Many hematologic malignancies have an underlying heritable component. Although not as well characterized as the acquired genetic abnormalities that define important prognostic and therapeutic subgroups of myeloid and lymphoid neoplasms, investigations are beginning to unravel the role of germline genetic variation in the predisposition to hematologic malignancies. Information gained from the study of striking family pedigrees, epidemiologic data, and candidate genes are now being combined with unbiased genome-wide investigations to outline the network of genetic abnormalities that contribute to hematologic malignancy risk. This article reviews the current understanding of the heritability of hematologic malignancies in the genomics era.
It has long been recognized that there are several familial genetic syndromes, such as Lynch syndrome or hereditary breast and ovarian cancer syndrome, associated with a predisposition to solid tumors. Although not as well characterized, there also is an underlying heritable component to many hematologic malignancies. Striking family pedigrees have been described in which multiple individuals are affected by leukemia or lymphoma, and hematologic malignancies are among the tumors associated with cancer predisposing syndromes such as Li-Fraumeni and ataxia telangiectasia (reviewed in Refs. ). Epidemiologic data also provide evidence for a heritable component to even seemingly sporadic cases of leukemia and lymphoma. Indeed, first-degree relatives of patients with leukemia are at a 2.5- to 6-fold increased risk of leukemia, and first-degree relatives of patients with non-Hodgkin lymphoma (NHL) are at a 1.6- to 3.5-fold increased risk of NHL.
Although these observations clearly demonstrate that there is an inherited component of risk for several hematologic malignancies, these genetic susceptibilities remain largely unknown. There is an extensive body of research characterizing the multitude of acquired recurrent genetic abnormalities in sporadic leukemia and lymphoma, which have facilitated the classification of these diseases into prognostically and therapeutically important subgroups. Little is known, however, about the genetic abnormalities that predispose one toward their development and influence the subtype diagnosed in any given individual. Thus far, with few exceptions, attempts to identify the genetic factors underlying even highly familial hematologic malignancies have not been successful. Consequently, new strategies are necessary to unravel the heritability in these families.
This review describes what has been learned about the genetic contribution to hematologic malignancies through the study of both familial and sporadic disease. Furthermore, the authors highlight how the study of familial disease may inform the study of sporadic disease. Thus, the goal is to provide a comprehensive overview of our current understanding of the heritable component of hematologic malignancies in the genomics era. Although some of the genetic syndromes with which hematologic malignancies are already known to be associated are discussed in this review, a more comprehensive list is provided in Table 1 .
Heme Malignancy | Pathway Disrupted | Syndrome | Inheritance Pattern | Gene Abnormality | Chromosomal Location | Risk of Heme Malignancy | References |
---|---|---|---|---|---|---|---|
AML | Tumor suppressor | Familial leukemia with CEBPA mutation | AD | CEBPA | 19q13 | Near 100% | |
Familial platelet disorder | AD | RUNX1 | 21q22 | MDS/AML: 50% a | |||
Neurofibromatosis, type I | AD | NF1 | 17q11 | Myeloid: 500-fold JMML 224-fold | |||
DNA damage response | Li-Fraumeni | AD | TP53 CHK2 | 17p13.1 22q12.1 | 4.8% b | ||
Telomere maintenance | Dyskeratosis congenita | X-linked R | DKC1 | Xq28 | MDS: >2000-fold (10%) AML: 200-fold (4%) | ||
AD | TERC TERT TNF2 | 3q26 5p15 14q12 | |||||
AR | NHP2 NOP10 | 5q35 15q14 | |||||
Ribosome biogenesis | Shwachman-Diamond syndrome | AR | SBDS | 7q11 | 10%–25% | ||
Diamond-Blackfan anemia | AD | RPS19 RPS17 RPS24 RPL11 RPL35A | 19q13 15q 10q22-q23 1p36.1-p35 3q29-qter | 4% | |||
DNA repair | Fanconi anemia | AR | FANCA FANCC FANCD1 ( BRCA2 ) FANCD2 FANCE FANCF FANCG FANCI FANCJ FANCL FANCM FANCN | 16q24 9q22 13q12 3p26 6p22 11p15 9q13 15q26 17q22 2p16.1 14q21.2 16p12.2 | 650- to 868-fold (8%) | ||
X-linked R | FANCB | Xp22.2 | |||||
Bloom syndrome | AR | BLM ( RECQL3 ) | 15q26.1 | 13% | |||
Werner | AR | RECQL2 | 8p12 | Unknown | |||
Rothmund-Thomson | AR | RECQL4 | 8q24.3 | Unknown | |||
Nijmegen breakage syndrome | AR | NBS1 | 8q21 | 1%–2% | |||
Megakaryopoiesis | Congenital amegakaryocytic thrombocytopenia | AR | MPL | 1p34 | Unknown | ||
Chromosome number | Down syndrome | Sporadic | Trisomy 21 | Additional 21 | M7 AML 400-fold TMPD 10% | ||
Ras/MAPK pathway | Noonan syndrome | AD | PTPN11 | 12q24 | Unknown | ||
Other | Severe congenital neutropenia | AD | ELA2 (50%–60%) GFI1 CSF3R | 19p13.3 1p22 1p35-p34.3 | 10% | ||
AR | HAX1 c G6PC3 | 1q21.3 17q21.31 | |||||
X-linked R | WAS | Xp11.23-11.22 | |||||
ALL | Chromosome number | Down syndrome | Sporadic | Trisomy 21 | Additional 21 | ALL: 10-20-fold | |
Tumor suppressor | Neurofibromatosis, type I | AD | NF1 | 17q11 | ALL: 5.4-fold | ||
DNA damage response | Ataxia telangiectasia | AR | ATM | 11q22.3 | ALL, PLL, lymphoma (mostly T cell): 13% | ||
Li-Fraumeni | AD | TP53 CHK2 | 17p13.1 22q12.1 | 4.8% b | |||
DNA repair | Bloom syndrome | AR | BLM ( RECQL3 ) | 15q26.1 | 13% | ||
Nijmegen breakage syndrome | AR | NBS1 | 8q21 | 3%–4% | |||
Immune deficiency | Wiskott-Aldrich | X-linked R | WAS | Xp11.23 | 13% d | ||
Ras/MAPK pathway | Noonan syndrome | AD | PTPN11 | 12q24 | Unknown | ||
HL | Apoptosis defect | ALPS | AD | APT1 ( TNFRSF6 ) | 10q24.1 | 50-fold | |
