Primary liver tumors are a common clinical problem in the United States and worldwide. Resection has historically been used to treat liver lesions. Commonly used liver-directed therapies include transarterial chemoembolization, selective internal radiation therapy, and ablative therapy. Only ablative therapy can cause direct destruction of the targeted tissue. The commercially available modalities in the United States are all based on thermoablative technology. This article examines the various ablative technologies and their application, as well as how these procedures can be performed safely and with optimal outcomes, in a community cancer center.
Primary liver tumors are a common clinical problem with a steadily increasing incidence in the United States and worldwide. The estimated death rate from primary liver and intrahepatic bile duct tumors has increased in both men and women in the United States in 2010, and the incidence of liver tumors is much higher if other malignancies that routinely metastasize to the liver are taken into account. Whether primary liver cancer or metastatic disease, if left untreated, the prognosis is dismal for these patients. Resection has historically been considered the gold standard treatment for liver lesions; however, the current treatment options have been expanded to include a wide array of liver-directed therapies. Today, commonly used liver-directed therapies include transarterial chemoembolization (TACE), selective internal radiation therapy (SIRT), and ablative therapy. All these treatment modalities can provide disease control depending on the cause of tumor. Among these different liver-directed therapy options, only ablative therapy can cause direct destruction of the targeted tissue. The commercially available modalities readily accessible to surgeons in the United States today are all based on thermoablative technology: cryosurgical therapy, monopolar radiofrequency ablation (RFA), and microwave ablation (MWA). All the 3 modalities can achieve tumor destruction; however, each modality carries distinct physical properties. Physicians should familiarize themselves with each technology to maximize its efficacy for clinical application. This article examines the various ablative technologies and their application, as well as how these procedures can be performed safely and with optimal outcomes, in a community cancer center.
Basic principles of thermoablative technology
Human cells are susceptible to thermal injury, and permanent cell damage is caused at extreme temperatures (<−100°C or >50°C). Cell exposure to temperatures greater than 50°C, even for as short as 4 to 6 minutes, results in protein denaturation that causes irreversible cell death. The formation of intracellular ice crystals, when cells are subjected to temperatures less than −40°C, causes lethal cell damage by microcirculatory failure and cellular necrosis. This article discusses the interaction of each therapy with local tissue factors and how this interaction plays a role in determining the final ablative zone.
Cryosurgical therapy
The application of cryosurgical ablation began in the 1960s, and the cryogens commonly used were nitrous oxide, liquid nitrogen, and argon. The general setup of cryosurgical devices use a segmental insulated probe through which the cryogen is delivered, causing rapid expansion of the cryogenic gas, with rapid cooling to temperatures approaching −100°C in a few seconds. The common cryogens used for hepatic ablation are liquid nitrogen and argon, with the latter using a smaller-size probe and a more-rapid freeze cycle. The small size of its applicator permits percutaneous use. The thawing of the resulting ice ball is facilitated by instillation of helium gas. It is recommended that a rapid freeze, followed by a slow thaw, then repeat freeze-thaw cycles be used for maximal effect. The advantage of cryosurgery is its ability to be visualized on computed tomography (CT) and magnetic resonance imaging (MRI) because of ice ball formation. A 3-cm ice ball can be generated through a single applicator, and larger areas of freezing can be achieved by overlapping multiple applicators. A niche application of cryosurgical ablation is for lesions located adjacent to a major vessel because the heating effect of flowing blood is protective to the vessel.
Cryosurgical therapy
The application of cryosurgical ablation began in the 1960s, and the cryogens commonly used were nitrous oxide, liquid nitrogen, and argon. The general setup of cryosurgical devices use a segmental insulated probe through which the cryogen is delivered, causing rapid expansion of the cryogenic gas, with rapid cooling to temperatures approaching −100°C in a few seconds. The common cryogens used for hepatic ablation are liquid nitrogen and argon, with the latter using a smaller-size probe and a more-rapid freeze cycle. The small size of its applicator permits percutaneous use. The thawing of the resulting ice ball is facilitated by instillation of helium gas. It is recommended that a rapid freeze, followed by a slow thaw, then repeat freeze-thaw cycles be used for maximal effect. The advantage of cryosurgery is its ability to be visualized on computed tomography (CT) and magnetic resonance imaging (MRI) because of ice ball formation. A 3-cm ice ball can be generated through a single applicator, and larger areas of freezing can be achieved by overlapping multiple applicators. A niche application of cryosurgical ablation is for lesions located adjacent to a major vessel because the heating effect of flowing blood is protective to the vessel.
