Bone marrow
Peripheral blood
Cord blood
Limiting factor
HLA match
HLA match
Cell quantity
Minimal HLA match
4/6
9/10
9/10
GVHD risk
Yes
Yes
No
Biggest risk
GVHD
GVHD
Delayed immune recovery
Cells collected from the umbilical cord and placenta after childbirth can also be used as a source of HSCs [15–20]. Advantages of using cord blood are as follows: (1) no risks to donors, (2) immediate availability of cells, and (3) lower risk of GVHD with increased HLA incompatibility [15, 17, 21]. Although HSCs are present at higher concentrations in cord blood, there is an overall smaller quantity that limits the use of cord blood for HSCT. Investigation into methods designed to expand umbilical cord HSCs is an active area of research [22–24].
10.2.2 Autologous and Allogeneic HSCT
Autologous HSCT refers to the infusion of hematopoietic stem cells that were harvested from oneself. Syngeneic HSCT refers to a transplant in which the donor and recipient are genetically the same. This term is used for HSCT between identical twins and for HSCT in animals when the donors and recipients are inbred and genetically identical. Hematologic cancers that are commonly treated with myeloablation and autologous HSCT include multiple myeloma (MM), non-Hodgkin lymphoma (NHL), Hodgkin lymphoma (HL), and acute myeloid leukemia (AML). Treatment of solid tumors such as neuroblastoma, ovarian cancer, and germ-cell tumors may also include autologous HSCT [4].
Allogeneic HSCT refers to donor-derived cells that were obtained from a genetically non-identical individual. Cancers that are often treated with allogeneic transplantation include AML, acute lymphoblastic leukemia (ALL), chronic myeloid leukemia (CLL), NHL, HL, chronic lymphocytic leukemia (CLL), MM, and juvenile chronic myeloid leukemia (CML) [4]. In addition to cancer, myelodysplastic syndromes and myeloproliferative disorders are also treated with allogeneic transplantation. Allogeneic transplantation became feasible during the 1960s with the identification of the major histocompatibility complex (human leukocyte antigen or HLA) and the advent of HLA tissue typing. Matching of donors and recipients is based upon the number of shared HLA antigens. Better HLA antigen matching between the donor and the recipient is associated with higher rates of HSC engraftment and a lower risk for developing life-threatening graft-versus-host disease (GVHD).
10.2.3 Graft-Versus-Host Disease and the Graft Versus Tumor Effect
Mismatches in major histocompatibility proteins as well as polymorphic differences in host proteins (so called “minor” histocompatibility antigens) both contribute to the generation of alloreactivity between the donor and host. GVHD is a complication that occurs when transplanted donor T cells become activated to host alloantigens. GVHD is a three-step process that involves antigen-presenting cell (APC) activation, donor T cell activation upon alloantigen recognition on host APC, and induction of pro-inflammatory cytokines [25]. As a consequence, the host-reactive donor T cells expand and release pro-inflammatory cytokines that support recruitment of other immune effector cells. Together, the activated immune cells can eventually destroy host tissues [26].
GVHD can present as either acute or chronic, and in either case, it is a major barrier to successful cancer-free survival. Acute and chronic GVHD are defined by their timing of occurrence after HSCT. Acute GVHD occurs within the first 100 days post-transplant. During acute GVHD, newly transplanted T cells recognize host alloantigens that are either directly presented by host APC or indirectly presented by donor APC. The major tissues that are targeted for destruction include the skin, liver, and the intestinal tract. Chronic GVHD occurs after 100 days post-transplant, and it is induced when T cells recognize host antigens as foreign after the donor HSCs have engrafted. The pathophysiology of chronic GVHD resembles an autoimmune disease process as opposed to the acute inflammatory process occurring during acute GVHD. Both acute and chronic GVHD can be fatal. Precautions, in the form of immune suppressive therapies, are taken with patients that receive allogeneic HSCT to reduce the incidence and severity of GVHD. It is important to note that minimal levels of GVHD can be beneficial for generating a graft-versus-tumor (GVT) effect that results in the elimination of residual tumor cells (Table 10.2).
