Fig. 12.1
(a) The target volume definition for glioblastomas according to the CCG-943 study and most commonly used in US centers. The red line depicts the extent of the primary postsurgical tumor bed and associated edema (gross tumor volume, GTV) on the appropriate sequence of a dedicated volumetric planning MRI scan. This target volume is then increased by an institutional three-dimensional margin of 2–2.5 cm (green line) limiting/editing the volume by any barriers of natural spread (clinical target volume, CTV). Finally a margin of 0.3–0.5 cm (pink line) is added to form the final planning target volume (PTV) which takes into account spatial variations of the target volume due to setup uncertainties during a course of daily radical radiotherapy. This PTV receives usually a total dose of 45–46 Gy. (b) Using a shrinking field technique (according to the CCG-943 guidelines) following 45–46 Gy patients receive a boost to the primary postoperative tumor bed and/or any areas of visible macroscopic contrast enhancing tumor (GTV, red line) outlined on a fused post-contrast T1-weighted volumetric sequence. This target volume is then increased by an institutional three-dimensional margin of 2–2.5 cm (green line) limiting/editing the volume by any barriers of natural spread (clinical target volume, CTV). Finally a margin of 0.3–0.5 cm (pink line) is added to form the final PTV. The PTV receives a further dose increase to the final prescription dose of 54–60 Gy depending on local institutional protocols. In contrast to many US sites this GTV/CTV/PTV is used in most European centers as the primary target volume using a single phase approach to the prescription dose of 54–60 Gy. At our own institution we have been using for more than two decades—compared to the published literature—smaller margins without detriment to clinical outcome. Our institutional GTV to CTV margin for high-grade gliomas is 1.7 cm and the CTV to PTV margin 0.3 cm and are depicted in (a)–(c). (c) This figure depicts the target volumes as defined in (b) transferred to the fused MRI planning sequence visualizing the tumor bed, postoperative changes and remaining peri-tumoral edema. It is highlighting that a target volume definition as used in many European centers does cover most of the remaining edema within the defined CTV/PTV albeit not completely. It is unlikely that any future clinical trials will prospectively assess the potential differences between these two different radiotherapeutic approaches either with respect to local control or toxicity. Thus the choice of the target volume definition used for glioblastomas will remain at the discretion of local services
12.7.4.1 Sequencing of Radiotherapy and Chemotherapy
The use of RT for children under 3 years requires careful evaluation on an individual basis, and is generally avoided in this age group of patients. The therapeutic ratio of brain RT for HGG is considered to be unfavorable for patients less than 3 years due to unacceptable neurocognitive toxicity, possibly as a result of incomplete myelination of brain white matter (Duffner et al. 1985; Ellenberg et al. 1987; Packer et al. 1989; Jannoun and Bloom 1990; Glauser and Packer 1991; Mulhern et al. 1992). Patients with HGG aged less than 3 years may be treated with surgery followed by prolonged chemotherapy, with the aim of deferring brain RT until the patient is older, and thereby reducing the risk of RT-induced morbidity (Duffner et al. 1993).
Limited data on effect of delaying RT after surgery for HGG are available from three studies. The Pediatric Oncology Group’s “Baby POG” study included 18 patients with HGG aged less than 3 years. Nine of these patients had an unclassified malignant glioma, six had GBM, and three had AA. Children less than 2 years received 24 months of chemotherapy (vincristine, cyclophosphamide alternating with cisplatin, etoposide), and those children between the ages of 2 and 3 years received chemotherapy for 12 months. Following chemotherapy, all children were recommended to receive brain RT. In addition, if any child developed progressive disease during chemotherapy, repeat surgery followed by RT was recommended. The 5-year PFS was 43%± and the 3-year and 5-year OS were both 50%±. The families of four children refused radiation after completion of 24 months of chemotherapy, and each of these four patients was alive and well at 43+ to 84+ months after diagnosis (Duffner et al. 1996). The CCG-945 study included 39 children younger than 2 years with HGG: 9 with GBM, and 20 with AA. Children received combination chemotherapy (the 8-in-1 regimen) for a maximum of ten cycles, amounting to up to 15 months of chemotherapy. The CCG-945 study protocol specified involved-field brain RT for all children following completion of chemotherapy, but only four patients received RT, and in two of these RT was given at the time of relapse. The 3-year PFS estimate for all patients was 31% ± 8%, and the OS at 3 years was 51% ± 8%. The 3-year PFS estimate for patients with AA and GBM was 44% ± 11% and 0, respectively (Geyer et al. 1995) [Geyer et al., Cancer 1995]. A chemotherapy only approach delivering 16 months of combination chemotherapy (the so-called BBSFOP regimen) was investigated in a multi-institutional French study that included 21 patients less than 5 years treated with postoperative chemotherapy following maximal surgical resection, with RT delayed until disease progression or recurrence. Consistent with the “Baby POG” results, this approach achieved a 5-year PFS of 35% ± and a 5-year OS of 59% ± (Dufour et al. 2006). Taken together, the results of these three studies suggest that HGG in younger patients has different biology and more favorable clinical behavior to HGG in older children and adults.
