To date, hepatic artery infusion (HAI) chemotherapy has primarily been investigated in the setting of colorectal cancer liver metastases (CRLM). Few studies have been conducted in North America regarding HAI chemotherapy for primary liver cancers (PLC) or noncolorectal liver metastases (non-CRLM). Despite decades of evaluation, controversy surrounding the use of HAI chemotherapy still exists. In this article the methods of HAI chemotherapy delivery, technical aspects of catheter and pump insertion, and specific complications of HAI chemotherapy are discussed. Outcomes of clinical trials and reviews of HAI chemotherapy in the setting of CRLM, PLC, and non-CRLM are evaluated.
Key points
- •
The predominant blood supply of primary and metastatic tumors of the liver is the hepatic artery.
- •
Administration of chemotherapeutic agents via the hepatic artery allows preferential delivery of cytotoxic drugs to tumor cells with a relative sparing of normal hepatocytes.
- •
Optimal drug delivery via surgically inserted hepatic artery catheters and implantable pumps is technically feasible, and in experienced centers is associated with acceptable morbidity.
- •
Evidence suggests that hepatic artery infusion (HAI) chemotherapy has a role in the treatment of both unresectable colorectal cancer liver metastasis (CRLM) and as adjuvant therapy in resectable CRLM.
- •
Trials of HAI chemotherapy for the treatment of primary liver cancer and non-CRLM are few, and its use in this setting requires further study.
Introduction
The liver is unique in that it receives blood from the systemic arterial circulation and the enterohepatic circulation. Most blood flow to normal hepatocytes is derived from the portal venous system; alternatively, liver tumors obtain their nutrient blood supply almost exclusively from the hepatic artery (HA). For decades, attempts to take advantage of this dual blood supply to selectively deliver regional therapy to tumors isolated to the liver have been made. In the early 1960s, initial trials of continuous hepatic artery infusion (HAI) chemotherapy for the treatment of liver cancers were reported by Sullivan and colleagues. Though small and inclusive of multiple tumor types, these seminal investigations indicated that HAI chemotherapy was feasible, and that there appeared to be a quantifiable tumor response. Unfortunately, general applicability was limited owing to a lack of standardized chemotherapeutic regimens and tumor response measurements. Furthermore, clinical implementation was hampered by unacceptably high complication rates related to catheter insertion, prolonged infusions, and cumbersome external infusion pumps. Despite the limitations of these early investigations, 3 essential components for safe and effective implementation of HAI chemotherapy were identified: (1) insertion of a durable arterial catheter that allows prolonged use; (2) identification of appropriate drugs; and (3) a delivery system that allows protracted, reliable, and convenient drug administration.
The primary goal of HAI chemotherapy is to selectively deliver high concentrations of drugs to cancer cells while limiting drug exposure to normal hepatocytes. To achieve this, drugs used for HAI chemotherapy must have quantifiable antitumor activity and an optimal pharmacokinetic profile characterized by: (1) high first-pass hepatic extraction; (2) short plasma half-life; and (3) first-order kinetics with steep dose-response curves. To date, several suitable agents with activity against primary and metastatic liver cancers have been evaluated in the setting of HAI chemotherapy. Pharmacokinetic profiles of these commonly used drugs are provided in Table 1 . Relative to other agents, delivery of floxuridine (FUDR) via the HA is most favorable. HAI chemotherapy with FUDR results in 100- to 400-fold greater exposure within the liver and a 15-fold higher drug concentration within tumor cells in comparison with normal hepatocytes. Up to 92% is extracted by the liver on first pass, limiting systemic exposure, and it is rapidly eliminated from the body.
