Clinical scenario
Recommendation
Level of evidenceb
Treatment of myelosuppression after chemotherapy (primary prophylaxis)
Consider if risk of neutropenia is ≥20 % or if patient had neutropenia with previous course of chemotherapy
1B
Prevention of febrile neutropenia to avoid delay or dose reduction in subsequent chemotherapy (secondary prophylaxis)
Can be considered in cases where delay or dose reduction is shown to be harmful in treatment outcome
2C
Treatment of neutropenia to prevent infection or with known infection
G-CSF should be given in patients with high-risk neutropenia (i.e., pneumonia, hypotension, multiorgan dysfunction, fungal infection, neutropenia >28 days, bacterial sepsis, age <12 months)
1C
Treatment of myelosuppression with radiation therapy
Can be considered without concomitant chemotherapy and if radiation is not to the mediastinum
1C
Utilization of G-CSF versus GM-CSF for neutropenia
G-CSF should be utilized for neutropenia due to increased efficacy and decreased side effects
1B
Utilization of filgrastim (G-CSF) versus pegfilgrastim
Likely equal efficacy; data are lacking in pediatric patients to routinely recommend pegfilgrastim
2C
Dose of G-CSF to use for neutropenia
Recommended 5 mcg/kg/day; alternative day schedules may be adequate but have not been studied
1A
Route of administration for G-CSF
Subcutaneous is the preferred route
2B
Timing of G-CSF after chemotherapy
Can be started 1–5 days after completion of chemotherapy; data are lacking to distinguish efficacy between starting 1 or 5 days after chemotherapy completion
1B
Timing of G-CSF discontinuation
Stop when post-nadir (i.e., >7–10 days after chemo initiation and ANC >0.5–1.0 × 109/L); stop ≥24 h before next chemotherapy cycle
1B
Utilization of EPO for chemotherapy-induced anemia
Can be considered only if there is contraindication to red blood cell transfusion
1C
Utilization of IL-11 for chemotherapy-induced thrombocytopenia
Significant side effects; lack of evidence to recommend
1A
TPO-receptor antagonists for chemotherapy-induced thrombocytopenia
Data are lacking to recommend
1B
15.2 Granulocyte Colony-Stimulating Factors
Colony-stimulating factors (CSFs) can be non-lineage specific such as IL-3, IL-6, IL-11 and stem cell factor or be restricted to a single lineage such as G-CSF (filgrastim) with neutrophils. Serum levels of CSFs are low unless stimulated by infection or by a reduction in terminally differentiated cells (Lieschke and Burgess 1992). CSF concentration may then be altered by both changes in production and rates of clearance; high neutrophil levels increase CSF clearance (Layton et al. 1989). Normal G-CSF levels are approximately 25 pg/mL with levels ≥1,000 pg/mL seen in response to severe infection (Kawakami et al. 1990). GM-CSF (sargramostim) was the first cytokine approved by the United States Food and Drug Administration (FDA) for stimulation of myelopoiesis in the posttransplant setting and has activity on multiple cell lineages including monocytes and neutrophils. GM-CSF was subsequently followed by G-CSF for both chemotherapy-induced neutropenia and in the posttransplant setting. A longer lasting pegylated form of G-CSF, pegfilgrastim, is FDA approved in adults for stimulation of granulopoiesis after myelosuppressive chemotherapy in nonmyeloid malignancies but is yet to be approved in pediatric patients (Holmes et al. 2002). Limited studies are available on the use of pegfilgrastim in pediatric oncology but it appears effective with a similar side effect profile to G-CSF in solid tumor patients (Wendelin et al. 2005; te Poele et al. 2005; André et al. 2007; Borinstein et al. 2009; Fox et al. 2009; Milano-Bausset et al. 2009; Spunt et al. 2010).