There are very few data on the use of chemotherapy before RT in children older than 3 years with HGG and the utility of this approach in this patient group is unclear. In a more contemporaneaous study, patients aged between 3 and 17 years with HGG were randomized between chemotherapy before RT (neoadjuvant) and chemotherapy during and after RT (concomitant and maintenance) (Wolff et al. 2002) Neoadjuvant chemotherapy consisted of alternating cycles of ifosfamide and etoposide, methotrexate, and cisplatin and cytarabine; RT started at week 17. Maintenance chemotherapy consisted of weekly vincristine during RT, and eight cycles of vincristine, lomustine, and cisplatin following RT. Although analysis of the subset of patients with gross total resection showed significantly improved survival for patients assigned to neoadjuvant chemotherapy compared to those who received concomitant and maintenance chemotherapy, more than one-third of patients with incompletely resected disease who received neoadjuvant chemotherapy experienced early tumor progression. Therefore, there is a concern that initial treatment with ineffective chemotherapy regimens and the delay of RT may worsen the outcome of children with macroscopic residual disease. Currently, involved-field RT is indicated for all children with HGG older than 3 years.
12.7.4.2 Radiotherapy Dose
The radiation tolerance of the normal brain parenchyma and its vascular and supporting structures are dose limiting for external beam brain RT. The risks of acute and long-term sequelae increase with increasing brain RT doses. Aside from the neurocognitive decline associated with brain RT, other well-recognized late effects include endocrinopathy, RT-induced tumors (benign and malignant), and vasculopathy. The risk and severity of these effects depend on patient age, the total volume of the planning target volume (PTV), and the proximity of PTV to critical organs at risk. The current standard RT dose for patients with HGG is 54–60 Gy delivered in daily fractions of 1.8–2.0 Gy over a period of 6 weeks. Since the overwhelming number of failures occur within the high-dose region of the RT volume, RT dose escalation has been investigated in several trials, but without evidence of benefit for doses greater than 60 Gy (Fulton et al. 1992; Packer et al. 1993). However, RT doses above 60 Gy are associated with significant long-term adverse normal tissue toxicity in long-term survivors (Werner-Wasik et al. 1996; Lawrence et al. 2010). Combined photon-proton therapy explored in a phase II trial at Massachusetts General Hospital—90CGE was delivered using accelerated fractionation, but there was no evidence of improved survival with 3-year OS of 18% (Fitzek et al. 1999). Alternative radiotherapy techniques such as hyper- and hypo-fractionation have not consistently proven to be statistically beneficial in children with HGG and should not be utilized outside of a clinical trial setting (Fallai and Olmi 1997). The failure of intensified RT to improve outcomes for HGG suggests that improved brain-directed therapies are needed.
12.8 Target Delineation
12.8.1 Radiotherapy Tumor Target Volumes
Involved-field RT for HGG delivers a therapeutic radiation dose to not only the postoperative tumor cavity and any residual tumor, that is the gross tumor volume (GTV), but must also include an additional circumference of brain tissue considered to be at risk of microscopic disease extension, which forms the clinical target volume (CTV). The GTV to CTV margin also allows for any uncertainties in tumor definition to be accounted for. A further circumferential margin is added to the CTV to allow for variations in daily treatment setup, to create the planning target volume (PTV). The ICRU 50 report provides formal definitions of the GTV, CTV, and PTV concepts and these are followed in RT planning for HGG. Specific normal tissue structures may be defined and identified as organs at risk (OARs), and each OAR may be given a surrounding safety margin to create a planning at risk volume (PRV) as defined by ICRU 50. The primary tumor should be treated, using a suitable technique that allows for the least amount of normal brain tissue and organs to be at risk from exposure to high-dose irradiation. Suitable techniques include 3D conformal RT, fixed field IMRT, and dynamic arc IMRT. 6MV photons are typically used to deliver RT. No therapeutic advantage from the use of particle therapy relative to megavoltage photon therapy has been demonstrated for treatment of pediatric HGG, with reported results being equivalent (McAllister et al. 1997). Dose variations across the PTV should be within +7% and −5% of the prescription dose according to ICRU 50 and 62 recommendations.