DNA damage response | Li-Fraumeni | AD | TP53 CHK2 | 17p13.1 22q12.1 | 4.8% b | ||
DNA repair | Bloom syndrome | AR | BLM ( RECQL3 ) | 15q26.1 | 1% | ||
Immune deficiency | Immunodeficiency with hyper-IgM | X-linked R | CD40LG | Xq26 | Unknown | ||
NHL | Apoptosis defect | ALPS | AD | APT1 ( TNFRSF6 ) | 10q24.1 | 14-fold | |
Tumor suppressor | Neurofibromatosis, type I | AD | NF1 | 17q11 | NHL: 10-fold | ||
DNA damage response | Ataxia telangiectasia | AR | ATM | 11q22.3 | ALL, PLL, lymphoma (mostly T cell): 13% | ||
DNA repair | Bloom syndrome | AR | BLM ( RECQL3 ) | 15q26.1 | 13% | ||
Nijmegen breakage syndrome | AR | NBS1 | 8q21 | 29% | |||
Immune deficiency | Wiskott-Aldrich | X-linked R | WAS | Xp11.23 | 13% d | ||
Common variable immunodeficiency | AR and AD | TNFRSF13B and others | 17p11.2 | 9% | |||
Severe combined immunodeficiency | AR | ADA | 20q13.11 | 5% | |||
X-linked lymphoproliferative syndrome | X-linked | SAP ( SH2D1A ) | Xq25 | 20% | |||
Immunodeficiency with Hyper-IgM | X-linked R | CD40LG | Xq26 | Unknown | |||
CLL | DNA damage response | Li-Fraumeni | AD | TP53 CHK2 | 17p13.1 22q12.1 | 4.8% b |
a Denotes the median incidence of MDS/AML in FPD/AML.
b Denotes incidence of all hematologic malignancies in Li-Fraumeni.
c Kostman syndrome is another name for severe congenital neutropenia with HAX1 mutations.
d Denotes incidence of all lymphoreticular malignancies in Wiskott-Aldrich.
Chronic lymphocytic leukemia
As early as the 1920s, detailed reports were published describing familial clustering of chronic lymphocytic leukemia (CLL) cases and proposing a possible genetic basis to this disease. Now, more than 200 familial CLL pedigrees have been identified. The largest of these families comprises 222 individuals, of whom 10 have developed CLL, 1 has developed a T-cell NHL, and others have developed a variety of other cancers. In a second family comprising 144 individuals, 11 have developed CLL. The onset of CLL in these multiplex families is markedly younger than is observed in sporadic disease, with an average age at diagnosis of 56 years compared with 72 years for sporadic cases. Several large epidemiologic studies undertaken in cohorts mainly comprised of individuals of European descent have confirmed that there is a heritable component of risk for CLL, demonstrating a 3- to 8.5-fold increased risk of CLL among first-degree relatives of probands. In addition, indolent NHLs cosegregate with CLL in these families with lymphoplasmacytic lymphoma/Waldenstrom macroglobulinemia (4-fold risk) and hairy cell leukemia (3.3-fold risk) the most common. Initially, it was believed that the inheritance of CLL in these large families was consistent with an autosomal dominant mode of inheritance with incomplete penetrance, but in smaller families, consisting mostly of affected siblings, it was found to be more consistent with a recessive or other mode of inheritance. Using DNA from multiple individuals within large multigenerational pedigrees, positional cloning techniques such as familial linkage mapping provided a logical method by which to uncover candidate CLL susceptibility genes. Consequently, both single-family and combined multifamily linkage studies were undertaken.
Neither of the 2 single-family studies undertaken was successful in identifying a single locus that was associated with disease, although several regions did emerge as interesting candidates. In the first study of 8 affected and 14 unaffected individuals from a single family, a locus at chromosome 14q24 achieved a suggestive nonparametric linkage (NPL) score of 2.24, and 3 other loci at 2q37, 4q35, and 11p15, achieved less significant NPL scores (0.3–2.07). In the second study of 4 affected and 2 unaffected individuals in a smaller family, chromosome 9q achieved an NPL score of 0.96. Multifamily studies, adding both genetic heterogeneity and a greater number of individuals for analysis, also identified numerous loci. In the largest and most recent of these studies, more than 10,000 single nucleotide polymorphisms (SNPs) were genotyped in more than 400 affected individuals from 182 CLL pedigrees and 24 pedigrees showing cosegregation of CLL and B-cell lymphoproliferative disorders. This study yielded evidence suggestive of linkage to 2q21.2 (maximum NPL 3.02, P = .001), linking 68% of families under a common recessive model of inheritance.
Comparing all CLL linkage studies, however, no single locus was consistently identified from study to study. In addition, none of the most significant loci identified in these studies corresponded to loci commonly somatically mutated in sporadic CLL, such as 13q14 (up to 55% of sporadic cases), 11q (17%–20%), 12q (16%–20%), 6q (2%–7%), and 17p (3%–5%). Although pedigree data from family studies provide some evidence for Mendelian inheritance, no single locus has emerged to support a simple Mendelian inheritance pattern. Instead, the data suggest a model in which multiple common susceptibility alleles are co-inherited, each of which contributes only modestly to CLL susceptibility.
Identifying these risk variants is challenging. Multiple candidate gene studies, in which individual genes are investigated, based on their known involvement in biologically relevant pathways, such as B-cell differentiation, or based on their location within disease-associated regions identified in linkage or association studies, have been performed in an attempt to identify these risk variants. Overall, they have had limited success in identifying genetic variants accounting for a significant portion of CLL heritability. Some, however, have yielded insight into potentially disrupted pathways that merit further investigation.