15.2.1 Clinical Usage of Myeloid Growth Factors
Utilization of myeloid CSFs has been reported for the following clinical situations: (1) treatment of myelosuppression after chemotherapy (primary prophylaxis), (2) prevention of febrile neutropenia and delay or dose reduction in subsequent chemotherapy delivery (secondary prophylaxis), (3) treatment of neutropenia to prevent infection, and (4) treatment of infection with neutropenia. Pediatric guidelines are lacking although reviews and meta-analyses have been published (Lehrnbecher and Welte 2002; Sung et al. 2004; Wittman et al. 2006). Although established for adult oncology patients, guidelines by ASCO, ESMO and the European Organisation for Research and Treatment of Cancer (EORTC) can be generalized to pediatric patients as pediatric data alone are inconclusive (Smith et al. 2006; ESMO 2007; Aapro et al. 2011). Generally, the three sets of adult guidelines are similar, recommending CSFs in patients who are: (1) expected to have a ≥20 % risk of chemotherapy-induced neutropenia, (2) have previously suffered chemotherapy-induced febrile neutropenia and delay or dose reduction in chemotherapy delivery which may affect treatment outcome, and (3) as supportive treatment in patients with high-risk febrile neutropenia, variably defined as >7–10 days of neutropenia with uncontrolled primary disease, hypotension, profound neutropenia (i.e., ANC <0.1 × 109/L), sepsis, pneumonia or fungal infection (Smith et al. 2006; ESMO 2007; Aapro et al. 2011).
15.2.1.1 Treatment of Myelosuppression with Chemotherapy Delivery
As stated, adult ASCO, ESMO and EORTC guidelines support the use of CSFs in patients with ≥20 % risk of neutropenia or in patients who have suffered neutropenia in previous courses of chemotherapy. Meta-analyses in pediatric patients by Sung et al. (2004) and Wittman et al. (2006) similarly support this recommendation in pediatric patients. Both found a significant reduction in febrile neutropenia, length of hospital stay and documented infection with prophylactic G-CSF although there was no benefit in infection-related mortality (Sung et al. 2004; Wittman et al. 2006). A meta-analysis by Sasse et al. (2005) in the use of CSFs in pediatric acute lymphoblastic leukemia (ALL) patients similarly found shorter hospital stays and fewer infections with prophylactic CSF use although there was no reduction in duration of neutropenia and no useful information on survival. Sung et al. (2013) recently analyzed G-CSF prophylaxis in pediatric acute myelogenous leukemia (AML) patients treated on the AAML0531 Children’s Oncology Group (COG) study and found a significant reduction in bacterial infection; this contrasts with Lehrnbecher et al. (2007) who reported that although G-CSF significantly reduced time of neutropenia in the AML-BFM 98 trial, there was no decrement in episodes of febrile neutropenia, documented infections, infection-related mortality or 5-year event-free survival. A meta-analysis of AML patients similarly found no benefit of CSF prophylaxis in the prevention of infection (Gurion et al. 2012).
15.2.1.2 Prevention of Febrile Neutropenia, Delay in Chemotherapy Delivery, or Dose Reduction
Adult guidelines recommend consideration for CSFs in patients in which delay in chemotherapy delivery or dose reduction is known to be potentially harmful in treatment outcome (Smith et al. 2006; ESMO 2007; Aapro et al. 2011). Early phase I and II studies in adult patients have shown that reduction in chemotherapy delay can be achieved with G-CSF and GM-CSF (Antman et al. 1988; Bronchud et al. 1989). Pediatric data are unclear although the recently published improved survival in localized Ewing sarcoma patients who received compressed every 2-week therapy with G-CSF support as compared to the standard every 3-week arm implies there are situations in which G-CSF may be beneficial (Womer et al. 2012). Further data are required to make more generalized pediatric recommendations. As a corollary, G-CSF has been analyzed as a method to increase dose intensity since chemotherapeutic effect is directly related to the dose delivered (Bonadonna and Valagussa 1981; Kwak et al. 1990). Multiple pediatric studies have evaluated increased dose intensity with G-CSF support, and although these studies have shown the safety of this method, benefit on outcome has not been delineated (Woods et al. 1993; Kushner et al. 1994; White et al. 1994; Jones et al. 1995; Kushner et al. 1995; Michon et al. 1998; Fernandez et al. 2000; Kushner et al. 2000; Michel et al. 2000; Saarinen-Pihkala et al. 2000; Alonzo et al. 2002). In the meta-analysis by Sasse et al. (2005) in pediatric ALL patients, CSF usage had no effect on chemotherapy delays.