Drug | Hepatic Extraction (%) | Plasma Half-Life (min) | Fold Increase Exposure with HA Infusion | Retained Systemic Exposure Relative to IV Administration (%) |
---|---|---|---|---|
5-Fluorouracil (5-FU) | 22–45 | 10 | 5–10 | 60–70 |
Floxuridine (FUDR) | 69–92 | <10 | 100–400 | <5 |
Mitomycin C (MMC) | 22 | ≤10 | 6–8 | 80–90 |
Cisplatin (CDDP) | — | 20–30 | 4–7 | 100 |
Doxorubicin | 45–50 | 60 | 2 | 40–50 |
Successful HAI chemotherapy is contingent on both the use of an effective drug and the ability to place a durable HA catheter, allowing safe, long-term drug delivery. At present HA catheters are primarily inserted via open laparotomy. In efforts to combat the morbidity of a major abdominal operation, percutaneous techniques have also been used. A recent study by Arru and colleagues compared transaxillary percutaneous (PCT) HA catheter insertion with insertion via laparotomy (LPT). Length of hospitalization and analgesia requirement was greater in the LPT. However, catheter-related complications resulting in delay or cessation of treatment were seen in 43% of the PCT group, compared with 7% of the LPT group ( P = .005). This finding translated into significantly fewer HAI chemotherapy cycles in the PCT group than in the LPT group (4.3 vs 6.5, P = .038). It seems that despite the initial morbidity of a major abdominal procedure, LPT-placed catheters are more durable and allow for more consistent long-term drug delivery. More recently, minimally invasive techniques for HA catheter insertion have been described. In a single-center review of 27 patients undergoing laparoscopic placement of HA catheters, correct catheter placement was observed in 100% of cases, average operative time was 45 to 55 minutes, and overall catheter-related complications were seen in 11% of patients. Use of laparoscopic techniques for HA catheter insertion is feasible, and appears to be associated with an acceptable complication rate in specialized centers. More recently, robotic-assisted procedures have also been documented.
Early HAI chemotherapy drug delivery systems included HA port systems and percutaneous catheters connected to external pumps. These devices were cumbersome, inconvenient, and associated with significant complications. Such device-related limitations contributed considerably to the slow evolution of HAI chemotherapy. In 1979, the first totally implantable pump system for continuous long-term drug delivery was introduced for clinical use. Subsequent evaluation of the pumps for HAI chemotherapy revealed the feasibility and safety of insertion in addition to acceptable device-related morbidity. In a series of more than 180 patients, Heinrich and colleagues compared totally implantable pumps with HA port systems for HAI chemotherapy. Complications that could not be salvaged and required cessation of treatment occurred in 47% of patients in the port group compared with 30% in the pump group. Complication-free survival was 12.2 months in the pump group compared with 7.3 months in the port group ( P = .002). A second study found that patients with implantable pumps had a 3-fold decrease in the number of treatment interruptions when compared with those with ports ( P = .003). Furthermore, a recent systematic review of more than 3000 patients reported complication rates of more than 30% for port systems, compared with 16% with implantable pumps. In this review, the number studies reporting on chemotherapy cycles delivered were few; however, among these, the median number of cycles delivered in the pump group was 12 versus 8 in the port group, suggesting increased durability and reliability of implanted pumps over HA port systems for HAI chemotherapy.
For completeness, it must be noted that liver-directed chemotherapy via the portal vein (PVI) has been described and evaluated extensively. PVI catheters may be placed into a convenient venous tributary (ileocolic, colic, inferior mesenteric, or gastroepiploic) and used for local drug delivery. A meta-analysis of PVI chemotherapy trials completed before 1987 (10 trials of PVI in more than 4000 patients) suggested an absolute survival benefit of 4.7%. However, this finding was not supported by more recent prospective trials of 5-fluorouracil (5-FU)-based PVI chemotherapy, all of which suggest no significant benefit of PVI in terms of recurrence-free survival (RFS) or overall survival (OS). At present, liver-directed chemotherapy via the portal vein seems to provide little, if any, benefit and is rarely used in the clinical setting.
Introduction
The liver is unique in that it receives blood from the systemic arterial circulation and the enterohepatic circulation. Most blood flow to normal hepatocytes is derived from the portal venous system; alternatively, liver tumors obtain their nutrient blood supply almost exclusively from the hepatic artery (HA). For decades, attempts to take advantage of this dual blood supply to selectively deliver regional therapy to tumors isolated to the liver have been made. In the early 1960s, initial trials of continuous hepatic artery infusion (HAI) chemotherapy for the treatment of liver cancers were reported by Sullivan and colleagues. Though small and inclusive of multiple tumor types, these seminal investigations indicated that HAI chemotherapy was feasible, and that there appeared to be a quantifiable tumor response. Unfortunately, general applicability was limited owing to a lack of standardized chemotherapeutic regimens and tumor response measurements. Furthermore, clinical implementation was hampered by unacceptably high complication rates related to catheter insertion, prolonged infusions, and cumbersome external infusion pumps. Despite the limitations of these early investigations, 3 essential components for safe and effective implementation of HAI chemotherapy were identified: (1) insertion of a durable arterial catheter that allows prolonged use; (2) identification of appropriate drugs; and (3) a delivery system that allows protracted, reliable, and convenient drug administration.