One such candidate gene, initially identified through a familial linkage study, was DAPK1 . In this study, Raval and colleagues identified a common inherited haplotype on 9q in family members by linkage mapping. They selected DAPK1 , which encodes a serine/threonine kinase that promotes apoptosis, for further investigation because they had previously shown that DAPK1 expression was commonly decreased in sporadic CLL by promoter hypermethylation. Consequently, they hypothesized that this gene was also involved in familial CLL. Indeed, in their familial CLL cases, they demonstrated decreased expression of DAPK1 by both genetic and epigenetic mechanisms. First, they identified a rare suppressor-region SNP, which was associated with a 70% decrease in gene transcription relative to wild-type. Then, they demonstrated an even further attenuation of gene expression in affected family members by promoter hypermethylation-mediated gene silencing. These results are intriguing, as they not only identify a single gene potentially involved in both familial and sporadic cases but also highlight epigenetic modulation as one possible mechanism underlying the development of CLL. This adds to evidence from sporadic disease, in which aberrant methylation has been found in both promoters of individual genes and more globally at nonrandom sites within 4.8% of the CLL genome.
In 2 other candidate gene studies undertaken in sporadically occurring CLL, investigators genotyped SNPs located in candidate cancer-related genes and detected putative risk loci in genes that are members of the ATM-BRCA2-CHEK2 DNA damage response pathway, as well as in genes involved in apoptosis ( APAF1 and CASP8 ), immunoregulation ( NOS2A , IL16 , and CCR7 ), and cell cycle regulation ( CCNH ). More than 900 candidate gene studies have been published on CLL. Despite the considerable effort involved in these investigations and these few intriguing results, most findings from candidate gene studies await replication in subsequent analyses. Thus, candidate gene studies have been largely unsuccessful in identifying sources of inherited genetic variation responsible for a significant portion of CLL risk. This is not surprising as they are inherently limited to the investigation of genes and variants based on preexisting knowledge.
Genome-wide association studies (GWAS) are a recently developed method of undertaking an unbiased analysis of the entire genome to identify common susceptibility alleles associated with a complex disease. In GWAS, SNPs throughout the genome are genotyped in cases and controls. Although only a relatively small number of SNPs are actually genotyped using even the densest of currently available genotyping platforms, because of linkage disequilibrium (LD), these SNPs serve as tags to identify genomic regions containing disease-associated variation. These studies, however, require large numbers of ethnically similar cases and controls to attain sufficient power for the detection of this variation, as well as sophisticated statistical analyses to control for multiple testing and to assess the potentially confounding effects of unsuspected genetic or nongenetic factors. Furthermore, because the effect size of most disease-associated variants is frequently small, these studies are reliant on replication in an independent cohort for validation. Consequently, the role of GWAS has been largely limited to the study of sporadic CLL.
However, one GWAS undertaken in CLL was enriched for familial disease with 155 probands from multiplex families included among 517 CLL cases of European descent analyzed. More than 350,000 SNPs were genotyped in these cases and in 1500 controls. Six susceptibility loci were identified and replicated in 2 separate case-control cohorts. Of these, one, located at 6p25.3 was in a gene involved in B-cell development and differentiation ( IRF4 ), and another, located at 2q37.1, was near a gene regulating the immunologic response to viral infection ( SP140 ). In both cases, the risk-associated SNP was correlated with decreased mRNA expression in Epstein-Barr virus (EBV)-transformed lymphocytes. None of the other 4 loci, 2q13, 11q24.1, 15q23, and 19q13.32, were associated with specific genes.
Recently, the investigation of microRNAs in cancer has engendered considerable excitement. MicroRNAs are 18 to 22 nucleotide single-stranded noncoding RNAs formed from a double stranded RNA precursor. They play a prominent role in the regulation of gene expression via multiple epigenetic mechanisms (reviewed in Ref. ). One mechanism by which microRNAs attenuate gene expression is by binding messenger RNAs through sequence complementarity and targeting them for degradation. Because of its short length, a single microRNA often has homology to a variety of mRNAs, and is therefore able to control the expression of a network of genes by binding to multiple messenger RNAs. Altered expression of microRNAs through various mechanisms, including somatic mutation, has been implicated in the pathogenesis of multiple cancers, including CLL.
To identify microRNAs involved in CLL pathogenesis, Calin and colleagues examined the global microRNA expression profile of CLL cells from 94 patients and identified a signature containing 13 microRNAs, which differentiated patients with poor prognostic molecular markers (ZAP-70 expression and unmutated IgV H ) from those without these markers. In addition, a germline mutation was identified in 1 allele of the precursor to miR-16-1 in 2 patients, including 1 with other family members with CLL and breast cancer. In both patients, a somatic mutation in the other allele was observed. This germline mutation in pri-miR-16-1 was associated with decreased expression of both miR-16-1 and miR-15a , which function as tumor suppressors in CLL by regulating the expression of many genes including the CLL-associated genes as BCL-2 and WT1 . These 2 microRNAs are located at 13q14, the site most frequently deleted in sporadic disease, and were previously shown to be lost in most sporadic cases of CLL. This evidence of microRNA deregulation in both sporadic and familial CLL gives further weight to their likely role in CLL pathogenesis.
Although suggestive, the positive associations identified through these varied techniques require replication in additional cohorts for validation. In addition, given the multiple loci identified from candidate gene, linkage, genome-wide association, and microRNA studies, the challenge now is to prioritize for further investigation and functional verification those associations that truly contribute to CLL risk. Ideally, as consistent genetic abnormalities and predispositions are identified, studies integrating genomics, bioinformatics, and molecular biology can be used to generate a network of independent and interacting susceptibility variants that together define the pathways associated with CLL risk.
For now, however, clinicians are limited in their ability to counsel families with CLL about the genetic basis of their disease. They can estimate the risk in family members for CLL to be between 3 and 8.5 times that of the general population. Given that the lifetime risk of CLL development in the general population is low at 0.47% ( http://seer.cancer.gov/statfacts/html/clyl.html ), however, the overall risk for family members remains small. The same is true for risk of other indolent lymphomas in these families. Epidemiologic data suggest that the risk of solid tumors (in particular, breast, prostate, and gastrointestinal cancer) is also increased in relatives of patients with CLL and in individuals with CLL. Multiple retrospective and observational studies have identified an overall 1.2- to 3-fold increased risk of second malignancies in patients with CLL, particularly lung and skin cancer, although a variety of tumor types have been reported, so these patients should follow routine cancer screening guidelines and be aware of this increased risk (reviewed in Ref. ).