15.2.1.3 Treatment of Febrile Neutropenia
As described, adult consensus guidelines recommend utilization of CSFs in patients with high-risk neutropenia which is variably defined as neutropenia for >7–10 days, profound neutropenia with ANC <0.1 × 109/L, as well as clinical situations including pneumonia, hypotension, sepsis syndrome with multiorgan failure, uncontrolled primary disease and invasive fungal infection (Smith et al. 2006; ESMO 2007; Aapro et al. 2011). In a meta-analysis of randomized controlled trials, Clark et al. (2005) found that the use of CSFs in established febrile neutropenia reduced hospital stay and neutrophil recovery with potential marginal effect on infection-related mortality; no subgroup analysis on patients defined as high-risk could be performed. Limited data exist in pediatric patients: three randomized prospective trials have shown shortened median hospital stays, days of antibiotic use, cost of treatment and a reduction in duration of the febrile neutropenic episode (Riikonen et al. 1994; Mitchell et al. 1997; Ozkaynak et al. 2005). Pediatric consensus guidelines suggest similar parameters for utilization of CSFs in febrile neutropenia as adult guidelines, recommending usage in patients with pneumonia, hypotension, multiorgan dysfunction and fungal infection as well as, potentially, prolonged neutropenia (i.e., >28 days), bacterial sepsis and age <12 months (Schaison et al. 1998; Lehrnbecher and Welte 2002). Although no pediatric study has demonstrated that CSF usage impacts infection-related mortality, reduction in hospital stay and therefore cost are reasonable parameters to support CSF utilization, especially in the high-risk patient.
15.2.1.4 Treatment of Myelosuppression with Radiation Therapy
Adult guidelines recommend avoidance of CSFs during concomitant radiation therapy to the mediastinum due to a noted increased risk of mortality (Smith et al. 2006; ESMO 2007). The ASCO guidelines also warn against the use of CSFs when chemotherapy and radiation therapy are being administered jointly (Smith et al. 2006). No pediatric data are available to make recommendations for these clinical scenarios. The 2002 pediatric guidelines by Lehrnbecher and Welte recommend against G-CSF usage with concomitant chemotherapy and radiation therapy while no mention is made of utilization with radiation therapy alone.
15.2.2 Optimal Administration of Colony-Stimulating Factors
The optimal CSF formulation in pediatric oncology patients as well as the best dosing schedule, route of administration and timing of administration must all be considered when administering CSFs.
15.2.2.1 Comparison of Granulocyte Colony-Stimulating Factor and Granulocyte-Macrophage Colony-Stimulating Factor
GM-CSF is not FDA approved for treating chemotherapy-induced myelosuppression or febrile neutropenia. A meta-analysis of G-CSF and GM-CSF trials for this purpose in adult oncology patients reported a significantly increased rate of fever in patients receiving GM-CSF, a lack of head to head trials between GM-CSF and G-CSF, and GM-CSF being ineffective in reducing febrile neutropenia compared with placebo (Dubois et al. 2004). EORTC adult guidelines recommend filgrastim, pegfilgrastim or lenograstim (not FDA approved) with equipotency; ESMO recommends either filgrastim or pegfilgrastim; and ASCO gives consideration for filgrastim, pegfilgrastim and GM-CSF while cautioning that there is no long-term data with pegfilgrastim and no significant comparative studies between G-CSF and GM-CSF (Smith et al. 2006; ESMO 2007; Aapro et al. 2011). Pediatric data are lacking. Lydaki et al. (1995) randomized a small cohort of pediatric oncology patients to G-CSF or GM-CSF and found a significant delay in neutrophil recovery in those treated with GM-CSF although this had no bearing on antibiotic usage or mean hospital stay.
15.2.2.2 Optimal Dosing
Adult guidelines recommend dosing of 5 mcg/kg of filgrastim, 100 mcg/kg of pegfilgrastim (max 6 mg) and 250 mcg/m2 of sargramostim (Smith et al. 2006; ESMO 2007; Aapro et al. 2011). Few pediatric studies are available. Cairo et al. (2001) compared 5 and 10 mcg/kg of G-CSF starting 24 h after intensive chemotherapy for 123 pediatric patients with relapsed or refractory solid tumors and found no significant difference in time to ANC ≥1.0 × 109/L, incidence of infection, febrile days, incidence of hospitalization or overall survival. A small pediatric study comparing 100 mcg/m2 vs. 250 mcg/m2 of GM-CSF showed that duration of neutropenia was significantly shortened in the 250 mcg/m2 arm with a trend toward decreased duration of febrile neutropenia and no noted difference in side effects (Kubota et al. 1995).
