ABO incompatibility of red blood cells leads to brisk complement-mediated lysis, particularly in the setting of red cell transfusion. The ABO blood group is the most clinically significant blood group because of preformed immunoglobulin M (IgM) and IgG antibodies to ABO blood group antigens (isohemagglutinins) in everyone except group AB individuals. In addition to transfusion, ABO incompatibility can cause hemolysis in hematopoietic and solid organ transplantation, hemolytic disease of the newborn, and intravenous immunoglobulin infusion. It is important to prevent ABO incompatibility when possible and to anticipate complications when ABO incompatibility is unavoidable.
Key points
- •
ABH antigens are the major antigens of the ABO blood group and are expressed on the cell surface in many tissues, including red blood cells.
- •
Most individuals express preformed immunoglobulin M (IgM) and IgG antibodies that bind to A and/or B antigens and cause hemolysis, largely through complement activation.
- •
Clinical situations involving ABO incompatible hemolysis include transfusion, hemolytic disease of the newborn, hematopoietic stem cell transplantation, solid organ transplantation, and intravenous immunoglobulin infusion.
- •
ABO incompatibility cannot always be avoided. An understanding of when and how ABO incompatible hemolysis occurs minimizes the incidence and adverse effects.
History
History provides several examples of the consequences of transfusing incompatible blood. The first report of a transfusion reaction was in the seventeenth century after transfusing humans with cow blood. Centuries later, research in animals revealed that interspecies transfusions were more likely to result in fatal reactions, and transfusions with human blood were attempted instead. Transfusions for severe obstetric hemorrhage were occasionally successful, likely because of small transfusion volumes and serendipitous ABO compatibility.
In 1900, Landsteiner identified the ABO blood groups through agglutination testing. This identification was possible because non-group O individuals make immunoglobulin M (IgM) and IgG antibodies that react with foreign A and/or B molecules expressed on erythrocyte cell membrane proteins. The clinical significance of Landsteiner’s discovery is underscored by his receipt of the Nobel Prize in Physiology or Medicine in 1930. With the knowledge of the ABO blood group, transfusion services were established during World War I and grew through the American Red Cross during World War II.
Major and Minor ABO Incompatibility
Hemolytic reactions can occur either by transfusion of incompatible cells into a person with preformed antibodies (major incompatibility, Fig. 1 ) or by transfusion of antibodies into an incompatible recipient (minor incompatibility, Fig. 2 ).
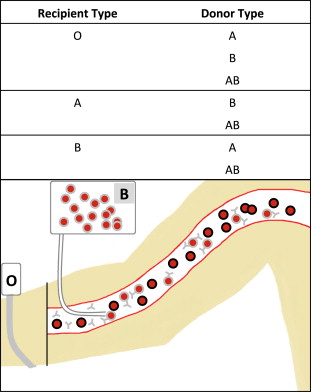
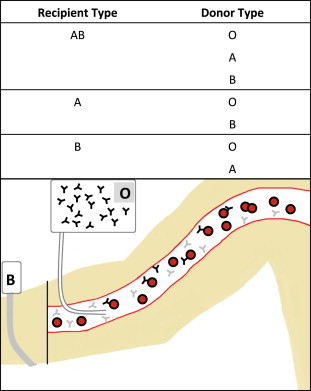
ABO Testing
Blood banks test the ABO type of all blood donors and recipients. Forward typing to determine red cell antigen expression is performed by mixing antibodies against A and B separately with red blood cells. Antigen expression is indicated by erythrocyte agglutination after the addition of a specific antibody ( Fig. 3 ). Reverse typing is performed by observing agglutination of reagent red cells to indicate the presence of antibody in plasma. Testing is performed manually in tubes (see Fig. 3 ) or in automated assays ( Fig. 4 ). Complete ABO typing includes congruent forward and reverse typing.
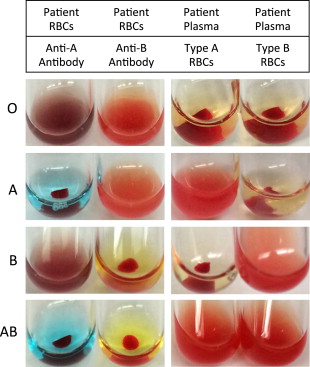
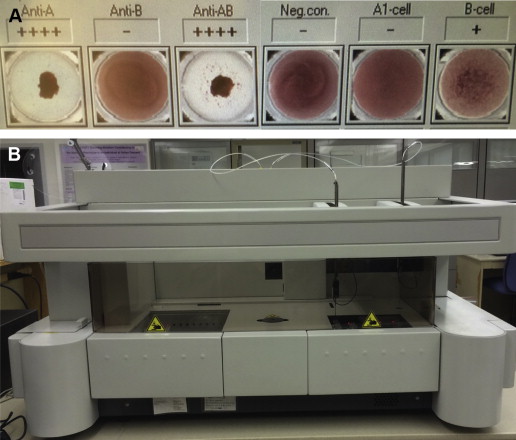
Although these methods are reliable, ABO typing inconsistencies can arise. A and B expression can be lost in the setting of leukemia. An acquired B phenotype occurs in the rare situation in which N-acetylgalactosamine (A substance) is converted into galactose (B substance) because of bacterial enzymatic changes in vivo.