The GTV is defined by co-registering a volumetric (3D) MRI scan with the RT planning CT scan within the RT treatment planning system (TPS). The MRI needs to be obtained as close to time of RT planning as possible. Three-dimensional imaging data (CT ± MRI) are acquired with the patient in the treatment position with a slice thickness of ≤2.5 mm. Three-dimensional treatment planning software is used to determine beam arrangements and dosimetry. Image data are used to delineate and reconstruct a GTV, CTV, PTV, and organs at risk (OAR) in three dimensions. Pre- and post-op MRI images should be co-registered to the planning CT and available within the TPS at the time of contouring.
Two somewhat different philosophies towards tumor target volume definition for HGG have developed in North America and Europe, as illustrated by outlining guidelines within study protocols from Children’s Oncology Group (COG) and the European Society of Paediatric Oncology (SIOP-Europe). American technical approaches, for example, frequently use a two-phased approach (shrinking field technique). GTV1 is defined as the volume encompassing the contrast enhancing tumor tissue and all high signal changes as seen on T2-weighted imaging. GTV2 is defined as the volume encompassing only any residual contrast enhancing tumor elements/tumor bed as seen on postoperative MRI. Marginal expansion for GTV1 is an anatomically confined 2 cm margin to form CTV1, and the marginal expansion for GTV2 is 1 cm to form CTV2. Bone and dura act as barriers to disease infiltration, and so CTV1 and CTV2 are manually edited following marginal expansion to reside within the skull vault and to avoid crossing dural planes unless there is definite radiological evidence of disease. The CTVs may also be manually modified to avoid crossing midline and to avoid critical structures including the optic apparatus, pituitary, and brainstem. The CTVs are expanded to form the PTVs by means of an isotropic expansion without regard to anatomy—the margin applied is dependent on the patient positioning and immobilization systems used and is therefore institutional dependent, but in practice will typically be between 0.3 and 0.5 cm. The PTV1 dose is 54 Gy, and an additional dose of 5.4 Gy is prescribed to PTV2.
The HGG tumor volume definitions in use in most European centers are most commonly using a single phase approach. GTV is defined as the enhancing tumor as seen on gadolinium contrast enhanced MR at the time of RT planning (postoperatively) together with the entire surgical cavity if patient has been able to undergo either a debulking procedure or a gross total resection. The GTV must take into account any anatomical shift or other tissue changes after surgery. The CTV includes an additional margin of 2.0–2.5 cm in directions of potential tumor spread, and is edited to avoid crossing anatomical boundaries to tumor dissemination as per the COG guidelines (Vern-Gross et al. 2014). The PTV is generated from the CTV by the addition of an isotropic margin according to department policy (usually 0.3–0.5 cm). The standardly prescribed PTV dose is ranging between 54 and 60 Gy in conventional fractionation over a period of 6 weeks although there remains controversy if a dose increase from 54 Gy to 60 Gy in this patient population is critical for outcome.
12.8.2 Radiotherapy Organs at Risk
The following organs at risk (OARs) are frequently defined on the treatment planning system (TPS): supratentorial brain (left and right hemispheres); hippocampi (left and right); cochlea (left and right); pituitary; optic globes (left and right); lenses (left and right); optic nerves (left and right); optic chiasm; brainstem and cervical spinal cord (foramen magnum to C2). The lenses of the eyes should be excluded from primary beams unless a primary intensity modulated arcing technique is employed.
Cochleae. Each cochlea is contoured on the RT planning CT, viewed using bone-windows, as a circular structure within the petrous portion of the temporal bone anterior to internal acoustic meatus (IAM). The contour should appear on at least two successive axial CT slices. The aim is for the cochlea dose to be no more than D50% < 35.00 Gy for hearing preservation (Bhandare et al. 2010).