15.2.2.3 Route of Administration
Although package inserts for CSFs consider intravenous and subcutaneous administration to be equipotent, adult data have found that 2–4 times dosing is required to achieve equivalent effect when either G-CSF or GM-CSF is given intravenous as compared to subcutaneous (Eguchi et al. 1990; Kaneko et al. 1991; Stute et al. 1995; Honkoop et al. 1996). Adult guidelines all suggest subcutaneous administration for CSFs (Smith et al. 2006; ESMO 2007; Aapro et al. 2011). No pediatric data are available and no mention of route of administration is made in pediatric guidelines (Schaison et al. 1998; Lehrnbecher and Welte 2002). In the pediatric clinical setting, intravenous G-CSF is often used when the patient is admitted while subcutaneous dosing is given when at home with no dose adjustment.
15.2.2.4 Optimal Timing
Pediatric guidelines suggest initiation of CSFs 1–5 days after completion of chemotherapy while adult guidelines suggest 24–72 h (Schaison et al. 1998; Lehrnbecher and Welte 2002; Smith et al. 2006; ESMO 2007; Aapro et al. 2011). Limited adult and pediatric data have both shown that delay in CSF initiation does not lead to significant difference in mean duration of neutropenia, number of hospital days on parenteral antibiotics or number of febrile neutropenic episodes (Ciernik et al. 1999; Hägglund et al. 1999; Lee et al. 1999; Rahiala et al. 1999; Hofmann et al. 2002). Optimal timing for stoppage of CSFs is also poorly studied. Both pediatric and adult guidelines recommend continuing CSFs through the neutrophil nadir, approximately 7–10 days after chemotherapy (Aapro et al. 2011). The ideal ANC threshold for discontinuation of CSFs is unknown with guidelines recommending ANC >0.5–5 × 109/L as a potential stopping point (Schaison et al. 1998; Lehrnbecher and Welte 2002; Smith et al. 2006). ESMO guidelines suggest a sufficient and stable recovery with no ANC threshold, although they do state the historical practice of continuing until ANC >10 × 109/L is unnecessary (ESMO 2007). The necessity of a daily dosing schedule (i.e., versus every other day or other potential schedules) has not been well studied in the literature and alternative schedules may potentially be equally efficacious at a reduced cost (Djulbegovic et al. 2005). CSFs should be discontinued at least 24 h prior to the initiation of the subsequent chemotherapy cycle due to risk for enhanced myelosuppression by destruction of CSF-stimulated precursors by cell-cycle-specific chemotherapy (Meropol et al. 1992).
15.3 Erythropoietin
Erythropoietin (EPO) is a sialoglycoprotein produced primarily in the cortical region of the kidneys. EPO stimulates the proliferation and terminal differentiation of erythroid precursors in the bone marrow and is specifically stimulated by hypoxic conditions (Krantz 1991; Jelkmann 1992). In addition to effects on proliferation and differentiation, EPO has been shown to modulate apoptosis and increase erythrocyte survival time (Masuda et al. 1999). Additional studies have shown that EPO stimulates the proliferation and migration of endothelial cells in vitro and stimulates the expression of other angiogenic growth factors including vascular endothelial growth factor (VEGF) and placental growth factor (Batra et al. 2003). Recombinant human erythropoietin (rhEPO) was first approved in 1989 for the treatment of anemia associated with chronic kidney disease and subsequently approved for the treatment of chemotherapy-induced anemia in patients with nonmyeloid malignancies. A second EPO-stimulating agent (ESA), darbepoetin alfa, which has a 2–3-fold half-life compared with rhEPO, is also approved by the FDA for adult patients (Zamboni and Stewart 2002). Darbepoetin has undergone a phase I trial in pediatric patients with chemotherapy-induced anemia but is not approved in this population (Blumer et al. 2007).