The primary goal of HAI chemotherapy is to selectively deliver high concentrations of drugs to cancer cells while limiting drug exposure to normal hepatocytes. To achieve this, drugs used for HAI chemotherapy must have quantifiable antitumor activity and an optimal pharmacokinetic profile characterized by: (1) high first-pass hepatic extraction; (2) short plasma half-life; and (3) first-order kinetics with steep dose-response curves. To date, several suitable agents with activity against primary and metastatic liver cancers have been evaluated in the setting of HAI chemotherapy. Pharmacokinetic profiles of these commonly used drugs are provided in Table 1 . Relative to other agents, delivery of floxuridine (FUDR) via the HA is most favorable. HAI chemotherapy with FUDR results in 100- to 400-fold greater exposure within the liver and a 15-fold higher drug concentration within tumor cells in comparison with normal hepatocytes. Up to 92% is extracted by the liver on first pass, limiting systemic exposure, and it is rapidly eliminated from the body.
Drug | Hepatic Extraction (%) | Plasma Half-Life (min) | Fold Increase Exposure with HA Infusion | Retained Systemic Exposure Relative to IV Administration (%) |
---|---|---|---|---|
5-Fluorouracil (5-FU) | 22–45 | 10 | 5–10 | 60–70 |
Floxuridine (FUDR) | 69–92 | <10 | 100–400 | <5 |
Mitomycin C (MMC) | 22 | ≤10 | 6–8 | 80–90 |
Cisplatin (CDDP) | — | 20–30 | 4–7 | 100 |
Doxorubicin | 45–50 | 60 | 2 | 40–50 |
Successful HAI chemotherapy is contingent on both the use of an effective drug and the ability to place a durable HA catheter, allowing safe, long-term drug delivery. At present HA catheters are primarily inserted via open laparotomy. In efforts to combat the morbidity of a major abdominal operation, percutaneous techniques have also been used. A recent study by Arru and colleagues compared transaxillary percutaneous (PCT) HA catheter insertion with insertion via laparotomy (LPT). Length of hospitalization and analgesia requirement was greater in the LPT. However, catheter-related complications resulting in delay or cessation of treatment were seen in 43% of the PCT group, compared with 7% of the LPT group ( P = .005). This finding translated into significantly fewer HAI chemotherapy cycles in the PCT group than in the LPT group (4.3 vs 6.5, P = .038). It seems that despite the initial morbidity of a major abdominal procedure, LPT-placed catheters are more durable and allow for more consistent long-term drug delivery. More recently, minimally invasive techniques for HA catheter insertion have been described. In a single-center review of 27 patients undergoing laparoscopic placement of HA catheters, correct catheter placement was observed in 100% of cases, average operative time was 45 to 55 minutes, and overall catheter-related complications were seen in 11% of patients. Use of laparoscopic techniques for HA catheter insertion is feasible, and appears to be associated with an acceptable complication rate in specialized centers. More recently, robotic-assisted procedures have also been documented.
Early HAI chemotherapy drug delivery systems included HA port systems and percutaneous catheters connected to external pumps. These devices were cumbersome, inconvenient, and associated with significant complications. Such device-related limitations contributed considerably to the slow evolution of HAI chemotherapy. In 1979, the first totally implantable pump system for continuous long-term drug delivery was introduced for clinical use. Subsequent evaluation of the pumps for HAI chemotherapy revealed the feasibility and safety of insertion in addition to acceptable device-related morbidity. In a series of more than 180 patients, Heinrich and colleagues compared totally implantable pumps with HA port systems for HAI chemotherapy. Complications that could not be salvaged and required cessation of treatment occurred in 47% of patients in the port group compared with 30% in the pump group. Complication-free survival was 12.2 months in the pump group compared with 7.3 months in the port group ( P = .002). A second study found that patients with implantable pumps had a 3-fold decrease in the number of treatment interruptions when compared with those with ports ( P = .003). Furthermore, a recent systematic review of more than 3000 patients reported complication rates of more than 30% for port systems, compared with 16% with implantable pumps. In this review, the number studies reporting on chemotherapy cycles delivered were few; however, among these, the median number of cycles delivered in the pump group was 12 versus 8 in the port group, suggesting increased durability and reliability of implanted pumps over HA port systems for HAI chemotherapy.
