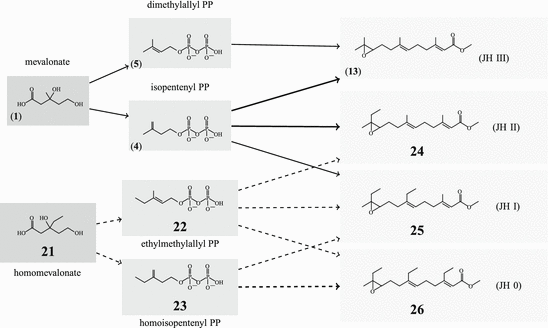
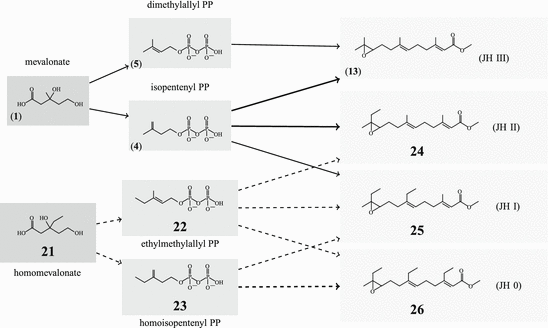
Fig. 6.5
The usage of propionyl-CoA (in Lepidoptera) leads to three additional juvenile hormones
JH diversity is further augmented by side chain hydroxylation, for example, in migratory locust (Fig. 6.6) where any of the three side-chains can be hydroxylated. 12-hydroxy-JHIII (27) tested in mealworms (Tenebrio molitor), was 100-fold more potent than JH III (Darrouzet et al. 1997).
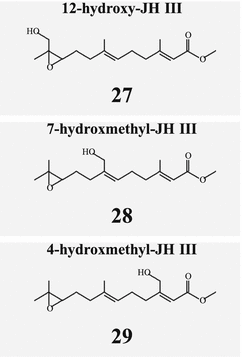
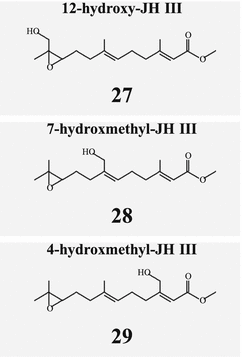
Fig. 6.6
Locusts possess hydroxylated derivatives of JH III
The juvenile hormone of D. melanogaster is a bisepoxide (31; Fig. 6.7). In a first step farnesoic acid it oxidized to 10,11-epoxyfarnesoic acid (12), and in the second step to 6,7;10,11-bis(epoxy)farnesoic acid (30 before being esterified by methyltransferase (Fig. 6.7). In such a pathway any JHIII would not be produced that had not been found in spite of a long search; very new orders of magnitude more sensitive analyses of farnesoates in D. melanogaster showed now that methyl farnesoate and the bisepoxide are present in excess in third instar larvae, whereas in the wandering stage methyl farnoseate in excess of some JHIII and almost no bisepoxide, and in the puparium only methyl farnesoate could be found (Jones et al. 2013). Only in 2009 did Kotaki et al. identify the structure of heteropteran JH as a stereoisomer of the Drosophila JH, and called this bisepoxide juvenile hormone skipped bisepoxide (JHSB3; 32). The slight difference in the enzyme specificity should help to evaluate the stereospecificity of the epoxidase.
Degradation of JH has two components: hydrolysis by an esterase to the free acid (12) and/or hydrolysis by an epoxide hydrolase to the diol derivative (Fig. 6.8). JH III diol (34)can be further phosphorylated by a kinase (35). Whether diols or diol phosphate are still biologically active has not been analyzed.