Table 10.2
Chemotherapeutic drugs used for myeloablative conditioning
Name | Type | Details | Use |
---|---|---|---|
Busulfan | Sulfonate | Cross-linkage of DNA strands | Leukemia |
Alkylating agent | Prevents DNA replication and transcription | Lymphoma | |
Multiple myeloma | |||
Testicular carcinoma | |||
Breast cancer | |||
Ewing’s sarcoma | |||
Carmustine | Nitrosourea | Cross-linkage of DNA strands | Hodgkin disease |
Alkylating agent | Prevents DNA replication and transcription | Non-Hodgkin lymphoma | |
Lymphoma | |||
Multiple myeloma | |||
Brain cancers | |||
Carboplatin | Heavy metal | Cell cycle nonspecific | Ovarian cancer |
“Alkylating-like” | Causes cross-linkage of DNA strands | Lung cancer | |
Inhibits DNA repair | Head/neck cancers | ||
Prevents DNA synthesis and cell division | |||
Cisplatin | Heavy metal | Cell cycle nonspecific | Sarcomas |
“Alkylating-like” | Causes cross-linkage of DNA strands | Lymphoma | |
Inhibits DNA repair | Ovarian cancer | ||
Prevents DNA synthesis and cell division | Testicular cancer | ||
Cyclophosphamide | Nitrogen mustard | Cell cycle nonspecific | Hodgkin disease |
Alkylating agent | Causes cross-linkage of DNA strands | Non-Hodgkin lymphoma | |
Prevents DNA synthesis and cell division | Leukemia | ||
Multiple myeloma | |||
Neuroblastoma | |||
Retinoblastoma | |||
Solid cancers | |||
Ifosfamide | Nitrogen mustard | Cell cycle nonspecific | Hodgkin disease |
Alkylating agent | Causes cross-linkage of DNA strands | Non-Hodgkin lymphoma | |
Prevents DNA synthesis and cell division | Acute and chronic leukemia | ||
Lung, breast, and ovarian cancer | |||
Melphalan | Nitrogen mustard | Cell cycle nonspecific | Multiple myeloma |
Alkylating agent | Causes cross-linkage of DNA strands | Ovarian cancer | |
Prevents DNA synthesis and cell division | |||
Oxaliplatin | Heavy metal | Cell cycle nonspecific | Colorectal cancer |
“Alkylating-like” | Causes cross-linkage of DNA strands | Gastric cancer | |
Prevents DNA synthesis and cell division | Ovarian cancer | ||
Thiotepa | Organophosphorus | Cross-linkage of DNA strands | Lymphoma |
Alkylating agent | Prevents DNA replication and transcription | Melanoma | |
Solid cancers | |||
Etoposide | Topoisomerase inhibitor | Interferes with action of topoisomerase | Leukemia |
Inhibits DNA synthesis in S and G2 phases | Lymphoma | ||
Cells do not enter mitosis | Kaposi’s sarcoma | ||
Poor immunosuppressive agent | Ewing’s sarcoma | ||
Lung cancer | |||
Testicular cancer | |||
Glioblastoma |
There is an estimated 30 % lower life expectancy in cancer patients that receive an allogeneic transplant as compared to the general cancer population [27–30]. The leading causes for this increase in mortality include recurrent malignancies, infection, secondary cancers, respiratory disease, and chronic GVHD [29]. Autologous HSCT has minimal treatment-related morbidity and mortality and little risk for GVHD; however, autologous HSCT is associated with a higher incidence of tumor relapse as compared to allogeneic HSCT. Occasionally, a syndrome resembling GVHD, often referred to as autologous GVHD, can occur after an autologous HSC transplant. Autologous GVHD appears to occur as a result of immune dysregulation by autoreactive T cells [31].
Despite the devastating consequences of GVHD, low levels of alloreactivity can be beneficial for generating a graft-versus-tumor (GVT) effect [32]. The GVT effect can occur after an allogeneic transplant when donor T cells reactive to host alloantigens present on the tumor cells eliminate the residual cancer. The GVT effect was discovered when physicians attempted to avoid GVHD by extensively depleting donor T cells from the allogeneic HSC graft. Despite a reduction in GVHD incidence and severity, T cell depletion of the graft correlated with a decrease in leukemia-free survival [33]. It has since been demonstrated that T cells are required for an optimal GVT effect, and removal of either CD4 or CD8+ T cells compromises GVT reactivity [34]. GVT effects have been identified in MM, NHL, HL, CLL, and acute leukemia (ALL and AML) [35]. GVHD and GVT both include three interlinked phases: (I) induced pro-inflammatory environment, (II) donor T cell activation and proliferation, and (III) migration of immune effector cells to target tissues [36]. Although the mechanisms of GVHD and GVT both involve the activation of donor T cells against host alloantigens, it appears that these outcomes can occur independent of each other [37, 38]. Approaches which induce a GVT effect while minimizing GVHD focus on reducing pro-inflammatory processes in the recipient while increasing the reactivity of tumor-specific donor T cells [36]. Mechanisms which allow for separation of GVHD from GVT are still not fully understood.