For completeness, it must be noted that liver-directed chemotherapy via the portal vein (PVI) has been described and evaluated extensively. PVI catheters may be placed into a convenient venous tributary (ileocolic, colic, inferior mesenteric, or gastroepiploic) and used for local drug delivery. A meta-analysis of PVI chemotherapy trials completed before 1987 (10 trials of PVI in more than 4000 patients) suggested an absolute survival benefit of 4.7%. However, this finding was not supported by more recent prospective trials of 5-fluorouracil (5-FU)-based PVI chemotherapy, all of which suggest no significant benefit of PVI in terms of recurrence-free survival (RFS) or overall survival (OS). At present, liver-directed chemotherapy via the portal vein seems to provide little, if any, benefit and is rarely used in the clinical setting.
Technical aspects
The Pump
Totally implantable infusion pumps are advantageous in that they allow for long-term, reliable, and continuous drug delivery in the outpatient setting. Several models have been produced. Some rely on a chemical power source while others are battery powered. One of the most commonly used continuous pumps is a titanium disk approximately 7 cm in diameter with volume capacitance varying from 20 to 50 mL ( Fig. 1 ). It consists of 2 chambers separated by a welded bellows. The inner infusate chamber contains the drug to be administered while the outer charging fluid chamber contains the volatile liquid/vapor mixture used as the chemical power source. The pump is designed such that the process of filling the infusate chamber “recharges” the chemical power source, and the only energy source required for continuous function and flow is that provided by periodic refill injections.
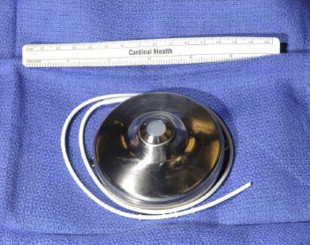
The Patient
All patients being considered for HAI chemotherapy require an extensive search for radiographic, endoscopic, and/or clinical evidence of extrahepatic disease (EHD). Table 2 outlines patient and tumor characteristics that are considered relative contraindications to HAI chemotherapy. In general, patients with no obvious EHD, good performance status, and preserved liver function may be considered for HAI chemotherapy. In those deemed suitable, evaluation of the celiac and superior mesenteric arteries is requisite to delineate HA anatomy. Direct angiography has long been the gold standard for evaluation of hepatic arterial anatomy. However, recent development of faster, better quality computed tomography (CT) scanners has significantly improved noninvasive arterial imagining. CT angiography, performed using the latest technology, allows rapid imagine acquisition and 3-dimensional volume rendering such that detection of aberrant/replaced HA anatomy is conducted similarly to traditional angiography techniques and the morbidity of an arterial puncture is avoided. Consequently, CT angiography is the preferred method for initial surgical evaluation of HA anatomy at most centers.
Patient Factors | Tumor/Anatomic Factors |
---|---|
Poor performance status (Karnofsky <60%) | Extrahepatic disease |
Liver insufficiency (total bilirubin ≥1.5 mg/dL) | Extensive hepatic metastasis (>70% hepatic replacement) |
Portal vein thrombosis |
The Procedure