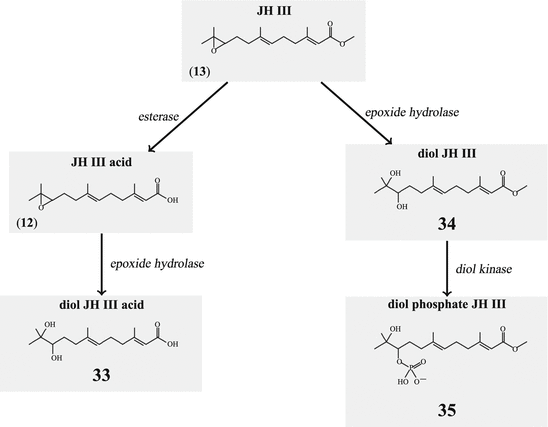
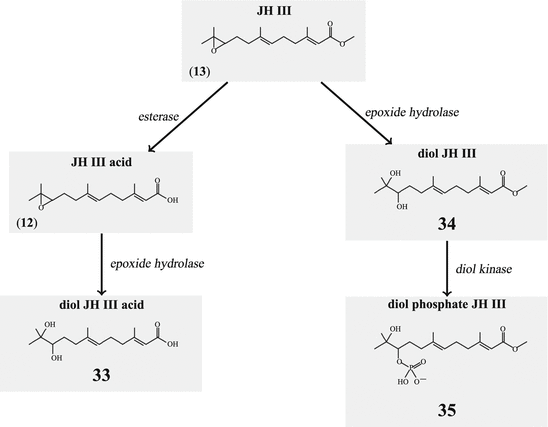
Fig. 6.8
Degradation of juvenile hormone
In the hemolymph, JH is transported bound to JH binding protein (JHBP).
The juvenile hormone of crustaceans is methyl farnesoate (11 which is made in the mandibular organ under the control of neuropeptides.
6.3.3 Physiology
Insect juvenile hormones have different functions in the larval and the adult animal. In the larval life JH inhibit precocious molt to the adult imago. Up to 15 molts are required in some species before the imago might arise. In the intensively studied butterflies or moths, for example, metamorphosis happens after the fifth instar. It can be postponed by experimental application of JH. For the regulation of JH synthesis stimulating allatotropins, inhibiting allatostatins, and JH-degrading enzymes such as esterases or epoxide hydrolases play their roles (Fig. 6.8).
The interactions of JH and ecdysons are discussed later.
Beyond their role in molting and pupation, JH exhibits several additional functions in the adult animal. In order to arrive at the final molt and at eclosion, the JH level in the hemolymph must be reduced to zero. After eclosion, however, the corpora allata again start JH synthesis and release. Within one day after eclosion JH levels in the hemolymph are again measurable. This JH simulates vitellogenin transcription, which protein is directly involved in female reproductive cycles. JH acts on the fat body. Furthermore, JH facilitates vitellogenin incorporation into competent follicles (Hartfelder 2000).
Crickets (Gryllus rubens ) exhibit two polymorphic types: long-winged, flying (LWF) and short-winged nonflying (SWN) crickets; these differ mainly in JH esterase expression (see Fig. 6.8). Thus it could initially be explained how small differences in JH levels in genetically identical crickets lead to two polymorphic phenotypes. In a particular phase JH titers in SWN crickets were lower than in LWF ones. A time-dependent, diurnal JH concentration in the hemolymph of LWF has been observed with fifteenfold to twentyfold JH increase in the light period compared to dark period whereas in SWN animals no difference due to a dark/light cycle could be found. The picture gets more complex by the finding that prolonged elevated JH levels cause the degradation of flight muscles. It is obvious that there is a narrow time window where LWF have to control their JH concentrations without losing their flight capacity (Zhao and Zera (2004).
In social insects the state of an individual within the colony depends on its hemolymph JH levels. In honeybees (A. mellifera) the different feeding of larvae to become queens and those to become workers induces enhanced JH levels in the queen larvae. These elevated JH levels no longer serve sex organ shaping. The queen suppresses sexual and egg-laying activities in worker bees by release of a pheromone: (E)-9-oxo-2-decenoic acid (Hartfelder 2000). In adult bees, flying activity is stimulated by JH: early in life in queens and drones, later in life for workers to get ready to collect nectar and pollen.
Whether a worker is active in the colony or foraging is determined by the corpora allata and thus most probably JH regulated. With increased corpora allata activity the nutrition forming hypopharynx undergoes a change of function, vitellogenin expression is reduced, and the cerebral mushroom bodies required for sensitive olfaction and orientation are enlarged. Such changes of JH function have only been observed in colony-forming honeybees; in solitary bumblebees, however, or in social wasps JH retains the original gonadotropic functions.