Monopolar RFA
The most widely used thermoablative technology worldwide is monopolar RFA, which uses an electrosurgical device operating at 200 to 1200 kHz on the electromagnetic spectrum. It is estimated that 90% of all ablations performed in the United States are monopolar RFAs. Monopolar RFA requires a closed circuit to function, which means grounding pads (active electrodes) must be applied to the patient, similar to electrosurgery. Alternating current is passed between the RFA electrode and the grounding pad. The rapidly oscillating current passing between the electrode and the grounding pad causes agitation of intracellular ions and leads to frictional heat formation. The heat energy generated leads to coagulative necrosis by conduction and is concentrated around the electrode because of higher current density secondary to its smaller surface area.
The clinical effects of RFA can be heavily influenced by the location of the lesion because current follows the path of least electrical resistance. This property can create a clinical problem when the electrode is in close proximity to a large blood vessel because blood vessels have lower impedance than the surrounding tissue. Current can be diverted away from the target area under these conditions, which can lead to smaller or more irregular ablation zones than anticipated. This phenomenon is known as electrical sink, a concept similar to thermal sink, in which heat is being shunted away by the flowing blood.
The rapid increase in tissue temperature around the RFA electrode can limit the efficacy of RFA because of tissue desiccation and charring. During RFA, it is imperative to maintain the target tissue temperature at less than 100°C to prevent tissue vaporization and carbonization, which increases the impedance significantly and hinders current flow. The commercially available systems optimize power output through an impedance-based or output-based system. These improvements in design for RFA allow an ablation diameter up to 4 to 5 cm with multiple electrodes used in combination; however, the local tissue effects discussed earlier may still decrease or alter the final ablative volume. Other improvements to RFA include the use of multiprong electrodes to allow larger current deposition and more predictable heating, as well as coupling of the electrodes to temperature or impedance sensors to allow real-time feedback and adjustment of energy output based on a preset algorithm to achieve effective ablation. Saline infusion within the shaft of the electrode can reduce the tissue temperature for a better electrode-tissue interface, and pulsed delivery of radiofrequency energy has also been developed to maximize current deposition. The next generation of radiofrequency technology is the development of bipolar RFA, and it is currently being used in selected centers in the United States. The deployment of 2 electrodes completes the circuit required; therefore, no grounding pad is necessary for bipolar RFA. However, reports on the clinical results for bipolar RFA are currently lacking.
MWA
MWA also uses radiofrequency energy, but it operates at a much higher frequency (900 MHz–10 GHz) than RFA. The energy deposition for MWA is also completely different from that for RFA. The MWA antenna broadcasts nonionizing radiation to achieve tissue destruction, and a grounding pad is not necessary because there is no flow of current. Heat generation by MWA is effected by water molecules, which orient themselves within the applied electromagnetic field. The dipole characteristic of water molecules causes them to orient toward the polarity of the rapidly changing electromagnetic field. This rapid alternation in polarity generates heat energy via vibration in a process termed dielectric hysteresis.
The distinct characteristic of MWA is its ability to homogeneously broadcast energy throughout its near field, which is largely defined by the microwave frequency and antenna configuration. All tissues in this zone are heated homogenously without being influenced by local tissue factors such as close proximity to blood vessels. These properties distinguish MWA from RFA and render MWA a more attractive option for hepatic ablation. However, in tissue that does not fall within the volume of the near field of microwave, the heating is conductive (as in RFA), and the tissue is hence susceptible to thermal sink. Given the superior physical properties and ease of use, microwave technology will replace monopolar RFA in the treatment of liver tumors.
Three microwave frequencies have been approved for clinical use in the United States: 915 MHz, 2.45 GHz, and 9.2 GHz. Only the manufacturers of the 915-MHz (Valleylab, Boulder, CO, USA) and 2.45-GHz (Acculis Ltd, Microsulis Medical Ltd, Denmead, UK) systems have US Food and Drug Administration (FDA)-approved commercially available systems for liver tumor ablation, and the authors use both systems in their institution. The 915-MHz system uses a solid-state amplifier to generate microwave energy, whereas the 2.45-GHz system uses a magnetron. Both systems have proved to be effective in the authors’ experience, as each system has its distinct properties and advantages. The difference in frequency theoretically permits deeper tissue penetration with the 915-MHz system, whereas the 2.45-GHz system is capable of higher energy deposition within the electromagnetic field given a similar wattage. The selection of system depends on the number of lesions and their location. The 915-MHz system uses a smaller antenna (13 gauge), which allows percutaneous application. It is often used in a clustered triple antenna formation to treat larger (>3-cm diameter) lesions, with the customary settings at 45 W for 10 minutes powered by 3 different 915-MHz generators. The 2.45-GHz system has a larger size (5-mm diameter) antenna and can produce comparable ablative sizes with 100 W over a 5-minute setting with 1 antenna alone. A smaller (1.8 mm) percutaneous antenna for the 2.45-GHz system has recently been approved by the FDA, which may expand its clinical usage.