Careful review of the preoperative hepatic arterial anatomy is essential for successful insertion of implantable pumps. The technical goals of pump placement are outlined in Box 1 . Laparotomy is typically performed via a midline incision unless a concomitant liver resection necessitates a subcostal or hockey-stick incision. On entering the abdomen a thorough search for EHD is completed. If the pump is being placed in the setting of unresectable disease, upfront staging laparoscopy is valuable to rule out EHD before committing patients to open exploration. Once EHD is ruled out, a cholecystectomy is performed to avoid the development of chemical cholecystitis. In patients with normal hepatic anatomy ( Fig. 2 ) the common hepatic artery (CHA) and gastroduodenal artery (GDA) are palpable within the porta hepatis. Once the CHA is localized, dissection is initiated 1 cm proximal to the takeoff of the GDA. The distal CHA, GDA, and proper hepatic artery (PHA) are mobilized circumferentially, and the right gastric artery is ligated. All collateral branches arising from the dissected CHA, GDA, and PHA are ligated to prevent extrahepatic perfusion. The pump pocket is then created at a convenient location, typically in the left abdomen. An 8-cm transverse incision is created in a location that prevents the pump from touching the costal margin and anterior superior iliac spine. The pocket is created superficial to the abdominal wall fascia so that the pump can be easily filled with a needle placed through the abdominal wall. Only when the pocket is complete and the GDA is ready for cannulation should the pump be brought to the surgical field. The pump is filled with heparinized saline and the catheter flushed, an aperture is created in fascia at the center of the pocket, and the catheter is passed into the abdominal cavity. The pump, with the catheter positioned behind it, is then secured in the pocket with stay sutures ( Fig. 3 ). Proximal and distal vascular control of the GDA is achieved through ligation of the distal GDA with nonabsorbable suture and placement of vascular bulldogs/clamps on the CHA and PHA. Alternatively isolated GDA control can be obtained by placing the vascular clamp on the GDA at its takeoff from the CHA. This latter approach facilitates proper catheter placement in that inadvertent advancement into the CHA is prevented. After appropriate vascular control is confirmed, an arteriotomy is made in the GDA and the catheter advanced such that the tip lies at the junction of the CHA and GDA ( Fig. 4 ). Placement of the catheter into the CHA may result in turbulent flow and thrombosis, whereas a catheter placed distally in the GDA leaves a segment of this vessel directly exposed to cytotoxic drugs with minimal flow. This malpositioning can result in sclerosis, thrombosis, or pseudoaneurysm. The catheter is then secured in place with 2 to 3 nonabsorbable ties, as outlined in Fig. 4 B.
Technical goals:
Catheter placement allowing uniform bilobar hepatic perfusion
Ligation of all collateral branches distal to the catheter and proximal to the liver to avoid extrahepatic perfusion
Strategic catheter placement with tip at the gastroduodenal artery/common hepatic artery junction to avoid thrombosis
Identification and ligation of any accessory/replaced hepatic arteries
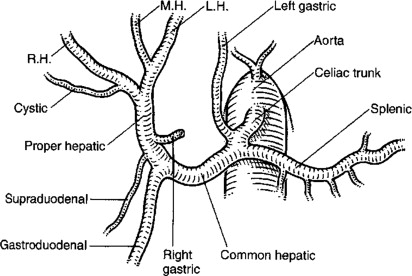
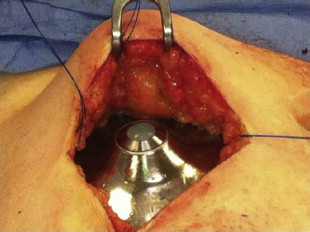
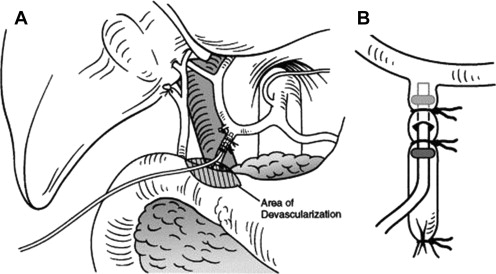
Once the catheter is secured in the GDA, catheter placement and hepatic perfusion are assessed. Methylene blue (using visible light) or fluorescein (using Wood lamp and black light) is injected via the pump and the liver is assessed for uniform dye distribution. Evaluation for evidence of extravasation of dye or inadvertent extrahepatic perfusion is also completed ( Fig. 5 ). In the event of abnormal liver perfusion and/or extrahepatic perfusion, the angiogram is rereviewed to identify potential accessory hepatic arteries or collateral vessels. When found, the vessel(s) should be localized and ligated. Following satisfactory completion of the perfusion test the catheter is flushed, the wounds are closed, and the procedure completed.