The juvenile hormone receptor has long been evasive. Very recently, the protein methoprene-tolerant has been found to mediate JH actions. Methoprene-tolerant is a bHLH protein that acts as a transcription factor. If methoprene-tolerant is indeed the JH receptor, then this fact would constitute a new type of mechanism of signal transduction: a bHLH transcription factor as receptor inhibiting ecdysone synthesis. Such a novel mechanism would also explain the long search for the receptor (Jindra et al. 2012). The other aspect of this regulation might be the fact that it antagonizes ecdysone-mediated actions and thus is a negative regulator of ecdysone-induced signal cascades.
6.4 Steroids
Steroids are derivatives of hypothetical sterane (36; aka gonane in the English literature; Fig. 6.9) a polycyclic molecule with four rings and 17 carbon atoms.
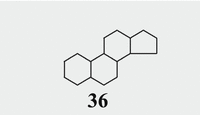
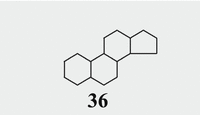
Fig. 6.9
Sterane/gonane backbone
Steroid hormones can be distinguished by the substituents of the 4-ring structure or by modifications of the ring itself: double bonds or one aromatic ring instead of an aliphatic one. Cholesterol is formed from squalene (14) which itself is built from mevalonic acid (1) via geranyl pyrophosphate (6) from farnesyl pyrophosphate (7) cyclized into lanosterol (16) (Fig. 6.4). Methyl groups in positions 4 and 14 are removed by cytochrome P450 (CYP) monooxygenases described below. The last intermediate is 7-dehydrocholesterol (18; see Fig. 6.26), which can be modified to vitamin D3 .
Vertebrate steroid hormones belong to five classes: gestagen s such as progesterone with 21 carbon atoms, glucocorticoid s such as cortisol and mineralocorticoid s such as aldosterone with 21 carbon atoms, androgen s such as testosterone with 19 carbon atoms, and estrogens such as estradiol with 18 carbon atoms. All these molecules are derived from common precursors: pregnenolone and progesterone the latter hormone and precursor for other steroids. The first characteristic and common precursor is prenenolone (37) which is derived from the membrane lipid cholesterol (19).
Numbers in Fig. 6.10 label the carbon atoms of cholesterol . This labeling is identical in all steroids: 17 carbon atoms in four rings, two methyl groups in positions 18 and 19, and the other carbon atoms in the side-chain.
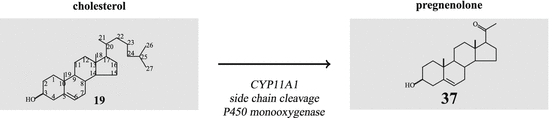
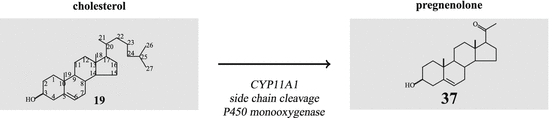
Fig. 6.10
Cholesterol and pregnenolone
Cholesterol is an important food ingredient for all those searching for healthy nutrition. Elevated cholesterol levels in blood indicate alimentation with a too-high fat content. Without cholesterol the entire steroid hormone anabolism would break down, with fatal consequences for metabolic homeostasis and reproduction. In addition cholesterol is indispensable for cell membrane stability.
It has been a long hunt to determine what the characteristic features of steroidogenic cells are. Not long ago, the activator protein StAR (steroid acute regulator) was identified as the first prerequisite. StAR itself is very labile: its translation in testis , adrenal , or ovary , as well as in brain is rate limiting for steroid production. StAR transports cholesterol from the cell membrane to the mitochondria, the only compartment where CYP11A1 cleaves the cholesterol side-chain giving rise to pregnenolone.
CYP11A1 is not the sole enzyme of steroidogenesis with such a determined place of action; we have to keep in mind that almost all steroidogenic enzymes act at selected places within the cell. The steroid intermediates thus have to migrate/be transported from one enzyme to the next, and the enzymes retain their positions .