Experimental modalities
High-intensity focused ultrasonography (HIFU) and irreversible electroporation (IRE) are experimental modalities on the horizon for hepatic ablation that are showing some promise. The arrival of these new technologies may supplement current technology and help define niche application for each modality in treating liver tumors.
HIFU
The attractive feature of HIFU is its ability to achieve coagulative necrosis without using an invasive applicator. The probe of HIFU can focus an ultrasound beam to an intensity of 100 to 10,000 W/cm 2 and increase its peak compression and rarefaction pressure up to 30 and 100 MPa, respectively. Comparatively, diagnostic ultrasonography uses only 0.1 to 100 mW/cm 2 or compression and rarefaction pressures of 0.001 to 0.003 MPa, depending on the mode of imaging. The acoustic energy is then converted to heat energy within the tissue, and coagulative necrosis is achieved within a focal region. The energy distribution of HIFU is confined to within 1 mm in diameter. The clinical advantage of such a precise application is that the effect of undesired thermal spread can be limited, which is often the undesired effect of other thermoablative technologies. HIFU was first developed to treat other areas of the body, such as the eye, prostate, and kidney. Initial investigation of HIFU in ablating liver lesions has shown some promising results. A randomized trial has compared TACE alone with TACE and HIFU for the treatment of hepatocellular cancer. The HIFU-TACE group demonstrated a survival benefit of 6 months over the TACE-alone group. Research is ongoing to define its role in treating hepatic tumors.
Irreversible Electroporation
Irreversible electroporation (IRE) is a distinct class of ablative therapy because it does not cause tissue necrosis by a thermal means. IRE works by delivering a high-voltage differential (1000–3000 V) across 1 cm for the duration of milliseconds to microseconds, inducing nanodefects on the cell membrane. The permanent defects disrupt cell homeostasis, and cell death ensues. The effect of IRE is different among different tissue types. Whereas IRE spares bile ducts and blood vessels, its primary effect is on the liver parenchymal cells. There are animal studies to support IRE as an ablative option, but clinical trials are currently lacking. Recently, a commercially available machine has been introduced in the United States, which makes IRE an exciting new option for liver tumor ablation.
Outcome for ablative therapies
Hepatocellular Carcinoma
Hepatocellular carcinoma (HCC) poses a significant clinical problem worldwide and in the United States because of its tumor biology and increasing incidence. HCC is the third leading cause of cancer death worldwide, and its incidence is steadily increasing in the United States. It is believed that viral hepatitis, especially hepatitis C, along with obesity and alcohol abuse, contributes significantly to the development of cirrhosis in the United States, which is the leading risk factor for developing HCC.
The clinical spectrum of HCC is diverse, and no staging scheme has been successful in accurately describing all the scenarios. However, HCC can usually be classified into local or regional disease and intrahepatic or extrahepatic metastases while factoring in patients’ underlying hepatic function. Local disease usually refers to a single small lesion, and the treatment intent is curative. The goal would be to remove the tumor, either by resection or by ablation. However, if patients have advanced disease, the focus should be on disease control, and patients can benefit from some combinations of liver-directed therapy.
There are 3 main treatment strategies for HCC depending on the clinical stage, each of which is potentially curative: resectional therapy (RT), orthotopic liver transplant (OLT), and ablative therapy. Historically, RT and OLT have the best 5-year survival rate, with up to a reported rate of 70%. However, only select patients are candidates for RT, and many patients cannot undergo resection because of underlying parenchymal disease with inadequate hepatic reserve, multiple tumors, unfavorable tumor location, and/or other prohibitive medical comorbidities. Patients who are candidates for RT usually have a small tumor and normal liver function, and this is the recommended treatment option by the European Association for the Study of the Liver and the American Association for the Study of Liver Diseases. The recurrence rate for RT at 5 years, however, can reach as high as 70%, especially for patients who have tumors larger than 5 cm, 3 or more tumors, vascular invasion, and/or a narrow resection margin.