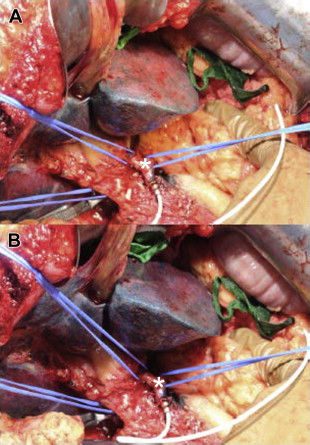
Management of variant hepatic arterial anatomy
Autopsy series suggest that normal HA anatomy, as depicted in Fig. 2 , is present in only 50% to 60% of humans. In his original autopsy series, Michels defined 10 different categories of anomalous hepatic circulation. In essence, these can be divided into variant right or variant left anatomy, and further subcategorized as accessory (in addition to a normal branch) or replaced (in place of a normal branch). One review of more than 1900 patients found that a replaced or accessory right hepatic artery (RHA) off the superior mesenteric artery is the most common variant (14.9%). The second most frequent anomaly is an accessory or replaced left hepatic artery (LHA) originating from the left gastric artery (11.3%).
The presence of aberrant HA anatomy does not usually preclude placement of an HAI pump. Previously dual-lumen pumps were used, whereby both the GDA and the aberrant vessel could be cannulated to achieve uniform drug delivery to the whole liver. These pumps, however, are no longer produced. In general, the optimal means of managing aberrant HA anatomy is to ligate the anomalous vessel and place the pump catheter in the GDA ( Fig. 6 A, B). The safety of this ligation was described in a study of liver perfusion in patients with ligated hepatic lobar arteries that demonstrated uniform bilobar hepatic perfusion in 100% of patients on postoperative day 5. This method, for the most part, also avoids cannulation of a vessel other than the GDA, which in a review of 544 patients undergoing HAI pump insertion was associated with increased risk of pump related complications and failure. Although relatively unusual, variation in the origin of the GDA may be encountered, and includes trifurcation of the LHA/RHA/GDA off the CHA. As depicted in Fig. 6 C, this may be managed in a similar fashion to accessory of replaced LHA/RHA, with ligation of the smaller lobar vessel and catheter insertion into the GDA. On rare occasion the nonaberrant vessel is very small and requires the use of specialized microcatheters for cannulation. In patients with a GDA that cannot be used for a catheter or in patients with no GDA and completely replaced hepatic arteries, advanced techniques using autologous vein or synthetic grafts to construct a conduit for the pump catheter have been described.
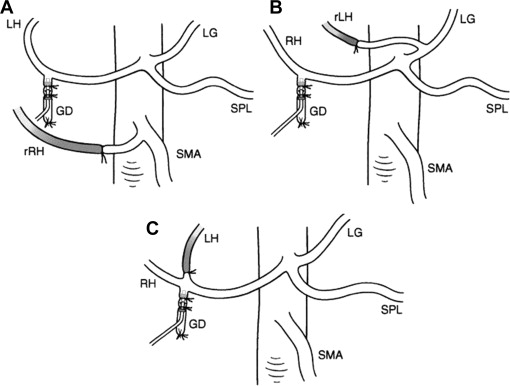
Postoperative management and treatment surveillance
Before initiating HAI chemotherapy, a radionuclide pump-flow study is completed to assess hepatic perfusion. Radiolabeled sulfur-colloid (SC) is injected intravenously, followed by technetium-labeled macroaggregated albumin (MAA) injected via the HAI pump. The perfusion scans are then overlaid and compared to determine uniformity of hepatic perfusion and assess for evidence of extrahepatic perfusion. In the setting of a normal MAA scan, HAI chemotherapy may be initiated, typically 1 to 4 weeks following pump placement. Patients undergoing HAI chemotherapy require fastidious clinical, laboratory, and radiographic follow-up to monitor for treatment related complications and assess disease status. In general, follow-up consists of biochemical laboratory screening (aspartate aminotransferase [AST], alanine aminotransferase, alkaline phosphatase [ALP], total bilirubin) every 2 weeks, and formal clinical examination and toxicity assessment every 4 weeks. Routine radiographic disease surveillance with CT scans of the chest, abdomen, and pelvis may be obtained on a 3- to 4-month basis.
Complications
Early observations of HAI chemotherapy in patients with primary and metastatic liver cancers suggested significant tumor response rates (RR). However, major concerns surrounding high rates of complications limited the use and widespread acceptance of HAI chemotherapy. Depending on the study, complication rates were reported to range anywhere from 30% to 79%. These high rates in part reflect that unlike systemic cancer therapies, HAI chemotherapy is subject to both chemotherapy-related toxicities and potential technical complications related to surgery, the HA infusion catheter, and the implanted pump.