Among the steroidogenic enzymes there are monooxygenase complexed with cytochrome P450 (Fig. 6.11). Such complexes in the liver are involved in detoxification of dangerous compounds. These different monooxygenase are counted CYP1 to CYP21 and further. For steroidogenesis, CYP11A1 , CYP11B1 , CYP11B2 , CYP17 , CYP19 , and CYP21 are relevant, together with CYP51 during cholesterol biosynthesis. Additional steroidogenic enzymes are 3
– and 17
-hydroxysteroid dehydrogenases (HSD) with several types of each. The conversion of testosterone in the more active androgen dihydrotestosterone is performed by the 5
-reductase.
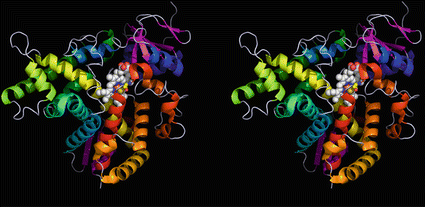



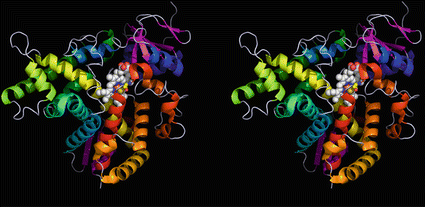
Fig. 6.11
Three-dimensional cytochrome P450 model: the lanosterol demethylase (CYP51). The protein consists of multiple helices (from blue to red), two beta sheet areas (pink), and the heme structure (white and red spheres) complexing a central iron ion (brown). Cysteine residue 394 is shown as spheres, its yellow sulfur atom binding to the iron atom. There are no disulfide bonds stabilizing the structure. CYP51 is widespread in all living organisms; the structure shown is from Mycobacterium tuberculosis (Source: Podust et al. (2001); Protein Data Bank entries 1EA1 and PyMOL)
To understand steroidogenesis it is not sufficient to know the steroid hormone structures: the knowledge of the steroidogenic enzymes, their time-controlled expression, and their compartmental organization are equally important.
6.5 Steroidogenic Enzymes
6.5.1 CYP11A1 , Cholesterol Monooxygenase
Fact sheet 6.2: CYP11A1: Cholesterol monooxygenase, 20,22-desmolase
Gene:
Chromosome 15, locus 15:q23, ten exons
Cell type:
All steroidogenic cells in adrenal, gonads and brain
Topology:
Located in the inner mitochondrial membrane
Function:
First steroidogenic enzyme, cleaves cholesterol to pregnenolone
This enzyme (aka P450 scc for side chain cleavage or 20,22-lyase) cleaves the cholesterol (19) side-chain between carbon atoms 20 and 22 (Fig. 6.10). The product of this reaction is pregnenolone (37). The earlier name cholesterol monooxygenase indicates that CYP11A1 acts as do all other CYP enzymes by oxidation. The enzymatic complex is assembled by the cytochrome P450 itself, the flavoprotein adrenodoxin reductase , the sulfur–iron-containing adrenodoxin and NADPH as cofactor. The CYP11A1 complex transfers stepwise oxygen molecules onto cholesterol carbon atoms 20 and 22 and takes up the hydrogen atoms, which are stored as water. The necessary electrons for the oxidation stem from NADPH which transfers electrons to the adrenodoxin reductase and in turn reduces adrenodoxin , and then transfers electrons to the cytochrome. From the first oxygen molecule (O2) one oxygen atom is bound to cholesterol as epoxide, the other atom becomes water. With the second O2 the epoxide is converted into the diol (plus a water molecule). The third O2 molecule cleaves the C–C bond and pregnenolone and isocaproic aldehyde are generated, the aldehyde being toxic is removed by the enzyme aldose-ketoreductase 1 B7 (AKR1B7). In very similar ways all CYP enzymes are active. These oxidations are irreversible.
CYP11A1 is an enzyme of the inner mitochondria l membrane (Farkash et al. 1986) as the adrenodoxin reductase . First, StAR has to transfer cholesterol from the cell membrane to the mitochondrium before side-chain cleavage and further steroidogenic conversions can occur.