Multiple adult randomized controlled trials have shown that ESAs increase hemoglobin, reduce red blood cell transfusion requirements and improve quality of life in patients with chemotherapy- or radiation therapy-associated anemia (Bokemeyer 2004; Bohlius et al. 2006). Very limited data exist for pediatric oncology patients and has most recently been summarized by Shankar (2008) (Porter et al. 1996; Csáki et al. 1998; Büyükpamukçu et al 2002; Wagner et al. 2004; Yilmaz et al. 2004; Razzouk et al. 2006; Abdelrazik and Fouda 2007; Çorapcioglu et al. 2008; Durmaz et al. 2011). These studies are generally with small cohorts of patients with a mixture of different pediatric malignancies and utilize variable doses, dose schedules, and routes of administration for rhEPO. The studies all show increased hemoglobin and decreased transfusion requirement as compared to controls. Quality of life data are limited, with only a subset of patients in the largest study showing significant improvement (Razzouk et al. 2006). Data of effect on overall survival between the two groups are not ascertainable due to the small cohorts studied and was only reported in two studies (Wagner et al. 2004; Durmaz et al. 2011).
Two adult trials of rhEPO in breast and head and neck cancer reported in 2003 were concerning for increased mortality rates and increased disease recurrence in the rhEPO treatment arm as compared to controls (Henke et al. 2003; Leyland-Jones 2003). Since that time, multiple meta-analyses have shown that survival may be worsened by utilization of ESAs, possibly secondary to an increased risk of thromboembolic events which may be related to a high hemoglobin goal with rhEPO therapy (Bohlius et al. 2006; Bennett et al. 2008; Bohlius et al. 2009, 2010; Glasby et al. 2010). No pediatric study has reported any case of venous thromboembolism secondary to rhEPO. Concern has also been raised due to the promotion of angiogenic growth factors and expression of antiapoptotic genes by EPO and potential effect on tumor cell growth (Batra et al. 2003; Yasuda et al. 2003). Batra et al. (2003) additionally reported the presence of EPO receptors and expression of EPO on pediatric tumor cells including neuroblastoma, Ewing sarcoma, Wilms tumor, rhabdomyosarcoma, hepatoblastoma, medulloblastoma, ependymoma and astrocytoma. Sartelet et al. (2007) similarly reported increased EPO-R expression on neuroblastoma cell lines, although in vitro they were unable to show increased tumor cell proliferation with exogenous EPO. No study has shown an in vivo effect of ESAs on tumor proliferation and in a recent review Aapro et al. (2012) conclude that current clinical and preclinical data have not shown that ESAs have an effect on disease progression.
Updated 2010 ASH/ASCO guidelines as well as the 2010 ESMO guidelines on the use of ESAs in adult oncology patients recommend a careful weighing of the risks and potential benefits of ESA therapy in patients with hemoglobin <10 g/dL and nonmyeloid malignancies (Rizzo et al. 2010; Schrijvers et al. 2010). Concerns remain in regard to the stimulation of the leukemic clone and therefore ESAs are not recommended in leukemia, especially acute myelogenous leukemia (Takeshita et al. 2000). The combined guidelines recommend that ESAs should be used only in patients currently undergoing chemotherapy and should be used cautiously in patients undergoing therapy with curative intent and in those with risk for thromboembolism (Rizzo et al. 2010; Schrijvers et al. 2010). Additionally, patients should be monitored and treated for other etiologies of anemia such as iron deficiency and also monitored to ensure that hemoglobin does not increase over 12 g/dL (Glaspy and Cavill 1999; Rizzo et al. 2010; Schrijvers et al. 2010). With the paucity of reported data, similar such guidelines are unavailable in the pediatric literature. For pediatric patients, the French National Cancer Institute concluded that: (1) systematic administration of ESAs is not recommended in pediatric cancer patients with anemia, (2) ESAs can be considered on a case-by-case basis in those patients with a contraindication to red blood cell transfusion, and (3) intravenous ESA use is the preferred method of administration (Marec-Berard et al. 2009). From the adult oncology literature it is unclear if there is potency difference between subcutaneous and intravenous rhEPO administration although studies in adult hemodialysis patients have shown that subcutaneous injection is approximately 30 % more effective (Kaufman et al. 1998; Galliford et al. 2005; Vercaigne et al. 2005). In his editorial response to the French guidelines, Feusner (2009) concurs that evidence is lacking to support ESA use in pediatric oncology patients as their benefit in quality of life and cost-effectiveness in this patient population as well as their potential risks in regard to tumor progression, overall survival and thromboembolism are unclear.