Within a single family, clinicians may be able to identify specific individuals more likely to develop CLL than others through detection of a monoclonal B-cell lymphocytosis (MBL). MBL is a clonal population of B cells at low levels not meeting the criteria for overt CLL, and can now be detected by sensitive techniques such as flow cytometry. MBL is considered a precursor condition to CLL. MBL is found in 3.5% to 5% of normal individuals in the general population with an approximately 1.1% per year risk of overt CLL development, but is found in up to 13.5% of healthy relatives of case with familial CLL, suggesting that these individuals may be at increased risk for CLL. Although interesting, the risk of progression to CLL among these individuals remains unknown and, even if MBL is detected and monitored, thus far, there is no evidence that early intervention for asymptomatic individuals has an effect on overall survival. Therefore, routine screening of relatives of CLL patients with MBL is currently not recommended, and they can be counseled that their risk of CLL and indolent NHL is low.
Hodgkin lymphoma
Classic Hodgkin lymphoma (HL) is morphologically characterized by clonal populations of neoplastic binucleated B cells, Reed-Sternberg cells, contained within a dense background of inflammatory cells. Antecedent exposure to EBV has been associated with the development of HL, especially the mixed cellularity subtype in children and older adults, but data suggest that there is also a heritable component to HL susceptibility. Familial clustering of HL is rare, but multiplex families have been identified in population-based registries and single or multi-institution case series. From these studies, first-degree relatives of probands have a 2.6- to 4.9-fold increased risk of HL compared with the general population, which increases further if the proband developed HL at less than 40 years of age. Twin studies also suggest a genetic component to HL risk. In 1 study of 179 monozygotic (MZ) and 187 dizygotic (DZ) twin pairs followed for 14 years in which 1 twin had been diagnosed with HL, 10 cases of HL occurred in previously unaffected MZ twins, but none in previously unaffected DZ twins, resulting in an impressive 100-fold increased risk of HL in an MZ twin of a proband. Most of these twin pairs were also concordant for HL subtype.
Risk within these HL families is not evenly distributed. In particular, siblings and cousins of probands are at greatest risk. This risk has been observed in both population-based registry studies, in which it is estimated that there is a 4.3- to 6.2-fold increased risk for siblings compared with a 1.2- to 3.1-fold increased risk for parents or offspring of probands, and in case series of multiplex families. Among siblings, same sex sibs are at even greater risk. Of the affected sibling pairs reported in the literature, sex concordance has been observed in 80 of 120 sib pairs. Based on data from population-based registries, the standardized incidence ratio is 8 for the brother of an affected brother and 11.8 for the sister of an affected sister, but only 1.37 for gender discordant sibling pairs.
This strong sex concordance has led to the hypothesis that an HL predisposition gene may be located on the pseudoautosomal region (PAR) of the sex chromosomes, which if inherited without recombination from a carrier father, results in gender concordant affected offspring. Evidence in support of this hypothesis includes (1) the finding that when gender was used as a covariate in a linkage study of 503 affected relatives from 112 HL families, a region in the PAR of the X chromosome was identified with a statistically significant logarithm of odds (LOD) score of 2.41 ; (2) the co-occurrence of HL in 1 pair of sisters affected by Leri-Weill dyschondrosteosis, a disorder caused by microdeletions in SHOX , a homeobox gene located in the PAR ; and (3) the localization to the PAR of MIC2 , which encodes CD99, a cell adhesion protein negatively regulated by EBV latent membrane protein 1 and, which when knocked down in B cells by expressing antisense CD99 mRNA, results in cells phenotypically similar to Reed-Sternberg cells.
Other candidate genes have been proposed in familial HL, most of which are involved in immune modulation. The most extensively studied of these are the HLA region genes on chromosome 6, which have been associated with both sporadic and familial HL. Reed-Sternberg cells are antigen-presenting cells that express class II antigens, and, in EBV-positive HL, also express class I antigens. It has been proposed that altered antigen presentation by the Reed-Sternberg cells could lead to activation of inflammatory cells and may underlie the inflammatory infiltrate characteristic of this disease (reviewed in Ref. ). Among both familial and sporadic HL, associations with multiple HLA class I and HLA class II alleles have been repeatedly reported, with A1, B5, B18, and DPB1 0301 the most consistently identified (reviewed in Ref. ). More recently, associations between specific HLA alleles and particular HL subtypes (eg, DRB1 1501-DQA1 0102-DQB1-0602 and nodular sclerosing HL ), and between specific HLA alleles and EBV status (eg, 2 HLA class I alleles near microsatellite markers D6S265 and D6S510 and EBV-positive HL ) have been reported. Despite these multiple associations, however, specific causative mutations have not been identified. This is largely because of strong LD within this vast region, containing more than 220 genes, which makes it difficult to distinguish disease-causing genetic variants from variants associated with disease by virtue of LD.
IL-6 and IL-12, 2 cytokines involved in immune system regulation, have also been implicated in HL risk, specifically among those developing HL as young adults. IL-6 has potent B-cell stimulatory properties and is secreted by Reed-Sternberg cells, and IL-12 stimulates cell-mediated immune responses. In HL twin pair studies, whereas inherited polymorphisms in IL-6 resulting in lower IL-6 blood levels were found to be protective against HL, polymorphisms in IL-12 resulting in lower IL-12 levels were associated with a nearly 3-fold increased risk of HL.
Because HL is a component of inherited immunodeficiency syndromes, the genes underlying these disorders have also been assessed for association with HL (see Table 1 ). Perhaps the best studied is autoimmune lymphoproliferative syndrome (ALPS, also known as Canale-Smith syndrome), an inherited disorder characterized by chronic lymphadenopathy, splenomegaly, autoimmunity, and a particularly high risk of HL (50-fold). Individuals with ALPS are frequently found to have germline mutations in the APT1 gene, encoding Fas, a cell surface receptor with a key role in initiating apoptosis in activated lymphocytes. In both in vitro and in mouse models, fas-mutant lymphocytes are resistant to fas-mediated apoptosis, which may result in inappropriate lymphocyte survival, enlarged lymphoid organs, and an increased risk of lymphoma.
Investigation of the fas pathway in 10 families with familial lymphoma, of which 3 had familial HL, led to the identification of another defect in this pathway in 2 families. In affected individuals compared with healthy controls, protein levels of caspase 8, an apoptotic initiator downstream of fas, were decreased relative to cFlip, a downstream apoptosis inhibitor. The mechanism accounting for this altered protein ratio was not identified nor was it determined if this defect was germline or acquired. Nonetheless, these data suggest that further investigation of the association between HL and both the fas pathway and deregulated lymphocyte apoptosis is warranted.