In GenBank there are sequences from fish, amphibians, reptiles, birds, and mammals. To our surprise two sequences from Trichoplax (an early metazoan precursor) were listed as Cyp11a1. As they are as much related to Cyp24 than to Cyp11a1, the vitamin D3 hydroxylase (Fig. 6.12) and only have an overall identity of 32 % we do not (yet) consider the putative protein as an active Cyp11a1.
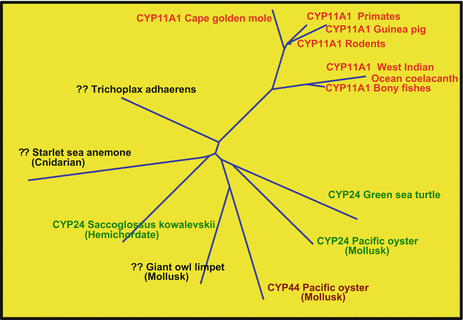
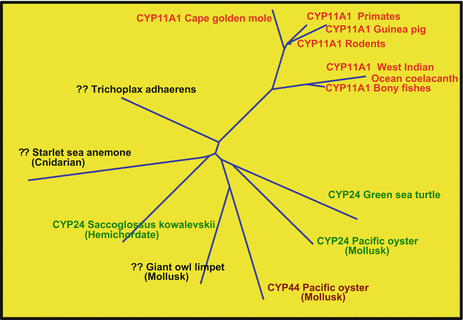
Fig. 6.12
A Cyp11a1 from Trichoplax? The Trichoplax protein TRIADDRAFT_56947 is most probably not Cyp11a1. It is as much related to Cyp11a1 as to different Cyp24.?? denotes a sequence not assigned to any Cyp
6.5.2 3β-HSD
Fact sheet 6.3: 3
-HSD type 1 (HSD3B1) and type 2 (HSD3B2)

Structure:
Not yet crystallized.
Gene:
Two genes on chromosome 1, locus 1p12, HSD3B1 four exons, HSD3B2 five exons.
Topology:
In the (smooth) endoplasmic reticulum.
Function:
This enzyme converts the 3-hydroxy group of several steroids with a 5,6 double bond into the 3-keto group and moves the double bond to the 4,5 position.
3
-Hydroxy-steroid dehydrogenase-
-isomerase converts pregnenolone (37) to progesterone (38) (Fig. 6.13). During this conversion the hydroxyl group on C-3 is oxidized to a keto group and the double bond between C-5 and C-6 is moved to positions 4 and 5.
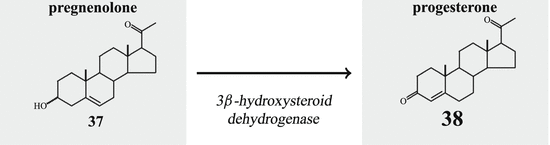


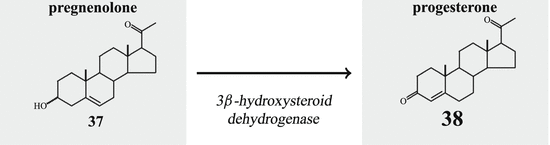
Fig. 6.13
Progesterone formation by 3β-HSD
Both human 3
-HSD isoenzymes are coded for on chromosome 1 and prefer NAD+ as cofactor. The type 2 enzyme is preferentially expressed in the adrenal and gonad s, whereas in placenta and other tissues type 1 is found.

The enzyme is not specific for the conversion of pregnenolone to progesterone. In a similar way, 17-hydroxypregnenolone (40) or dehydroepiandrosterone (DHEA (40) are concerted 17-hydroxy progesterone (41) or androstenedione (43) Fig. 6.14).
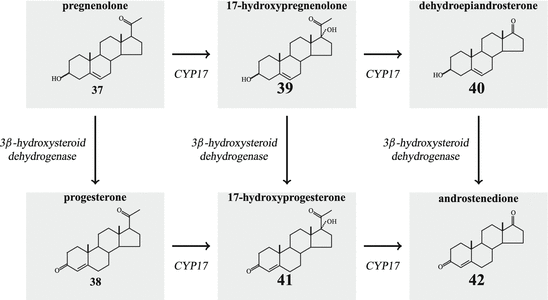
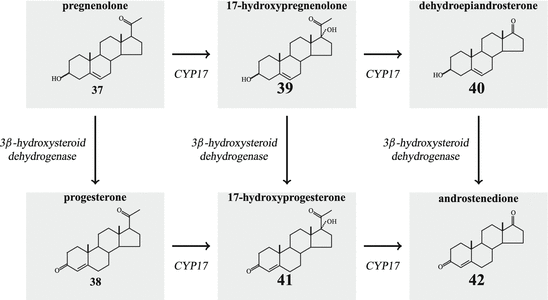
Fig. 6.14
Androgen formation by CYP17 and 3
-HSD

3
-HSD is an enzyme of the smooth endoplasmic reticulum.