15.4 Platelet Growth Factors
Platelet transfusion remains the only method for treatment of clinically significant thrombocytopenia in pediatric oncology patients. Multiple growth factors have in vitro stimulatory effects on platelet production, but only IL-11, stem cell factor and thrombopoietin (TPO) have shown in vivo benefit (Broudy et al. 1995; Kuter et al. 1999; Kaushansky 2005; Bhatia et al. 2007; Zeuner et al. 2007). Only IL-11 is approved for chemotherapy-induced thrombocytopenia and only in adult patients. TPO-receptor antagonists have been approved for the treatment of adult immune thrombocytopenic purpura (ITP) but as yet have not been found effective for the treatment of chemotherapy-induced thrombocytopenia. Multiple additional agents including IL-1, IL-3, IL-6 and GM-CSF have been attempted and found ineffective, to have unacceptable toxicity or lead to antibody development (O’Shaughnessy et al. 1996; Jones et al. 1999; Miller et al. 1999; Demetri 2001; Farese et al. 2001; Vadhan-Raj et al. 2005).
15.4.1 Interleukin-11
Interleukin-11 stimulates megakaryocyte maturation in addition to effects on bone, chondrocytes, neurons, adipocytes as well as gastrointestinal and bronchial epithelium (Musashi et al. 1991; Teramura et al. 1992; Orazi et al. 1996; Du and Williams 1997). Studies in adult solid tumor patients have shown benefit in platelet count and subsequent need for platelet transfusion with no benefit in patients with AML and no benefit in overall survival (Gordon et al. 1996; Tepler et al. 1996; Isaacs et al. 1997; Vredenburgh et al. 1998; Giles et al. 2005; Cripe et al. 2006; Usuki et al. 2007). Only one study has been conducted in pediatric patients. Cairo et al. (2004) reported on 47 patients with solid tumors who received IL-11 after ifosfamide, carboplatin and etoposide chemotherapy. The study compared results to historic controls and found a decreased median time to platelet recovery and need for platelet transfusion although there were significant noted side effects including the development of IL-11 antibodies, papilledema, periosteal bone changes, cardiomegaly, edema and tachycardia (Cairo et al. 2004).
15.4.2 Thrombopoietin Receptor Agonists
TPO is the primary regulator of megakaryopoiesis and has shown both in vitro and in vivo effects with increase in platelet counts in 5–14 days in normal bone marrow (Kaushansky et al. 1994; Kuter et al. 1994; Kaushansky 1998). Although the original recombinant products, recombinant human TPO and recombinant human megakaryocyte growth and development factor, were shown to increase platelet counts, both were discontinued due to development of platelet-neutralizing antibodies (Li et al. 2001; Basser et al. 2002). Prior to discontinuation, both agents had shown benefits in children and adults with solid tumors with a trend toward decreased platelet transfusion and level of thrombocytopenia; benefit on survival was not reported and important side effects included risk for thromboembolism and dose-dependent thrombocytosis (Basser et al. 1997; Fanucchi et al. 1997; Basser et al. 2000; Vadhan-Raj et al. 2000, 2003; Angiolillo et al. 2005; Muskowitz et al. 2007).
Second-generation TPO-receptor antagonists, notably TPO peptide mimetic romiplostim and non-peptide mimetic eltrombopag, have shown dose-dependent increases in platelet count without the development of neutralizing antibodies and both drugs have been FDA approved for the treatment of ITP in adult patients (Bussel et al. 2006; Andemariam et al. 2007; Bussel et al. 2007; Jenkins et al. 2007; Kuter et al. 2008; Bussel et al. 2009). Two small, randomized, placebo-controlled studies in pediatric patients with ITP have similarly shown benefit with no significant short-term side effects (Bussel et al. 2011; Elafy et al. 2011). No study has been published using second-generation TPO-receptor antagonists in chemotherapy-induced thrombocytopenia (Andemariam et al. 2007). Although longer-term treatment in adult ITP patients has been shown safe, further study on the long-term effect of these agents in pediatric patients, especially in regard to the potential for thrombosis, tumor or leukemic cell growth, development of neutralizing antibodies and increased bone marrow reticulin or collagen deposition, is warranted (Kuter 2007; Gernsheimer 2008; Kuter et al. 2013).