In addition to T cells, natural killer (NK) cells have also been shown to induce GVT effects. NK cells quickly replicate, produce numerous cytokines, kill aberrant cells, and therefore can be useful for boosting an antitumor response [39]. NK cells eliminate tumor cells in a MHC-unrestricted manner either by direct cytotoxicity or by the production of inflammatory cytokines [39]. Clinical trials using NK cells as part of transplant immunotherapy have demonstrated that NK cells have potent antitumor effects [39].
Continued research is needed to advance the field of HSCT for the treatment of malignancy. Specifically, research is needed to (1) optimize the antitumor effect that occurs following an autologous HSC transplant, (2) uncover mechanisms that promote alloreactive effects against tumor cells, and (3) reduce the incidence of severe GVHD following allogeneic transplantation [32, 33].
10.2.4 Myeloablative Effects That Promote the Elimination of Hematologic Malignancies
Depletion of host bone marrow via myeloablative conditioning is a critical prerequisite for the successful engraftment of transplanted HSCs. Bone marrow destruction that occurs from myeloablative conditioning results in the elimination of malignant hematopoietic cells, normal hematopoietic cells including lymphocytes (lymphodepletion), and bone marrow progenitor cells. Myeloablative conditioning is accomplished through the administration of chemotherapy drugs with or without total body irradiation (TBI). Typically, TBI between 8 Gy (800 rad) and 14.4 Gy (1440 rad) is combined with an alkylating chemotherapeutic agent such as cyclophosphamide. Cyclophosphamide is a commonly used chemotherapeutic agent and is often administered for its global lymphodepleting effects as well as for its ability to eliminate malignant cells such as those present in HL, NHL, acute and chronic leukemias, and MM, as well as solid tumors such as neuroblastoma, retinoblastoma, rhabdomyosarcoma, lung cancer, testes cancer, and ovarian cancer. Listed in Table 10.2 are chemotherapeutic drugs that are commonly used for myeloablative conditioning.
Total body irradiation (TBI) in combination with chemotherapeutic drugs has shown benefit over chemotherapy alone for the elimination of hematologic malignancies. Several advantageous effects of TBI include the following: (1) a homogeneous effect regardless of blood supply as the myeloablative effects of TBI can more effectively reach body areas that are underperfused, (2) targeting of specific areas through the use of shields to prevent exposure to body areas where TBI is undesirable, (3) different doses of TBI can result in differential myeloablative and immunosuppressive outcomes, (4) a reduction in the requirement for drug detoxification, (5) TBI is effective against a wide variety of malignancies, and (6) TBI is effective against chemotherapy-resistant malignancies [4]. Originally, myeloablative TBI was given as a single high-dose irradiation. The advantage of this approach was elimination of theoretically all hematologic cancerous cells in the host. However, a major disadvantage included extended cell death beyond the hematopoietic compartment, resulting in debilitating negative side effects. As a result, when TBI is now used for myeloablative conditioning, dosing is typically fractionated. Even though each fraction consists of a lower dose of radiation, the combined myeloablative effect is equivalent to that obtained by a single high dose of radiation. The fractionated radiation is sufficient to eradicate malignant cells and destroy the patient’s HSCs. The time allotted between each TBI treatment allows for some repair of normal tissue damaged by the radiation. Fractioning the TBI has been shown to result in lower toxicity and better survival outcomes when compared to single high-dose treatment. When the toxic side effects of TBI conditioning are of particular concern to certain individuals, such as children and the elderly, radiation-free conditioning methods can be employed instead. For instance, the combination of cyclophosphamide and busulfan can induce a myeloablative outcome similar to that of TBI-containing regimens.
10.2.5 Non-myeloablative Conditioning
Non-myeloablative conditioning results in transient depletion of lymphocytes and other leukocytes without completely ablating the host HSC compartment. Therefore, HSCT is not required following non-myeloablative conditioning, although HSC transplant may still be given in an effort to generate a state of mixed donor-host chimerism. The goal of non-myeloablative conditioning is to eradicate hematologic malignant cells while preserving the HSC compartment and some normal mature hematopoietic cells including immune cells. Non-myeloablative conditioning consists of reduced doses of irradiation and/or chemotherapy. Irradiation of 2 Gy (200 rad) is sufficient to induce damage to quickly replicating cells such as peripheral blood cells and tumor cells. Sublethal doses of irradiation do not eliminate HSCs, allowing for relatively rapid repopulation of the depleted lymphocyte compartment.