6.5.3 CYP17 : Two Activities in One Enzyme
Fact sheet 6.4: CYP17
Structure:
3SWZ
Gene:
Chromosome 10; locus q24.32
Topology:
In the ER
Function:
Two enzymatic functions: 17-
-hydroxylase and 17,20-lyase

In the synthesis steps to cortisol and androgens/estrogens pregnenolone and progesterone are oxidized by CYP17. As the name suggests, the target of this enzyme is the C-atom in position 17 of the sterane/gonane backbone (see Fig. 6.10).
The human CYP17 gene is located on chromosome 10. The enzyme is, like HSD3B, active in the sER . For the conversion of pregnenolone by CYP17 the steroid has to leave the mitochondrion , where it has been generated, and to migrate through the cytosol to the ER.
CYP17 is like CYP11A1 described above, a monooxygenase, and uses electrons and oxygen to oxidize the carbon atom in position 17. The P450 oxidoreductase provides the electrons.
When CYP17 is phosphorylated by a cAMP-dependent protein kinase on serine and threonine amino acids, and
When the cytochrom B5 is present, and there is a threefold excess of P450 oxidoreductase above CYP17, then
CYP17 acquires a second function and becomes a 17,20-lyase that cleaves the bond between carbon atoms 17 and 20 thus removing the side-chain from the polycyclic ring system. Thus androgenic steroids with 19 carbon atoms are generated.
In contrast to other vertebrate-specific CYP enzymes, there are CYP17 in almost any deuterostomes and even in bacteria, making it one of the primordial cytochrom P450 monoxygenases such as CYP51.
6.5.4 17β-HSD
Fact sheet 6.5: 17
-HSD

Structure:
HSD17B type 1, 4, 5, 8, 10, 11, 14 have been crystallized (see Moeller and Adamski 2009).
Phylogeny:
Type 1: vertebrate; type 2: vertebrate, yeast; type 3: vertebrate; type 4: vertebrate, invertebrate, yeast, plants; type 5: vertebrate, invertebrate, plants; type 6: vertebrate, invertebrate; type 7: vertebrate, yeast; type 8: vertebrate, invertebrate, yeast, plants; type 9: mammalia; type 10: vertebrate, invertebrate, yeast; type 11: mammalia, birds; type 12: vertebrate, invertebrate, yeast, plants, plasmodium; type 13: vertebrate; type 14: vertebrate, invertebrate, plants.