An additional candidate gene, KLHDC8B , a gene with previously unknown function located at 3p21, was identified by the mapping of a constitutional reciprocal translocation involving chromosomes 2 and 3 in a family with multiple individuals with HL. This translocation disrupted the 5′ portion of KLHDC8B and resulted in loss of gene expression. In addition, a heterozygous single nucleotide variant in the 5′ untranslated region of the same gene that led to decreased mRNA and protein levels was identified in 3 of 52 other multiplex HL families and was found to segregate with disease in the affected families. The protein was investigated and found to localize to the midbody of the cytoplasmic bridge linking dividing daughter cells during cytokinesis, and its depletion via RNA interference led to the formation of binucleated cells similar to HL’s characteristic Reed-Sternberg cells. The association between KLHDC8B and sporadic HL remains to be investigated.
In addition to these candidate gene studies, 1 group also undertook a genome-wide linkage study in HL families. They genotyped more than 1000 microsatellite markers in 254 individuals from 44 HL families and found evidence for linkage on chromosome 4p (peak LOD score 2.6, P = .0002), linking 43% of families under a recessive model. Other regions with less significant evidence of linkage were identified on chromosomes 4q, 7, and 17. Only modest linkage was seen on chromosome 6 near the HLA region (maximum NPL score 2), suggesting a more limited association of HLA in this larger population of HL families. Although all these studies have yielded some plausible candidate HL susceptibility genes, specific variants accounting for the increased risk of HL in these families have not yet been identified.
Clinically, there is limited information for HL families. They can, however, be counseled on the differing risks to specific family members so that those at the highest risk (ie, same sex sibs) can be closely followed. It must be kept in mind, however, that the lifetime risk of HL in the general population is estimated to be 0.24%. Thus, the overall lifetime risk for HL, even in same sex sibs, remains low at 2% to 3%. With regard to risk of other tumors, a small increased risk of NHL and CLL, as well as breast, ovarian, cervical, and brain tumors, was reported for relatives of individuals with HL in 1 population-based study ; these findings, however, await confirmation.
Hodgkin lymphoma
Classic Hodgkin lymphoma (HL) is morphologically characterized by clonal populations of neoplastic binucleated B cells, Reed-Sternberg cells, contained within a dense background of inflammatory cells. Antecedent exposure to EBV has been associated with the development of HL, especially the mixed cellularity subtype in children and older adults, but data suggest that there is also a heritable component to HL susceptibility. Familial clustering of HL is rare, but multiplex families have been identified in population-based registries and single or multi-institution case series. From these studies, first-degree relatives of probands have a 2.6- to 4.9-fold increased risk of HL compared with the general population, which increases further if the proband developed HL at less than 40 years of age. Twin studies also suggest a genetic component to HL risk. In 1 study of 179 monozygotic (MZ) and 187 dizygotic (DZ) twin pairs followed for 14 years in which 1 twin had been diagnosed with HL, 10 cases of HL occurred in previously unaffected MZ twins, but none in previously unaffected DZ twins, resulting in an impressive 100-fold increased risk of HL in an MZ twin of a proband. Most of these twin pairs were also concordant for HL subtype.
Risk within these HL families is not evenly distributed. In particular, siblings and cousins of probands are at greatest risk. This risk has been observed in both population-based registry studies, in which it is estimated that there is a 4.3- to 6.2-fold increased risk for siblings compared with a 1.2- to 3.1-fold increased risk for parents or offspring of probands, and in case series of multiplex families. Among siblings, same sex sibs are at even greater risk. Of the affected sibling pairs reported in the literature, sex concordance has been observed in 80 of 120 sib pairs. Based on data from population-based registries, the standardized incidence ratio is 8 for the brother of an affected brother and 11.8 for the sister of an affected sister, but only 1.37 for gender discordant sibling pairs.
This strong sex concordance has led to the hypothesis that an HL predisposition gene may be located on the pseudoautosomal region (PAR) of the sex chromosomes, which if inherited without recombination from a carrier father, results in gender concordant affected offspring. Evidence in support of this hypothesis includes (1) the finding that when gender was used as a covariate in a linkage study of 503 affected relatives from 112 HL families, a region in the PAR of the X chromosome was identified with a statistically significant logarithm of odds (LOD) score of 2.41 ; (2) the co-occurrence of HL in 1 pair of sisters affected by Leri-Weill dyschondrosteosis, a disorder caused by microdeletions in SHOX , a homeobox gene located in the PAR ; and (3) the localization to the PAR of MIC2 , which encodes CD99, a cell adhesion protein negatively regulated by EBV latent membrane protein 1 and, which when knocked down in B cells by expressing antisense CD99 mRNA, results in cells phenotypically similar to Reed-Sternberg cells.
Other candidate genes have been proposed in familial HL, most of which are involved in immune modulation. The most extensively studied of these are the HLA region genes on chromosome 6, which have been associated with both sporadic and familial HL. Reed-Sternberg cells are antigen-presenting cells that express class II antigens, and, in EBV-positive HL, also express class I antigens. It has been proposed that altered antigen presentation by the Reed-Sternberg cells could lead to activation of inflammatory cells and may underlie the inflammatory infiltrate characteristic of this disease (reviewed in Ref. ). Among both familial and sporadic HL, associations with multiple HLA class I and HLA class II alleles have been repeatedly reported, with A1, B5, B18, and DPB1 0301 the most consistently identified (reviewed in Ref. ). More recently, associations between specific HLA alleles and particular HL subtypes (eg, DRB1 1501-DQA1 0102-DQB1-0602 and nodular sclerosing HL ), and between specific HLA alleles and EBV status (eg, 2 HLA class I alleles near microsatellite markers D6S265 and D6S510 and EBV-positive HL ) have been reported. Despite these multiple associations, however, specific causative mutations have not been identified. This is largely because of strong LD within this vast region, containing more than 220 genes, which makes it difficult to distinguish disease-causing genetic variants from variants associated with disease by virtue of LD.