Technical Complications
Over the past 40 years multiple studies have sought to evaluate the incidence of, risk factors for, and clinical impact of technical complications of HAI chemotherapy. The most comprehensive review of these complications, published by Allen and colleagues in 2005, included 544 patients undergoing pump placement over a 15-year period. In this study, the overall pump-related complication rate was 22%. Complications related to the HA system (thrombosis, incomplete perfusion, extrahepatic perfusion, hemorrhage) were most common (51%). Table 3 summarizes complication and salvage rates stratified by timing of complication. The overall pump salvage rate for all complications was 45%. Stratification of complications into those occurring early (<30 days) versus late (>30 days) revealed late complications to be more common and less likely to be salvaged compared with early complications (30% vs 70% respectively; P <.001). In total, 12% of patients had a complication that resulted in pump failure and cessation of therapy. Independent risk factors for pump-related complications in this study were non-GDA cannulation and surgeon experience.
Type of Complication | n | Early (<30 d) | Late (>30 d) | ||
---|---|---|---|---|---|
n | % Salvaged | n | % Salvaged | ||
Pump malfunction | 6 | 6 | 100 | — | — |
Infection | 14 | 4 | 50 | 10 | 40 |
Hematoma | 1 | 1 | 100 | — | — |
Pump migration | 4 | 1 | 100 | 3 | 33 |
Catheter | |||||
Occlusion | 11 | — | — | 11 | 36 |
Dislodgment | 18 | — | — | 18 | 11 |
Erosion | 4 | — | — | 4 | 0 |
Arterial | |||||
Hemorrhage | 1 | 1 | 100 | — | — |
Thrombosis | 33 | 13 | 31 | 20 | 30 |
Extrahepatic perfusion | 16 | 9 | 100 | 7 | 57 |
Incomplete perfusion | 12 | 9 | 78 | 3 | 67 |
Overall | 120 | 44 | 70 | 76 | 30 |
Arterial Thrombosis
Acute intraoperative thrombosis and intimal dissection of the GDA or CHA have been reported during pump insertion. A systematic review of more than 17 studies of implantable pumps revealed a thrombosis rate of 6.6%. Thrombosis can occur early (<30 days) or late (>30 days) following pump insertion; the latter is more common and related to chronic exposure to cytotoxic drugs, sclerosis, and subsequent thromboses. Comparatively, early thrombosis is usually related to catheter malposition and flow disturbance. Regardless of timing, the use of thrombolytics and/or anticoagulants results in salvage rates of approximately 30%.
Extrahepatic/Incomplete Perfusion
Incomplete hepatic perfusion (IHP) occurs in 2% of cases, and typically results from failure to ligate an unrecognized aberrant HA or from failure of collaterals to develop following ligation of a lobar artery. Although IHP presents no danger to the patient or to liver function, the concern is that the oncologic effectiveness of HAI chemotherapy will be diminished. In general, most patients with IHP in the setting of a ligated lobar artery can be followed; repeat imaging scans in 2 to 4 weeks have been associated with near 100% resolution of perfusion abnormality. Alternatively, IHP secondary to a patent accessory artery may be managed with embolization.
Extrahepatic perfusion (EHP) occurs in 2% to 9% of cases. EHP may be related to catheter malposition or collateral vessels arising distal to the catheter tip, leading to perfusion of the stomach, duodenum, or pancreas. These complications may be detected early with a postoperative MAA scan, or later with chemotherapy infusion. EHP is commonly associated with signs and symptoms such as severe epigastric pain or diarrhea with infusion. These symptoms can be caused by ulcers or pancreatitis. In this setting the infusion is discontinued, the drug is emptied from the pump, and investigations including pump flow studies and endoscopy are completed. Embolization of culprit vessel(s) allows for pump salvage in most cases. Fig. 7 depicts an abnormal MAA scan in which EHP is evident in the area of the porta hepatis, and subsequent embolization and repeat scan reveals complete resolution of EHP. Sofocleous and colleagues evaluated the use of embolization for pump salvage in 473 patients with implantable pumps, 45 (9.5%) of whom had MAA scans suggestive of EHP. Of the patients with EHP, 32 (7%) were found to have concomitant angiographic abnormalities. In 8 of 32 (25%) patients, HA thrombosis or catheter-tip migration precluded embolization. In the remaining 24 patients embolization was performed and was technically successful in 21 (87.5%), and resulted in clinical pump salvage in 79% of patients.