Optic Globes. Each eye is separately contoured on the RT planning CT from the most superior to inferior aspect. Effort should be made to avoid direct treatment of the anterior chamber of the eye to protect the lenses. Dose to the entire eye should be kept to the minimum possible, without compromising PTV coverage. If the COG tumor target definitions are used, then in the event that the optic globe dose constraints cannot be met, as a result of treatment of PTV2, then it may be preferable to compromise PTV2 coverage. Dose to the optic globes should be constrained to D50% < 20.00 Gy and D10% < 54.00 Gy (Mayo et al. 2010).
Optic Nerves and Chiasm. The optic nerves and optic chiasm may be contoured on the RT planning CT, or on a co-registered T1-weighted MRI. The optic chiasm is most readily identified on coronal images prior to outlining on axial images on the TPS. The right and left optic nerves are contoured separately, starting from the posterior globes to their exit from the optic canals. The optic chiasm is contoured as a single structure consisting of pre- and post-chiasmal nerves and a central body. The pre-chiasmal nerves start at the optic canals and terminate at the anterior body of the chiasm; the post-chiasmal nerves start from the posterior body of the chiasm and run posteriorly to enter the thalami. The incidence of radiation-induced optic neuropathy (RION) is uncommon with a Dmax <55 Gy at fraction sizes of <2 Gy. The RION risk increases to 3–7% for doses in the region of 55–60 Gy with fraction sizes of 1.8–2.0 Gy (Mayo et al. 2010). SIOP-E protocols specify optic apparatus dose constraints of D50% < 56.00 Gy and D10% < 60.00 Gy. Efforts should be made to avoid direct treatment of the optic nerves and chiasm—without compromising target volume coverage during treatment of PTV1. In the event that the recommended constraints provided in this section would be exceeded as a result of treatment of PTV2, the treating radiation oncologist may use their discretion to reduce target volume coverage.
Hypothalamus/Pituitary. The hypothalamus and pituitary may be contoured as a single composite structure on the RT planning CT. Guidance on the definition of this volume is available from contouring atlases produced for clinical trials, such as COG ACNS0331 (http://www.qarc.org/cog/ACNS0331Atlas.pdf Michalski J). Retrospective data from a cohort of childhood cancer survivors treated with cranial radiotherapy for a variety of diagnoses found that compared with total RT doses <22 Gy, doses of 22 Gy to 29.9 Gy were associated with GH deficiency; doses ≥22 Gy were associated with LH/FSH deficiencies; and doses ≥30 Gy were associated with TSH deficiency and ACTH deficiency (Chemaitilly et al. 2015) The aim is to keep the hypothalamus and pituitary dose D50% < 20.00 Gy, but never at the expense of PTV coverage, which should always take priority.
Hippocampus. The hippocampal structures are best contoured on a T1-weighted MRI co-registered with the RT planning CT, according to the Radiation Therapy Oncology Group (RTOG) hippocampal outlining atlas (Gondi V et al.). Retrospective data from the Childhood Cancer Survivor Study (CCSS) demonstrate an increased risk for memory difficulties with RT doses above ≥30 Gy to the temporal lobe region (Armstrong et al. 2010). Effort should be made to minimize dose to the hippocampi but without compromise to PTV coverage, which always takes priority.
Brainstem. The brainstem is contoured directly on the RT planning CT. The volume includes the midbrain, pons, and medulla. The cranial boundary is inferior to the third ventricle and optic tracts. The caudal boundary of the brainstem is taken as the foramen magnum. For supratentorial HGG, the brainstem can usually be excluded from the CTV. The entire brainstem may be treated to 54 Gy using fraction sizes of ≤2 Gy with a low risk of irreversible neurological toxicity (Mayo et al. 2010; Merchant et al. 2010). Smaller volumes of the brainstem (1–10 cc) may be irradiated to a maximum dose of 59 Gy at dose fractions of ≤2 Gy, but the risk of neurological toxicity increases markedly at doses beyond 64 Gy (Mayo et al. 2010).
Spinal Cord. For supratentorial HGG, spinal cord dose constraints do not typically pose difficulties for RT planning. With conventional fraction sizes of ≤2 Gy, total doses of 50 Gy and 60 Gy to the full spinal cord cross section are associated with a risk of myelopathy of 0.2% and 6% respectively (Kirkpatrick et al. 2010). SIOP-E guidelines specify spinal cord dose constraints of D50% < 50.00 Gy and D10% < 55.80 Gy. The spinal cord (not the spinal canal) is contoured on the RT planning CT using suitable window settings. The spinal cord begins where the brainstem ends, at the inferior border of the foramen magnum.