IL-6 and IL-12, 2 cytokines involved in immune system regulation, have also been implicated in HL risk, specifically among those developing HL as young adults. IL-6 has potent B-cell stimulatory properties and is secreted by Reed-Sternberg cells, and IL-12 stimulates cell-mediated immune responses. In HL twin pair studies, whereas inherited polymorphisms in IL-6 resulting in lower IL-6 blood levels were found to be protective against HL, polymorphisms in IL-12 resulting in lower IL-12 levels were associated with a nearly 3-fold increased risk of HL.
Because HL is a component of inherited immunodeficiency syndromes, the genes underlying these disorders have also been assessed for association with HL (see Table 1 ). Perhaps the best studied is autoimmune lymphoproliferative syndrome (ALPS, also known as Canale-Smith syndrome), an inherited disorder characterized by chronic lymphadenopathy, splenomegaly, autoimmunity, and a particularly high risk of HL (50-fold). Individuals with ALPS are frequently found to have germline mutations in the APT1 gene, encoding Fas, a cell surface receptor with a key role in initiating apoptosis in activated lymphocytes. In both in vitro and in mouse models, fas-mutant lymphocytes are resistant to fas-mediated apoptosis, which may result in inappropriate lymphocyte survival, enlarged lymphoid organs, and an increased risk of lymphoma.
Investigation of the fas pathway in 10 families with familial lymphoma, of which 3 had familial HL, led to the identification of another defect in this pathway in 2 families. In affected individuals compared with healthy controls, protein levels of caspase 8, an apoptotic initiator downstream of fas, were decreased relative to cFlip, a downstream apoptosis inhibitor. The mechanism accounting for this altered protein ratio was not identified nor was it determined if this defect was germline or acquired. Nonetheless, these data suggest that further investigation of the association between HL and both the fas pathway and deregulated lymphocyte apoptosis is warranted.
An additional candidate gene, KLHDC8B , a gene with previously unknown function located at 3p21, was identified by the mapping of a constitutional reciprocal translocation involving chromosomes 2 and 3 in a family with multiple individuals with HL. This translocation disrupted the 5′ portion of KLHDC8B and resulted in loss of gene expression. In addition, a heterozygous single nucleotide variant in the 5′ untranslated region of the same gene that led to decreased mRNA and protein levels was identified in 3 of 52 other multiplex HL families and was found to segregate with disease in the affected families. The protein was investigated and found to localize to the midbody of the cytoplasmic bridge linking dividing daughter cells during cytokinesis, and its depletion via RNA interference led to the formation of binucleated cells similar to HL’s characteristic Reed-Sternberg cells. The association between KLHDC8B and sporadic HL remains to be investigated.
In addition to these candidate gene studies, 1 group also undertook a genome-wide linkage study in HL families. They genotyped more than 1000 microsatellite markers in 254 individuals from 44 HL families and found evidence for linkage on chromosome 4p (peak LOD score 2.6, P = .0002), linking 43% of families under a recessive model. Other regions with less significant evidence of linkage were identified on chromosomes 4q, 7, and 17. Only modest linkage was seen on chromosome 6 near the HLA region (maximum NPL score 2), suggesting a more limited association of HLA in this larger population of HL families. Although all these studies have yielded some plausible candidate HL susceptibility genes, specific variants accounting for the increased risk of HL in these families have not yet been identified.
Clinically, there is limited information for HL families. They can, however, be counseled on the differing risks to specific family members so that those at the highest risk (ie, same sex sibs) can be closely followed. It must be kept in mind, however, that the lifetime risk of HL in the general population is estimated to be 0.24%. Thus, the overall lifetime risk for HL, even in same sex sibs, remains low at 2% to 3%. With regard to risk of other tumors, a small increased risk of NHL and CLL, as well as breast, ovarian, cervical, and brain tumors, was reported for relatives of individuals with HL in 1 population-based study ; these findings, however, await confirmation.
NHL
NHL encompasses a diverse group of more than 50 lymphomas with distinct morphologic, immunophenotypic, cytogenetic, genetic, and clinical features. Diffuse large B-cell lymphoma (DLBCL) and follicular lymphoma (FL) are the most common subtypes, accounting for 25% to 36% and 17% to 32% of NHL, respectively, in western countries. Ideally, investigations into the cause of NHL would examine each subtype separately to identify environmental and genetic risk factors unique to each lymphoma. This has not been feasible, however, because of the relative rarity of many NHL subtypes. In addition, the changing NHL classification systems have made it difficult to compare results from older and newer studies. The current World Health Organization classification system (2008), divides lymphoid neoplasms by cell of origin (eg, B vs T cell) and stage of lymphocyte development (precursor vs mature). This groups plasma cell disorders and CLL (ie, small lymphocytic lymphoma) with other mature B-cell NHLs. However, this section focuses on evidence for inherited susceptibility to NHL, excluding CLL and plasma cell disorders, which are addressed separately.
Despite significant research, the cause of most NHLs remains unknown. Lifestyle risk factors and the possible contribution of chemical and other environmental exposures to risk have been studied extensively, but except for the well-recognized association between NHL and infectious agents such as EBV and human immunodeficiency virus (HIV), the results of these studies have been controversial. That there is a heritable component to NHL is based on several observations. First, there are multiple reports of clustering of NHL with and without other types of hematopoietic malignancies in families (reviewed in Ref. ). Second, immigrants retain the NHL incidence patterns of their country of origin rather than adopting that of their new location. Multiple large population-based studies, including a pooled analysis of 17 studies conducted in the United States and Europe, have identified a 1.5- to 3-fold increased risk of NHL in first-degree relatives of patients with NHL. Other population-based studies, however, conducted in the United States and Iceland, did not confirm this increased risk, and the results of twin studies to determine whether the MZ twin of an individual with NHL is at greater risk than the DZ twin have been conflicting.
These inconsistencies are likely due in part to the heterogeneity of NHL itself. Risk factors almost certainly differ among different NHL subtypes, and both genetic risk factors and environmental exposures are also likely to differ among different ethnicities. To minimize disease heterogeneity, several large studies have assessed susceptibilities to the most common lymphoma subtypes. One large registry-based study from Sweden found a significantly increased risk of DLBCL in relatives of patients with DLBCL (relative risk [RR] 9.8), and an increased risk of FL (RR 4) and other indolent NHL (RR 2) among relatives of patients with FL. The largest population-based study undertaken to date, in which more than 10,000 patients with NHL were compared with 11,000 controls by the International Lymphoma Epidemiology Consortium (InterLymph), examined the risk of specific NHL subtypes in relatives of patients with NHL and found an increased risk of DLBCL (odds ratio [OR] 1.4) and FL (OR 1.8) among those with a family history of NHL, which increased if the affected case was male (OR 2 and 2.2 for DLBCL and FL, respectively) and increased even further if the case was a brother (OR 2.7 and 2.6). Relatives with a brother with NHL also had a statistically significant increased risk of marginal zone lymphoma (RR 6.1) and mantle cell lymphoma (RR 4.9). However, a much smaller study did not find increased risks for any subtype among those with a family history of NHL. These studies highlight the need for large numbers of cases to discover subtype- and population-specific risks.