Gene:
See Table 6.1
Table 6.1
Human 17
-hydroxysteroid dehydrogenases (Source: Moeller and Adamski 2006; Peltoketo et al. 1999a; Lukacik et al. 2007, PubMed/OMIM, http://www.ensembl.org/Homosapiens

HSD type | Gene name | Other names | Comments | Genomic location | GenBank no. | Subcellular localization | Tissue distribution | Oxidizing/activating? |
---|---|---|---|---|---|---|---|---|
1 | HSD17B1 | E17KSR, EDH17B1, EDHB17, EDH17B2 | 17 ![]() | 17q21.2 (plus one pseudogene) | X13440 | Cytosolic | Ovary, placenta, breast | Activating |
2 | HSD17B2 | E2DH, HSD17 | 17 ![]() ![]() | 16q23.3 | L11708 | Microsomal | Placenta, liver, GI tract, kidney, uterus, breast, prostate | Oxidizing |
3 | HSD17B3 | 17 ![]() | 9q22.32 | U05659 | Microsomal | Testis | Activating | |
4 | HSD17B4 | MFP-2, MFE-2, DBP | 3-Ketoacyl-DH, 17 ![]() | 5q23.1 | X87176 | Peroxisomal | Widely distributed | Oxidizing |
5 | AKR1C3 | HSD17B5 | 17 ![]() ![]() | 10p15.1 | D45850 | Cytosolic | Liver, kidney, testis, prostate, adrenal, bone | Activating |
6 | HSD17B6 | HSE, RODH | Retinal reductase/DH | 12q13.3 | NP_003716.2 | (Membrane bound) | (Prostate, liver) | Oxidizing |
7 | HSD17B7 | 3-Keto reductase, 17 ![]() | 1q23.3, 1q44 (pseudogene) 10p11.2 (short form) | NP_057455.1 | (Membrane bound/associated) | (Ovary, placenta, mammary gland) | Activating | |
8 | HSD17B8 | 17 ![]() probably fatty acid CoA-dehydrogenase | 6p21.32 | D82061 | Unknown | Liver, pancreas, kidney, skeletal muscle | Oxidizing | |
9 | RDH5 | HSD17B9 | Retinal reductase/DH | 0135437 | 12q13.2 | |||
10 | HSDH2 | HSD17B10, ERAB | 17 ![]() ![]() ![]() ![]() ![]() | Xp11.22 | Q99714 | Mitochondrium | Widely distributed | Oxidizing |
11 | DHRS8 | retSDR2, 17 ![]() ![]() | 17 ![]() | 4q22.1 | NP_057329.2 | Liver, lung, adrenal, steroidogenic cells | Oxidizing | |
12 | HSD17B12 | KAR | Ketoacyl-CoA reductase | 11p11.2 | NP_057226.1 | Liver, kidney, heart, skeletal muscle, pancreas, adrenal, pituitary, testis, placenta | Activating | |
13 | HSD17B13 | SCDR9, DHRS8 | Activity not demonstrated | 4q22.1 | NP_835236.2 | Cytoplasmic | Liver | Oxidizing |
14 | DHRS10 | HSD17B14, retSDR3 | 17 ![]() | 19q13.33 | NP_057330.2 | Cytoplasmic | Brain, liver, placenta | Oxidizing |
Function
17
-HSD catalyze the reduction of the 17-keto group to a 17
-hydroxy group (this is a steroid activating activity, for example, in the case of androstenedione to testosterone or estrone to estradiol conversion) or they catalyze the oxidation of the 17
-hydroxy function to a keto group, which is often the first stage of steroid inactivation. Many of the 14 enzymes have additional catalytic activities.



17
-Hydroxysteroid dehydrogenases belong with the exception of type 5 to the small dehydrogenase/reductase supergene family (SDR). Type 5 is a member of the aldoketo reductase gene family (AKR). Thus far 14 human enzymes with 17
-HSD activity have been described. Most of these enzymes possess other catalytic activities apart from 17
-dehydrogenase activity. The SDR family of enzymes is of ancient origin and some of the 14 HSD17B genes have homologues in protozoa, plants, and fungi. There are, however, some genes that are not older than the beginning of vertebrate evolution. The SDR enzymes are characterized by the so-called Kallman fold consisting of a bundle of seven parallel sheets, surrounding helices, and binding sites for the NAD/NADP cofactor and steroids.



The intracellular location of these enzymes is diverse. Some of them are cytosolic, some found in microsomes and other vesicles, and others are membrane-bound. It is difficult to see a general rule: whereas 17
-HSD type 5 (HSD17B5 ) in the adrenal cortex converts androstenedione (42) into testosterone (44) in the cytosol, the same conversion in testis is performed by 17
-HSD type 3 in the endoplasmic reticulum.