12.9 Outcomes
12.9.1 Follow-Up Guidelines
CNS tumors are the leading cause of morbidity in survivors of childhood tumors/cancers. They require dedicated follow-up clinics with access to all aspects of the multidisciplinary team, including endocrinology and psychology. The CCLG guidelines are a good reference for the organization of a follow-up clinic.
12.9.2 EFS, DFS, OS
Historical data is somewhat clouded by the contamination of trials by low-grade gliomas, rather than true high-grade tumors. The ACNS0126 trial (with fewer than 10% of tumors reclassified as low grade) demonstrated a 3-year EFS of 13 ± 6% for those patients with an anaplastic astrocytoma (AA) and 7 ± 4% for GBM.
12.9.3 Toxicities
Depending upon the treatment paradigm used, toxicity patterns differ. In the acute period, common toxicities whilst on chemotherapy include myelosuppression (with the resulting risk of infection), nausea, hair loss, etc. For those on radiotherapy, hair loss, skin changes, headaches, and seizures are seen. Long-term toxicities are more problematic; however, given the poor long-term survival rates, they are not as pressing an issue as they are in low-grade glioma, for example. Medium- and long-term toxicities can be broadly grouped into those caused by chemotherapy and those caused by radiotherapy. Chemotherapy may lead to sensorineural hearing deficits, infertility, and peripheral neuropathy. Long-term toxicity data from radiotherapy pertains to historic techniques and, as such, may differ from those expected with modern techniques. Toxicities are related to the organs at risk and their respective radiotherapy doses. Endocrine function must be monitored. Longer term, there is a potential risk of second malignancies and stroke.
12.10 Future Directions
Despite advances in many other areas of oncology, improvements in outcomes for patients with pediatric high-grade glioma remain poor. Improvements in surgical resection techniques through the use of aides, such as cortical mapping and 5-ALA fluorescence, may improve macroscopic complete resection rates. Combination regimens of traditional cytotoxic chemotherapies have not provided the improvements expected.