There are strict selection criteria for OLT, the most widely used being the Milan criteria. These patients have either a single HCC of up to 5 cm or up to 3 tumors, with none more than 3 cm. OLT has the advantage of treating both the HCC and the underlying liver disease; however, the time on the wait list can be variable, and up to 10% to 30% drop out rates have been reported. Effort has been made to allocate additional MELD (model of end stage liver disease) score points in hopes of shortening the waiting period for patients with HCC. These additional MELD points are maintained following ablation of HCC, whereas they are lost following resection, making ablation the preferred treatment modality for lesions smaller than 5 cm as a bridge to transplant. However, the scarcity of suitable organs remains a major hurdle for the widespread implementation of OLT as a treatment option.
Ablation therapy has been extensively studied in the treatment of HCC and demonstrated to provide adequate locoregional disease control in patients with small-volume disease alone. Patients who cannot undergo resection or who are poor surgical candidates can also benefit from ablative therapy as one of the components of liver-directed therapy for disease control, which commonly entails selective internal radiation therapy, TACE, or portal vein embolization.
Ablative therapy for HCC has evolved from cryosurgical therapy and monopolar RFA to now include MWA. The series confirming the role of cryosurgical ablation for HCC mostly involve open intraoperative application in the 1990s, and good results were reported with tumors less than 5 cm in diameter. The 1-, 3-, and 5-year survival rates of patients with tumors smaller than 5 cm were reported as 97%, 77%, and 55%, respectively, in a series of 235 patients. However, for patients with tumors larger than 5 cm, the 5-year survival rate was only 25%. Percutaneous application of cryosurgical ablation for treating HCC is not widely reported. Patients with a tumor burden replacing more than 40% of the liver content are poor candidates for cryotherapy. One devastating complication for cryosurgical therapy is cryoshock, which entails severe coagulopathy and multiorgan failure. The incidence of cryoshock is estimated at 1%, but it carries a 28% reported mortality. Cracking of the ice ball (<25%), hemorrhage (<13%), bile fistula/biloma (<10%), abscess (0%–9%), pleural effusion (4%–18%), and death (0%–8%) are other observed complications. Despite the proven clinical value of cryosurgical therapy, it has been replaced by thermoablative therapy (monopolar RFA and MWA), because of their ease of use, better safety profile, and shorter application time.
The most studied modality in ablative therapy for HCC is monopolar RFA, which accounts for 90% of all liver ablation worldwide. The 3 commercially available systems (AngioDynamics, Latham, NY, USA; Boston Scientific, Natick, MA, USA; and Valleylab) have all been shown to be equally effective for HCC up to 3 cm. Excellent results have been reported for treating HCC with RFA for different tumor sizes: less than 3 cm, 3 to 5 cm, and greater than 5 cm. There have been reports suggesting that RFA should be the treatment of choice for patients with small HCC (<2 cm), including those who have Child class A cirrhosis. In one retrospective study of 218 patients, the local recurrence rate was 2.8% and complication rate was 1.8%. There have been randomized trials suggesting that survival rates for patients with small HCC were similar when treated with RT or RFA. However, the recurrence rate of RFA could be as high as 14.9% as reported in a large meta-analysis of 2369 cases of HCC ablation. One of the challenges for treating small HCC by RFA is the difficulty in targeting the small lesion, especially via the percutaneous route. Another concern is that RFA has an inferior physical profile (compared with MWA, as discussed earlier), which renders incomplete ablation possible. The advancement of modern ablative technology and the improvement in lesion targeting would improve the local recurrence rate.
The treatment of HCC using MWA is still in its early stage in the United States, but extensive experience using MWA in Asia and Europe has been reported with good results. The FDA first approved the clinical application of MWA in 2008 following clinical trials that confirmed the efficacy of MWA in producing complete tumor destruction. These findings were confirmed by immunohistochemical analysis in the ablation zone in all 11 patients who underwent hepatoma ablation followed by resection, with an average reported ablation diameter of 5.5 cm. A subsequent phase 2 trial enrolled 87 patients, of which 23 had HCC, and at a mean follow-up of 19 months, 47% of patients were alive without evidence of disease. The reported local recurrence was 2.3%. A similar local recurrence rate (2%) was observed in another series. MWA also demonstrated a promising survival rate: 1-, 3-, and 5-year survival rates were reported as 97%, 81%, and 43%, respectively. Other series have examined the role of MWA in larger lesions, and a 44% 3-year survival rate has been reported in a series of 69 patients with HCC up to 4 cm in diameter.