Clues to the genetic basis of heritability in NHL have come from examining genetic syndromes with an increased risk of NHL. One such group of syndromes is caused by defects in DNA repair, and includes autosomal recessive disorders (gene defects noted in parentheses) such as ataxia telangiectasia ( ATM ), Bloom syndrome ( BLM ), Nijmegen breakage syndrome ( NBS1 ), and mismatch repair deficiency syndrome ( MSH2 , MLH1 , MSH6 , PMS2 ), in which affected individuals have a striking propensity to develop lymphomas at an early age. In addition, individuals with 1 defective copy of some of these same genes may also be at increased risk of NHL, suggesting a dosage effect. Heterozygous carriers of the NBS1 657del5 mutation, the founder Nijmegen breakage syndrome mutation in Eastern Europeans, for example, were enriched among Polish adults and children with NHL but not adults with NHL in the United States. Heterozygous carriers of mismatch repair gene defects have hereditary nonpolyposis colon cancer (Lynch syndrome) and are at risk for development of multiple tumor types, especially colon cancer. An increased risk of lymphoma in these patients has been controversial, but multiple reports of lymphoma in patients with Lynch syndrome exist, with 1 report suggesting that lymphoma in these patients is associated with an acquired inactivating mutation of the remaining wild-type copy of the mismatch repair gene.
Genetic syndromes with defects in immune function are also associated with a significant risk of NHL. Individuals with common variable immunodeficiency caused by defects in CD40 signaling, severe combined immunodeficiency disease caused by adenosine deaminase defects, Wiskott-Aldrich syndrome caused by WASP defects, and 2 X-linked immunodeficiency syndromes (X-linked lymphoproliferative syndrome and X-linked immunodeficiency with normal or increased immunoglobulin M [IgM]) all have a propensity to develop lymphoma, which is often striking (eg, 18% of patients with Wiskott-Aldrich syndrome develop lymphoma). In addition, patients with syndromes characterized by autoimmunity are also at risk. Individuals with ALPS are not only at risk for HL (50-fold increased risk) but they also have a 14-fold increased risk of NHL compared with the general population. The increased risk of NHL in patients with sporadically occurring medical conditions characterized by autoimmunity such as rheumatoid arthritis (RR 1.5–3.9), systemic lupus erythematosus (RR 4.6–8.4), Sjogren syndrome (RR 5.1–18.8), and celiac disease (RR 2.1–9.1) provides further evidence that pathways involved in autoimmunity have a role in lymphomagenesis (reviewed in Ref. ).
These observations have suggested potential candidate pathways for investigation into associations with NHL. To screen the large number of genes involved in the multiple proposed pathways in the genomics era, many groups have undertaken association studies comparing the frequency of a large number of individual SNPs located in candidate genes in patients affected with NHL versus controls. To date, the most consistently replicated finding has been the association between SNPs in the inflammatory response pathway ( TNF and LTA ) and in the immunoregulatory gene, IL10 , and NHL, in particular DLBCL. The largest candidate gene association study in NHL was undertaken through the InterLymph Consortium and examined TNF family SNPs in more than 3500 cases and 4000 controls, mostly of European descent, pooled from 8 separate case-control studies. This study found a statistically significant increased risk of NHL with SNPs in TNF (−308G>A, OR 1.19, P = .005) and IL10 (−3575 T>A, OR 1.11, P = .037) in the pooled analysis. These same SNPs were associated with an even greater risk of DLBCL (OR 1.33, P = .00021, and OR 1.22, P = .006, respectively). An SNP in LTA (252 A>G), which is in LD with the TNF −303G>A SNP in the HLA III region on chromosome 6p21, was also associated with an increased risk of NHL (for homozygous GG: OR 1.18, P = .11) and DLBCL (for homozygous GG: OR 1.47, P = .001). These associations have been confirmed in other studies undertaken primarily in individuals of European descent, strengthening the evidence that these regions are associated with increased risk for NHL.
Other candidate gene association studies have been performed looking at cell cycle, apoptosis, lymphocyte development, DNA repair, immune system regulation, metabolism (both 1 carbon and xenobiotics), hormone production, and oxidative stress pathways. A review of these studies is beyond the scope of this article (see Ref. for an excellent review), but some of the more recently identified positive associations are highlighted here. For NHL as a group, associations with SNPs in or near several other genes within the TNF/NFκB pathway ( NFKBIL1 , FAS , IRF4 , TNFSF13B , TANK , TNFSF7 , and TNFRSF13C ), within lymphocyte development and immune system regulation ( BCL6 , BCL2L11 , PIM1 , and BCL7A ), within apoptosis pathways ( CASP1 , CASP8 , and CASP9 ) and within innate immunity pathways ( TLR10-TLR1-TLR6 gene cluster ) have been reported. In addition, a deletion polymorphism in a glutathione S -transferase (GSTT1 0), an enzyme involved in detoxification of reactive oxygen species, has been found to increase NHL and HL risk in those homozygous for this polymorphism in several studies (reviewed in Ref. ). Among specific NHL subtypes, associations have been identified for DLBCL ( IL10 , TNF , LTA as described, as well as BCL7A and PIM1 ), FL ( BCL2L11 , BCL6 , FAS , TNFSF7 , TNFRSF5 ), and marginal zone lymphoma ( TLR4 , TNFRSF13C , BCL6 ).