The chemotherapeutic drugs used for non-myeloablative conditioning are often similar to those used for myeloablative conditioning (see Table 10.3); however, these drugs are administered at lower doses. Chemotherapeutic drugs used specifically for non-myeloablative conditioning include fludarabine, cladribine, and pentostatin. Non-chemotherapeutic agents, such as alemtuzumab, can also be used for non-myeloablative conditioning. Alemtuzumab is a monoclonal antibody (mAb) that binds to CD52, a protein present on the surface of mature lymphocytes, resulting in their depletion. Since CD52 is not present on HSCs, alemtuzumab will only target mature lymphocytes for depletion allowing the HSCs to remain viable for reconstitution of the immune cell repertoire.
Table 10.3
Drugs used for non-myeloablative conditioning
Name | Type | Details |
---|---|---|
Total lymphoid | Sublethal irradiation | 2 Gy of radiation |
Irradiation | Induces damage to quickly replicating cells | |
Fludarabine | Chemotherapy | Inhibits DNA synthesis |
Purine analog | Interferes with ribonucleotide reductase and DNA polymerase | |
Cladribine | Chemotherapy | Inhibits DNA synthesis through cell’s ability to process DNA Inhibits the enzyme adenosine deaminase |
Purine analog | ||
Pentostatin | Chemotherapy | Inhibits DNA synthesis through cell’s ability to process DNA Inhibits the enzyme adenosine deaminase |
Purine analog | ||
Alemtuzumab | Chemotherapy | Binds CD52 protein on mature lymphocytes |
Purine analog | Results in depletion of lymphocytes only |
Total lymphoid irradiation (TLI) is a type of non-myeloablative conditioning that induces lymphodepletion prior to HSCT or is used alone as a cancer treatment. During TLI, all lymph nodes and the thymus and spleen are irradiated using a linear accelerator, while non-lymphoid tissues are spared. Individuals do not require HSCT after TLI; however, TLI is known to establish allograft tolerance in humans and animals when allogeneic bone marrow cells are transplanted immediately following the TLI [40, 41]. The major advantage of TLI versus non-myeloablative TBI is an observed reduction in organ toxicity and decreased severity of GVHD [41, 42].
Both myeloablative and non-myeloablative conditioning can stimulate antitumor immunity by causing tumor cell death and subsequent release of tumor antigens that can facilitate the activation of antitumor immunity. The tumor antigens released by apoptotic tumor cells can be processed and presented to T cells by APC leading to activation of tumor-reactive cytolytic T cells.
Other mechanisms that may promote antitumor immunity include the elimination of immune suppressive T cells and a decrease in cellular competition for immune stimulatory cytokines [43–46]. For these reasons, both myeloablative and non-myeloablative conditioning regimens have been incorporated into treatment protocols for a variety of hematologic malignancies and solid tumors.
10.3 Lymphodepletion for the Treatment of Solid Tumors
Changes in the hematopoietic compartment after myeloablative and non-myeloablative conditioning have the potential to alter antitumor immunity in several ways. Conditioning eliminates or reduces all hematopoietic cells including immune suppressive myeloid derived suppressor cells (MDSC) and regulatory T cells (Tregs). Reduction in hematopoietic cells, including lymphocytes (T, B, and NK cells) creates “space” in hematopoietic tissues which is necessary for transplanted stem cells to divide and expand. During lymphodepletion, reduction of lymphocytes results in a generalized state of immune suppression. However, decrease in immune suppressive regulatory cells, as well as the reduction in lymphocytes and innate immune cells, allows the remaining T cells to have increased access to cytokines important for their proliferation and activation (IL-7 and IL-15) [47]. The loss of inhibitory regulatory cells, the availability of cytokines, as well as the space provided by lymphodepletion provide an environment that promotes the expansion of cytolytic T cells capable of recognizing tumor antigens. Creating space in the hematopoietic cell compartment is a prerequisite for the promotion of homeostatic proliferation (HP), which allows for the skewed production of tumor-reactive memory T cells. Overall, lymphodepletion favors the maturation of APC necessary for efficient presentation of tumor antigens to tumor reactive T cells, thereby facilitating antitumor immunity [48].