Colorectal Liver Metastases
Each year, more than 140,000 cases of colorectal cancer are diagnosed in the United States, and there are 50,000 cancer deaths related to colorectal cancer yearly. It is the third leading cancer-related cause of death, and many patients (up to two-thirds) develop metastases, most commonly to the liver.
The treatment of colorectal liver metastases (CRLM) is evolving, and the choice of treatments depends on multiple factors, including tumor burden, tumor location, number of lesions, and tumor responsiveness to chemotherapeutic agents. Ideally, patients who are amenable to resection should receive RT, but only 10% to 25% of patients are candidates for RT. The advancement in neoadjuvant chemotherapeutic agents has demonstrated that up to 38% of patients can potentially be down staged and become candidates for RT. The survival rate for patients undergoing hepatic resection has improved significantly compared with earlier studies, with reported 5- and 10-year survival rates as high as 71% and 60%, respectively. Treatment strategies focused on liver-directed therapy have been developed to provide alterative treatments for disease control. Ablative therapy, in conjunction with TACE and SIRT, has significantly improved the survival of these patients, who otherwise would have a 5-year survival rate of 0% to 1% if left untreated.
There are case series reporting the treatment of CRLM with cryosurgical and ethanol ablation with good results, but the newer modalities, RFA and MWA, have largely made ethanol and cryosurgical ablation an historical interest only. As with HCC, RFA is the most studied modality for treating CRLM, with excellent 1-, 2-, and 3-year survival rates reported as 87%, 77%, and 50%, respectively. Multiple other series have confirmed the role of RFA as an effective treatment for CRLM. Long-term survival meta-analysis data for RFA have shown that percutaneous application can lead to lower rates of local disease control and an increased risk of tumor tracking. This study also suggested that surgical RFA is an effective treatment for CRLM less than 3 cm, with results comparable with RT. It is believed that the high recurrence rate for RFA may be intrinsic to its physical limitation, especially if perivascular lesions are treated. The addition of MWA, along with image guidance for lesion targeting, should minimize the risks of local recurrence and improve overall survival.
Clinical studies have emerged in the literature validating MWA as an effective treatment for CRLM. The local recurrence and 3-year survival rates reported are encouraging, and a small randomized controlled trial (n = 30) has demonstrated that MWA and RT have similar overall survival rates. Patient management for CRLM should involve a multidisciplinary approach to determine the best treatment plan. Generally, a cytoreductive strategy is advocated if the morbidity and mortality risks of the treatment are low. However, the underlying biology of CRLM is different for each patient, and treatment should be tailored individually. Aggressive tumor biology of CRLM has been defined, and features such as positive margins, extrahepatic disease, node-positive primary, disease-free interval from primary to metastasis of less than 12 months, more than 1 hepatic tumor, largest hepatic tumor greater than 5 cm, and carcinoembryonic antigen level greater than 200 ng/mL are associated with a poor outcome with RT. However, even for patients with extrahepatic disease, adequate locoregional disease control can still improve outcomes. MWA provides an effective option for treating CRLM, as it has a lower complication profile than resection and is capable of complete tumor destruction.
Neuroendocrine Tumors and Metastasis from Other Primary Tumors
Neuroendocrine tumors and other primary cancers, such as breast carcinoma and genitourinary tumors that metastasize to the liver, are traditionally evaluated for hepatic resection. Improved survival has been reported in selected patients who undergo RT for noncolorectal/nonneuroendocrine metastases. In regards to neuroendocrine metastases, not unlike HCC and CRLM, 90% of these patients are not candidates for hepatic resection, and many of them remain symptomatic from local and endocrine effect. Curative and palliative resections are possible only in 20% to 25% of these patients, but these resections can relieve symptoms for more than 2 years in 90% of the patients. Five-year survival is prolonged to 40% to 85%, although metastases recur or progress in almost all patients. RFA has been used for endocrine symptom palliation, and 70% to 90% of patients have a good effect for 2 years. Other liver-directed therapies, such as chemoembolization and embolization, have been used to treat patients not amenable to surgical treatment (RT and RFA), with an observed 50% tumor burden reduction and 60% 5-year survival rates.