In the era of personalized medicine, next generation sequencing and whole exome sequencing of tumor samples have led to the identification of potential molecular targets. These include mutations within the phosphatidylinositol-3-kinase pathway (PI3K), the sonic hedgehog (SHH) pathway, and the RAS/RAF/MEK pathway. Molecules targeting these mutations are already in clinical trials; we await results. The heterogeneity of mutational profiles will need to be incorporated even more into clinical trials. Already in the adult setting, it is recognized that molecular characteristics may be more significant than histological classification; undoubtedly, pediatric glioma is similar. The increasing incorporation of “basket” design studies will facilitate further investigation of targeted therapies.
Immune modulation is an area of intense interest across many oncological specialties; neuro-oncology is no exception. Studies in pediatric gliomas have focused on vaccines, deriving antigens from the tumor itself. These studies are ongoing and their results are awaited.
Another novel strategy under investigation in a new cooperative study is the combination of multi-agent chemotherapy with a randomization to maintenance valproic acid as a cell differentiating agent.
References
Alonso M, Hamelin R, Kim M et al (2001) Microsatellite instability occurs in distinct subtypes of pediatric but not adult central nervous system tumors. Cancer Res 61:2124–2128PubMed
Armstrong GT, Jain N, Liu W et al (2010) Region-specific radiotherapy and neuropsychological outcomes in adult survivors of childhood CNS malignancies. Neuro Oncol 12:1173–1186. doi:10.1093/neuonc/noq104 CrossrefPubMedPubMedCentral
Barrow J, Adamowicz-Brice M, Cartmill M et al (2011) Homozygous loss of ADAM3A revealed by genome-wide analysis of pediatric high-grade glioma and diffuse intrinsic pontine gliomas. Neuro Oncol 13:212–222. doi:10.1093/neuonc/noq158 CrossrefPubMed
Batra V, Sands SA, Holmes E et al (2014) Long-term survival of children less than six years of age enrolled on the CCG-945 phase III trial for newly-diagnosed high-grade glioma: a report from the Children’s Oncology Group. Pediatr Blood Cancer 61:151–157. doi:10.1002/pbc.24718 CrossrefPubMed
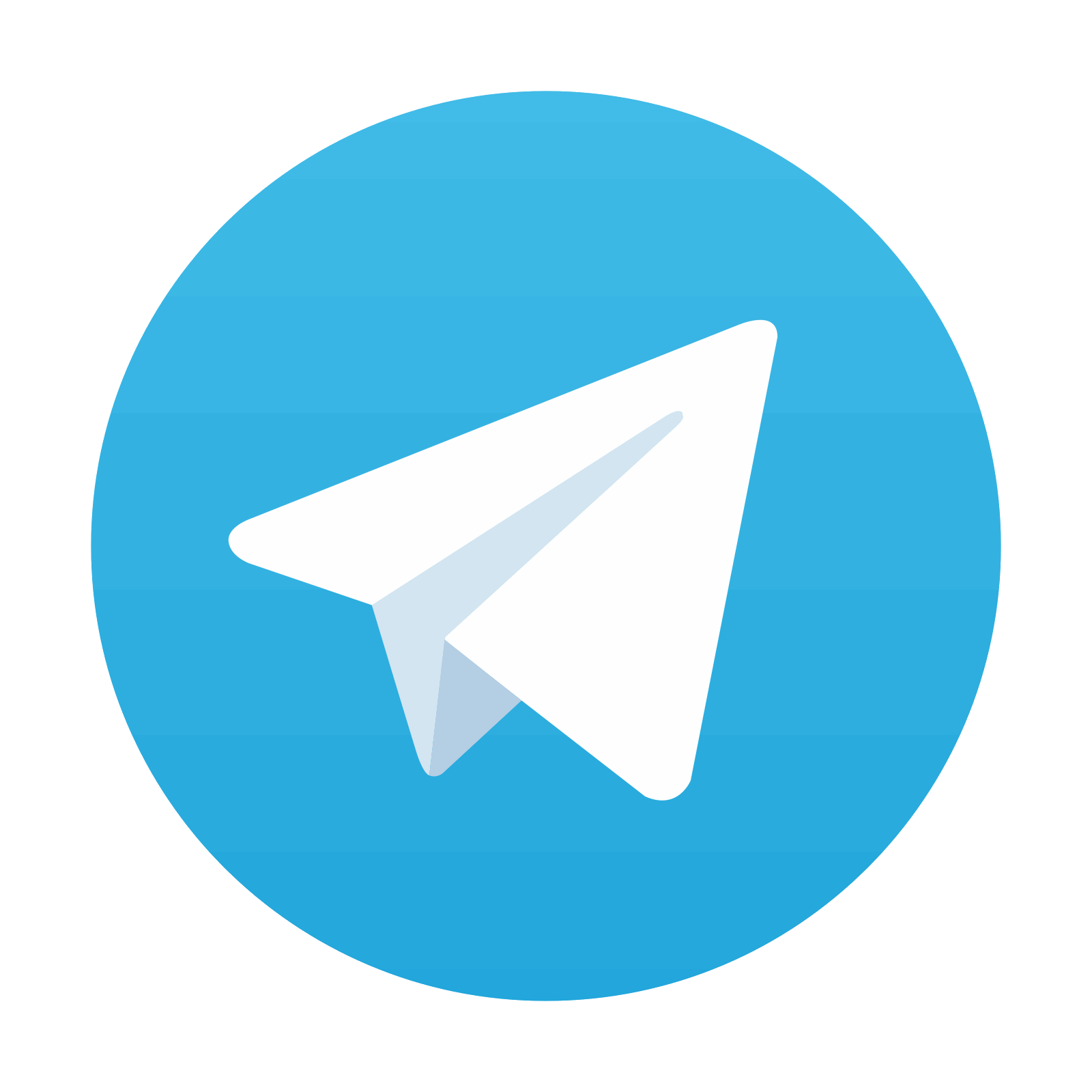
Stay updated, free articles. Join our Telegram channel

Full access? Get Clinical Tree
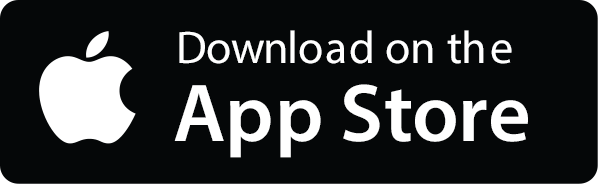
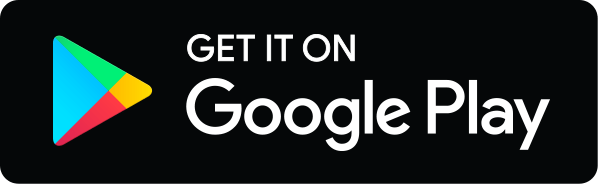