Familial clustering of lymphoplasmacytic lymphoma/Waldenstrom macroglobulinemia (LPL/WM) has also been described. In these families, unaffected family members have a low-level IgM monoclonal gammopathy of unknown significance (MGUS), which is characterized by detection of a low-level monoclonal immunoglobulin in the serum without evidence of multiple myeloma (MM)-related end-organ dysfunction and less than 10% plasma cells in the bone marrow, at a much higher rate than in the general population (3.2–6.3% vs 0.25–0.64%, respectively), suggesting that this may be a precursor condition. A population-based case-control study from Sweden examining 2144 LPL/WM cases and more than 24,000 controls found a 20-fold increased risk of LPL/WM in first-degree relatives of cases. In addition, relatives were at increased risk of NHL (RR 3), CLL (RR 3.4), and MGUS (RR 5), suggesting shared susceptibilities for these disorders. Relatives of LPL/WM patients were not at increased risk for MM, despite known associations between MGUS and MM. A genome-wide linkage analysis performed on 11 LPL/WM families found evidence suggestive of linkage at 1q and 4q (NPL 2.5 and 3.1, respectively) and less strongly at 3q and 6q, which may suggest that these regions harbor susceptibility alleles for LPL/WM, as well as associated conditions such as CLL and MGUS.
Clinically, genetic counseling is currently of limited value for NHL families. Detailed family and medical histories are essential, especially in children, to rule out any of the known genetic syndromes with a predisposition to NHL. For those families with specific subtypes of NHL, they can be counseled regarding subtype specific risks. For example, families with LPL/WM can be counseled about the increased risk of IgM MGUS and can be screened for this condition. If IgM MGUS is diagnosed, monitoring is necessary because of the increased risk for LPL/WM (1.5% per year). The identification of highly penetrant NHL families may ultimately yield families large enough for linkage analysis. In addition, GWAS in NHL are currently underway, which will contribute significantly to our understanding of the heritable contribution to risk in NHL.
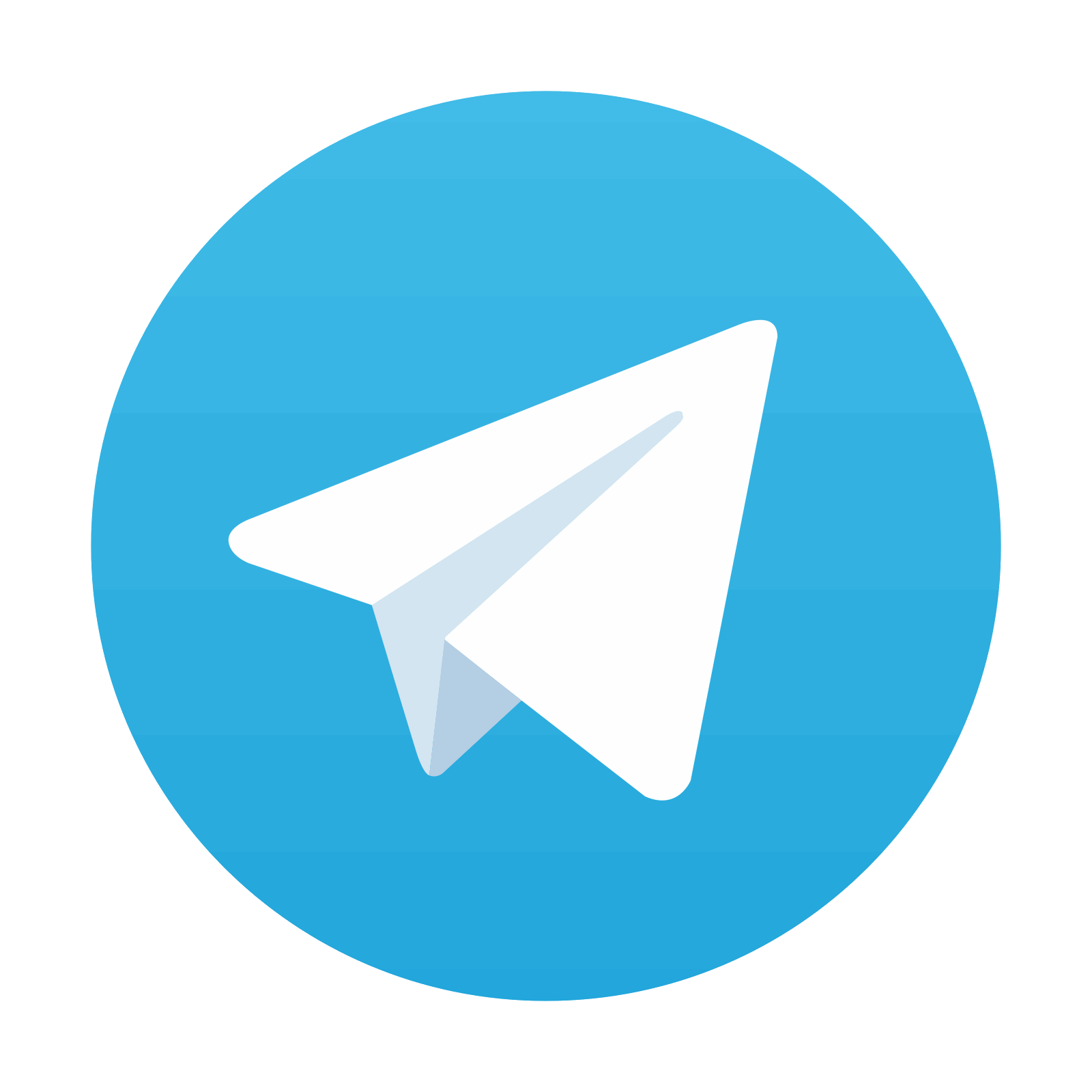
Stay updated, free articles. Join our Telegram channel

Full access? Get Clinical Tree
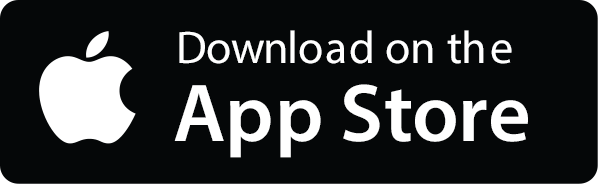
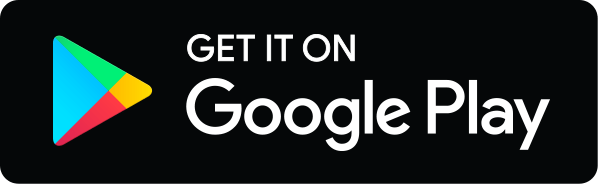