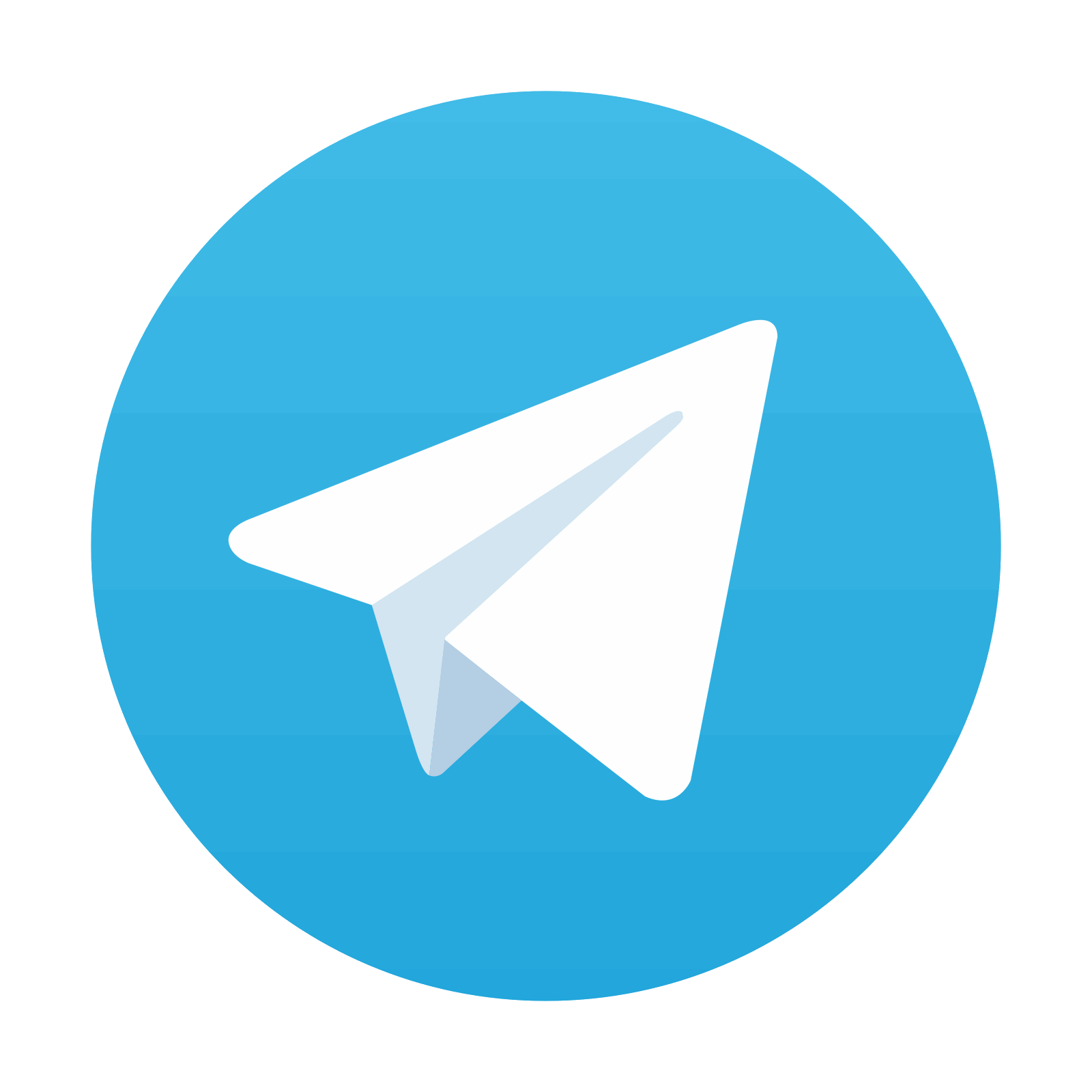
Stay updated, free articles. Join our Telegram channel

Full access? Get Clinical Tree
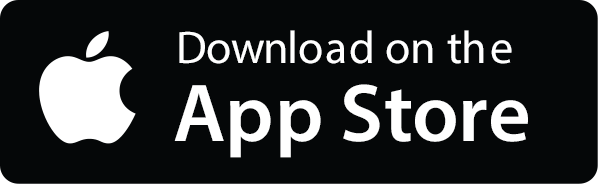
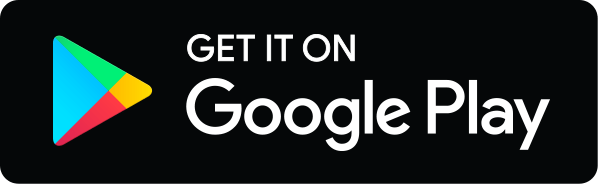