History
History provides several examples of the consequences of transfusing incompatible blood. The first report of a transfusion reaction was in the seventeenth century after transfusing humans with cow blood. Centuries later, research in animals revealed that interspecies transfusions were more likely to result in fatal reactions, and transfusions with human blood were attempted instead. Transfusions for severe obstetric hemorrhage were occasionally successful, likely because of small transfusion volumes and serendipitous ABO compatibility.
In 1900, Landsteiner identified the ABO blood groups through agglutination testing. This identification was possible because non-group O individuals make immunoglobulin M (IgM) and IgG antibodies that react with foreign A and/or B molecules expressed on erythrocyte cell membrane proteins. The clinical significance of Landsteiner’s discovery is underscored by his receipt of the Nobel Prize in Physiology or Medicine in 1930. With the knowledge of the ABO blood group, transfusion services were established during World War I and grew through the American Red Cross during World War II.
Major and Minor ABO Incompatibility
Hemolytic reactions can occur either by transfusion of incompatible cells into a person with preformed antibodies (major incompatibility, Fig. 1 ) or by transfusion of antibodies into an incompatible recipient (minor incompatibility, Fig. 2 ).
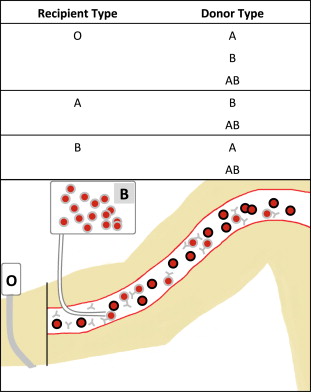
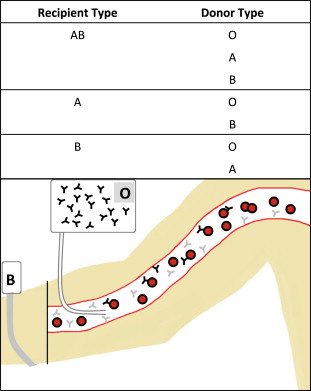
ABO Testing
Blood banks test the ABO type of all blood donors and recipients. Forward typing to determine red cell antigen expression is performed by mixing antibodies against A and B separately with red blood cells. Antigen expression is indicated by erythrocyte agglutination after the addition of a specific antibody ( Fig. 3 ). Reverse typing is performed by observing agglutination of reagent red cells to indicate the presence of antibody in plasma. Testing is performed manually in tubes (see Fig. 3 ) or in automated assays ( Fig. 4 ). Complete ABO typing includes congruent forward and reverse typing.
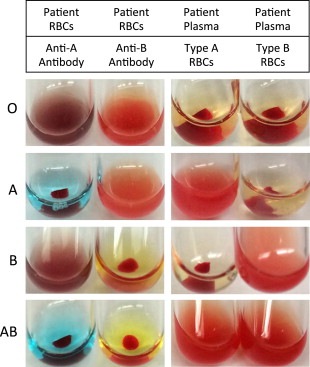
Although these methods are reliable, ABO typing inconsistencies can arise. A and B expression can be lost in the setting of leukemia. An acquired B phenotype occurs in the rare situation in which N-acetylgalactosamine (A substance) is converted into galactose (B substance) because of bacterial enzymatic changes in vivo.
ABO antigen expression and biochemistry
ABO Antigen Structure
Only humans and higher apes express ABH antigens on red blood cells. ABH antigens are also expressed in epithelial cells of the skin and mucosa as well as vascular endothelial cells and solid organ endothelium in most vertebrates, including humans.
The ABO blood group comprises specific polysaccharide motifs on glycoproteins that are expressed at around 1 million antigens per erythrocyte. The A and B groups represent a difference in the selection of a sugar molecule added to the H antigen, whereas the O group results from the absence of A or B sugars. The H antigen is formed by FUT1 enzymes adding fucose to glycoproteins, resulting in a Fuc(α1-2)Gal(β1-3)GlcNAc(β1-R) motif ( Fig. 5 ). Individuals lacking the FUT1 enzyme have the Bombay phenotype and do not express the H antigen. These individuals are incompatible with all ABO blood groups.