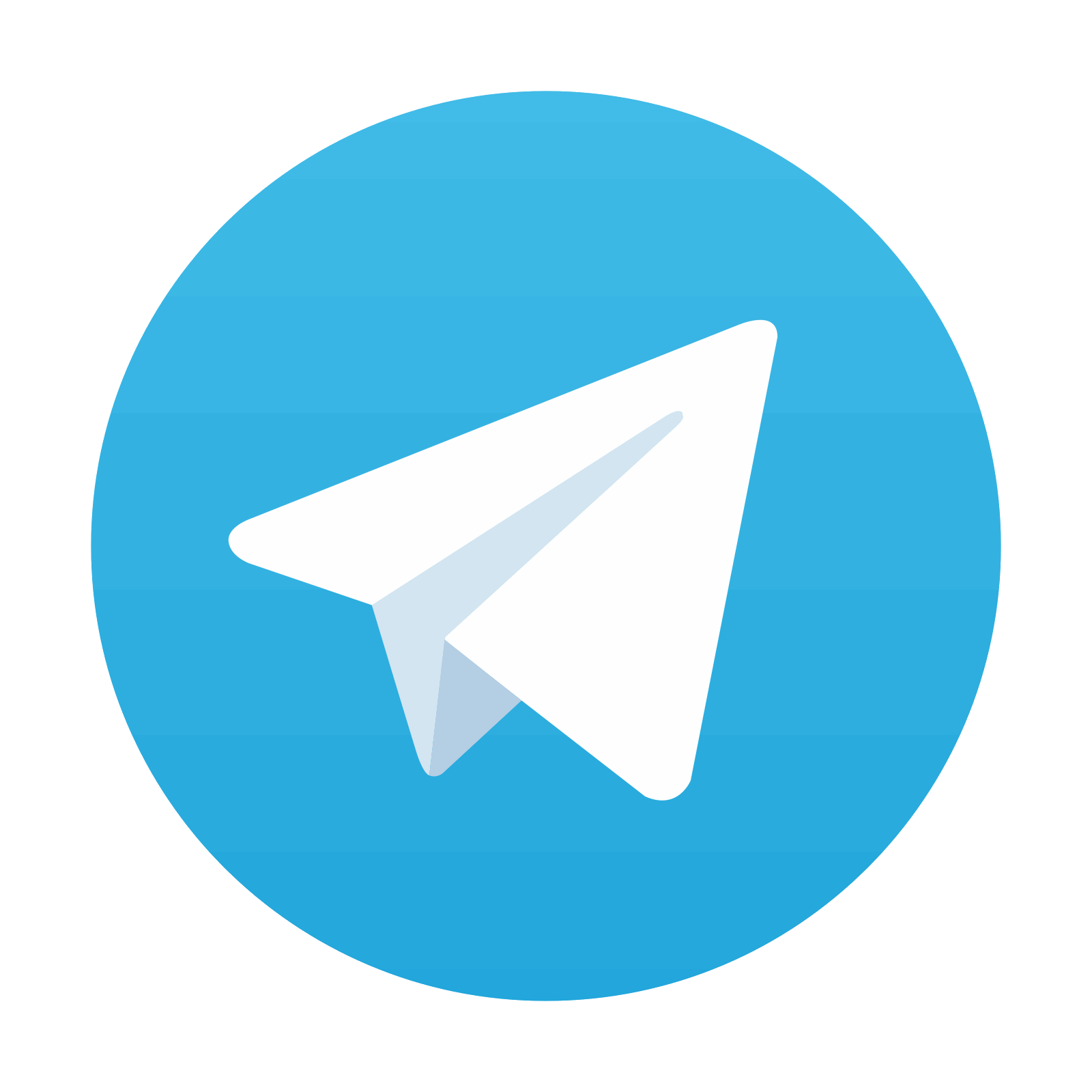
Stay updated, free articles. Join our Telegram channel

Full access? Get Clinical Tree
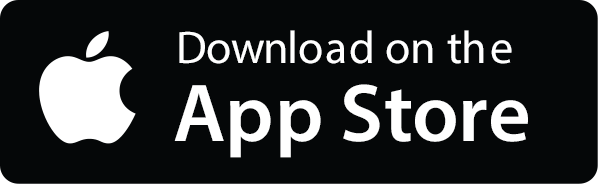
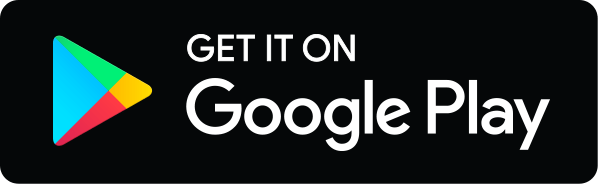