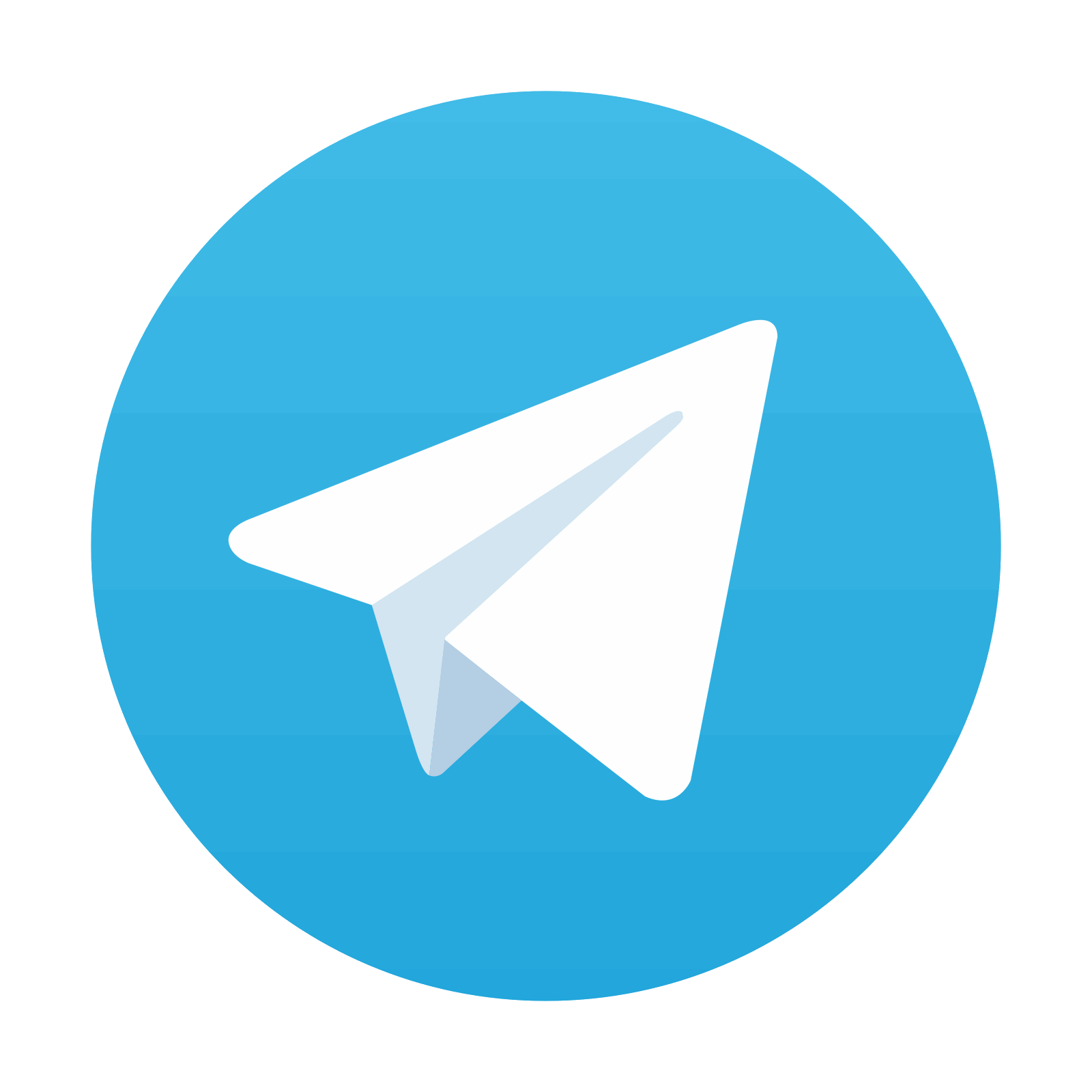
Stay updated, free articles. Join our Telegram channel

Full access? Get Clinical Tree
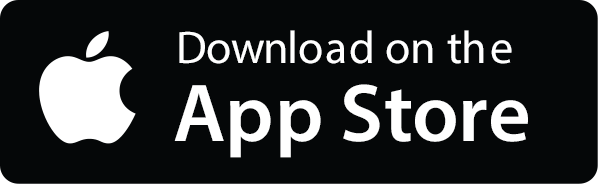
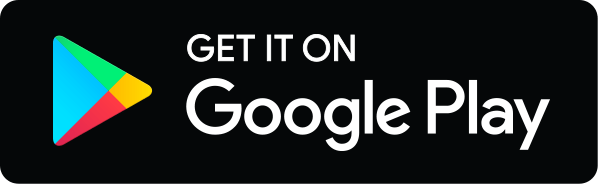