A small group of enzymes modifies polysaccharides to produce the ABH antigens, and minor changes in these enzymes cause significant changes in substrate specificity and activity. A and B antigens are produced through modification of the H antigen by glycosyltransferase enzymes (see Fig. 5 ) with substrate specificity for N-acetyl-galactose (A antigen) or galactose (B antigen). Nucleotide changes in the DNA coding sequence determine whether the transferase will produce the A or B antigen. Other changes can either inactivate the protein or prevent translation entirely, resulting in the O phenotype.
Other nucleotide changes affect the activity of glycosyltransferase, creating subgroups of A and B. The A 1 blood type is the most common subtype of A and expresses the A antigen at high levels on erythrocytes. In the A 2 blood type, a single nucleotide deletion leads to the extension of the protein by 21 amino acids resulting in a functional protein with decreased activity. Thus, individuals with the A 2 phenotype have 10-fold lower levels of the A antigen than A 1 individuals.
Epidemiology
The ABO blood group is present in different frequencies in populations around the world. Although type O is most common worldwide, type A is expressed fairly widely in African and European populations, whereas type B is more common in Asian populations ( Fig. 6 ).
Pathophysiology
Initial exposure to an antigen is conventionally required for humoral antibody responses. Repeat exposure is necessary to drive antibody class switching and memory responses. This requirement is true for many blood group antigens, but most individuals express preformed antibodies to foreign ABH antigens without prior transfusion or pregnancy. These antibodies drive complement-mediated intravascular hemolysis during the first transfusion with ABO incompatible blood.
Natural ABO Antibodies
People spontaneously produce antibodies that recognize foreign ABH antigens. These natural antibodies neutralize viruses that express ABH antigens and tend to be nonspecific with low affinity and auto-reactivity. The most abundant ABO antibodies are low-affinity IgM molecules that cross-react with multiple antigens. Antibody titers against A or B antigens are 6 to 10-fold higher in people who do not express those antigens (eg, type O individuals). IgG antibodies are also produced against foreign A or B molecules.
Natural antibody responses to foreign ABH molecules are enhanced by exposure to environmental microbes. Bacteria such as E. coli express a variety of polysaccharides including some homologous to ABH antigens. Total immunoglobulin levels are two-fold to three-fold lower in germ-free animals than normal ones, and anti-B titers are undetectable in germ-free animals. In humans, oral intake or IV injection of microbes that express ABH antigens drives antibody titers up to one hundred-fold higher. Humans naturally produce antibodies that cross-react with diverse pathogens, and coexistence with microbes drives higher titer IgM and IgG antibodies.
Complement Fixation and Red Blood Cell Lysis
When ABO-incompatible red blood cells are transfused, circulating antibodies bind to the ABH antigens and fix complement, resulting in hemolysis (see the article by Varela and Tomlinson elsewhere in this issue for more details). The proportion of ABO-incompatible erythrocytes that are hemolyzed depends on IgM titers. Hemolysis occurs in the vasculature, liver, and spleen within minutes, followed by a second phase in which monocytes phagocytose erythrocytes coated with IgG, C3b, and C3d.
An IgM pentamer is sufficient to fix complement, whereas different IgG subclasses have different efficiencies to fix complement and require at least 2 IgG molecules bound closely together. ABH antigens are expressed at high density so antibody binding drives rapid complement fixation and formation of membrane attack complexes through the classical pathway. C5a generated through complement activation upregulates tissue factor expression on endothelial and immune cells, leading to diffuse intravascular coagulation. The complement cascade alters phospholipids on cell surfaces and creates a surface favorable to thrombosis.
Intravascular hemolysis causes release of noxious intracellular products such as potassium and hemoglobin. Free hemoglobin is bound by haptoglobin and cleared by phagocytes. The system can be overwhelmed and cause hemoglobinemia (and subsequent hemoglobinuria), which depletes nitric oxide and causes endothelial dysfunction. At high levels, hemoglobinemia causes acute renal failure. The release of intracellular contents through hemolysis and activation of inflammatory and coagulation pathways can be life threatening.
Settings of ABO incompatible reactions
Although ABO-incompatible reactions are classically seen in the setting of mismatched red blood cell transfusion, major and minor ABO incompatibility may occur in a variety of different settings ( Box 1 ).