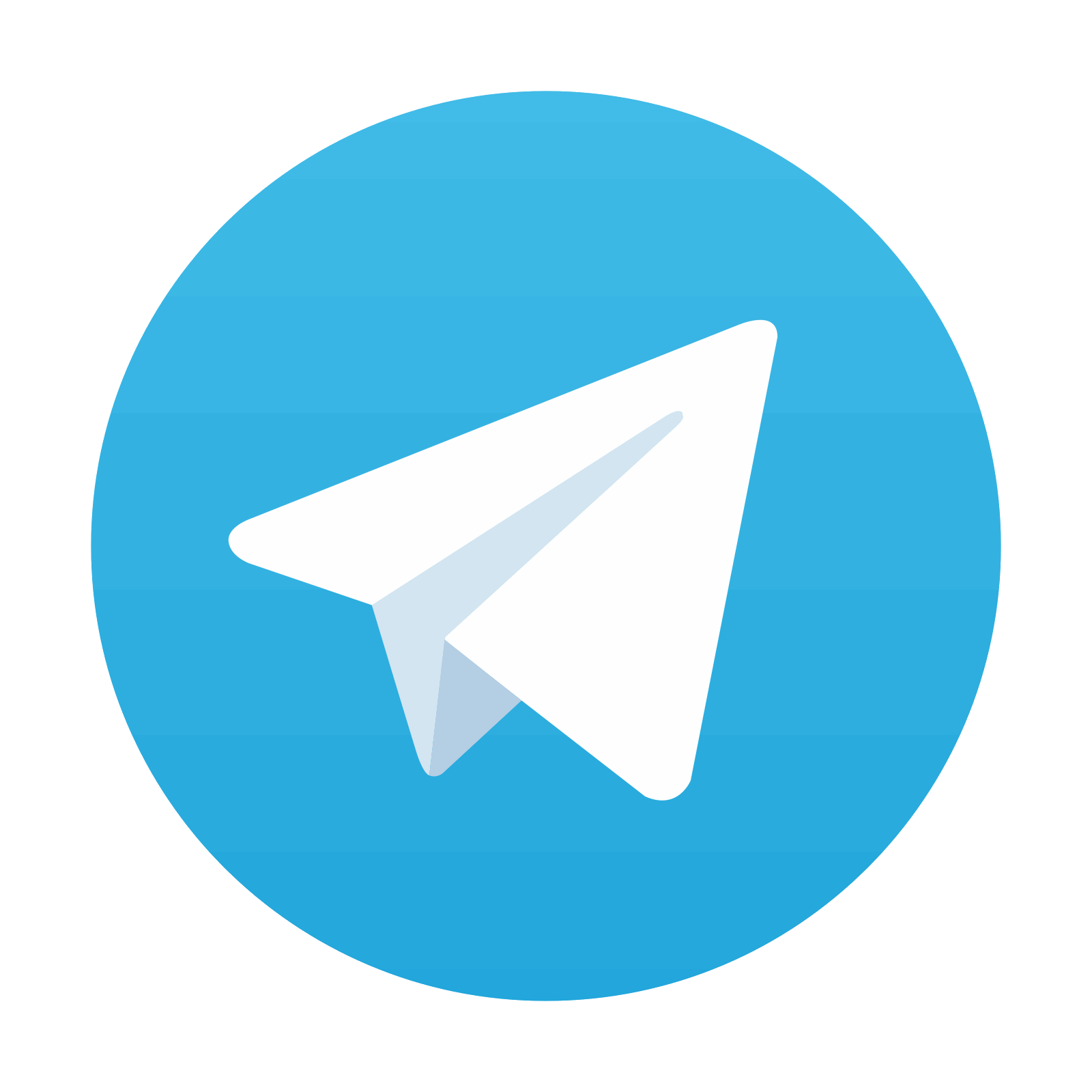
Stay updated, free articles. Join our Telegram channel

Full access? Get Clinical Tree
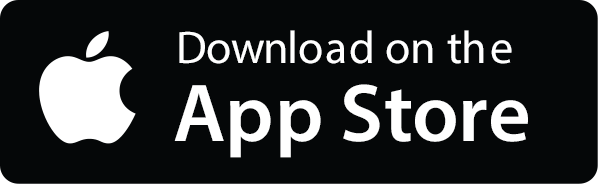
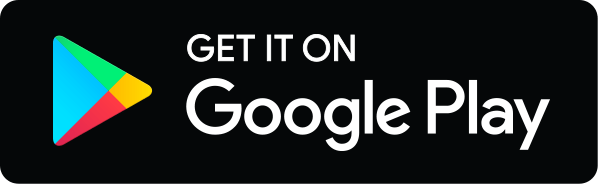