- •
Incompatible red blood cell transfusion
- •
Mismatched platelets or plasma
- •
Hemolytic disease of newborn
- •
Hematopoietic stem cell transplant
- •
Solid organ transplant
- •
Intravenous immunoglobulin
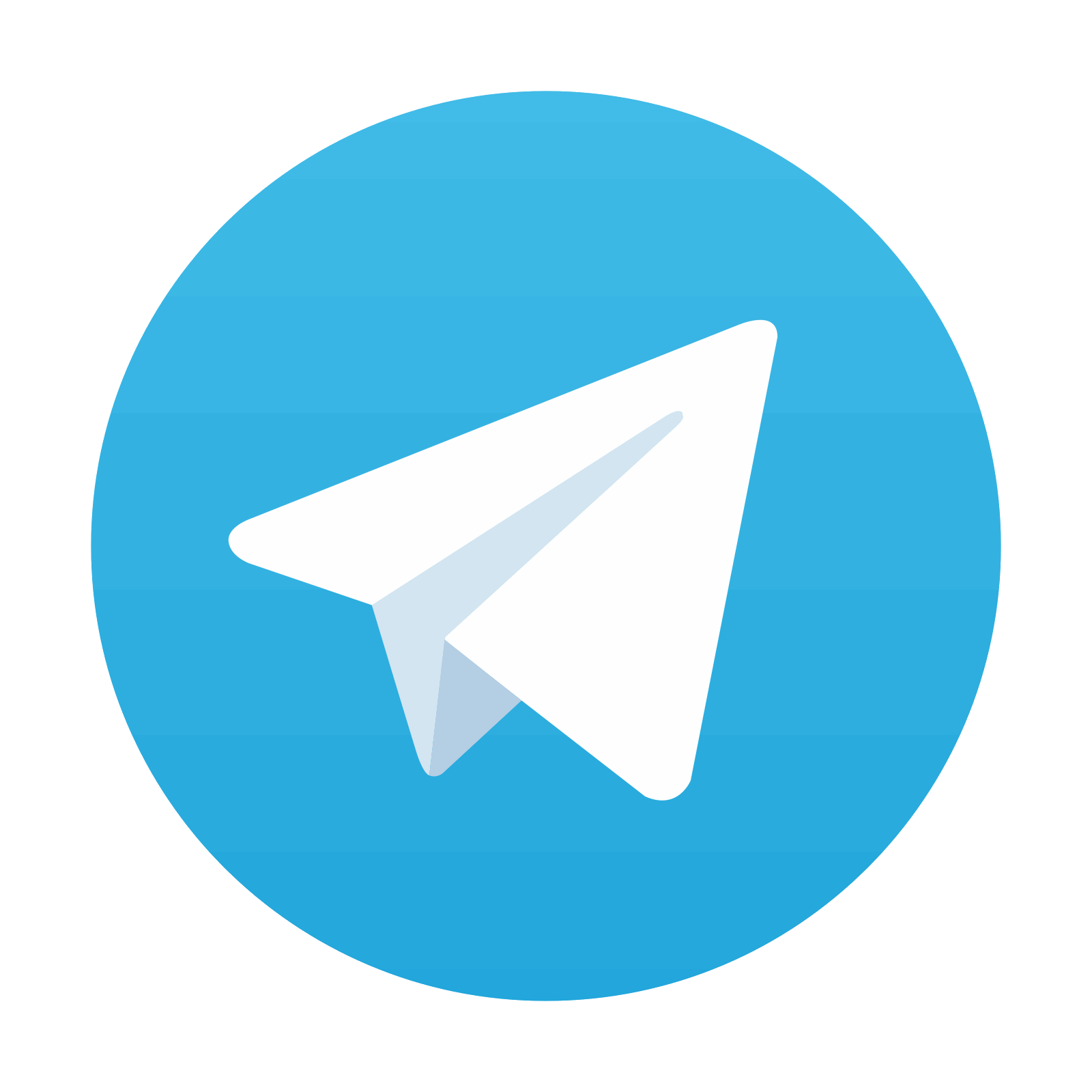
Stay updated, free articles. Join our Telegram channel

Full access? Get Clinical Tree
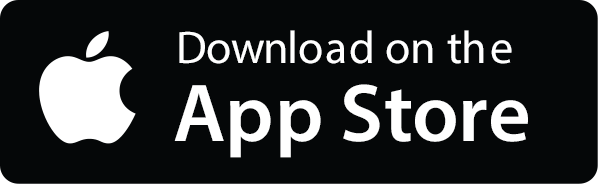
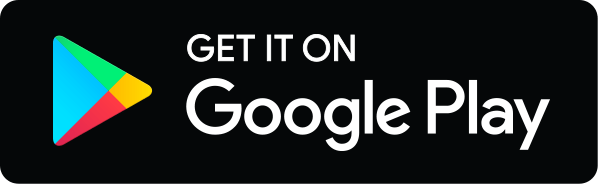
