Hematologic Support of the Cancer Patient
Davey B. Daniel
Jeffrey Crawford
No one thinks of how much blood it costs.
–Dante Alighieri
Anemia, neutropenia, and thrombocytopenia are common complications of cancer and its treatment. Physicians caring for oncology patients must recognize the high frequency of myelosuppression, the significant impact on quality of life (QoL), and the risks inherent in severe myelosuppression. Originally armed only with transfusion products, antibiotics, and time, oncologists now have powerful, yet expensive tools to deal with cytopenia. The annual cost of recombinant erythropoietin agents is >1% of overall drug costs in the United States and European Union (1) and approximately 10% of overall direct costs for cancer care (2). Granulocyte growth factors add to the costs and are increasingly used in both hematologic and solid malignancies. On the other hand, the impact of hospitalization for fever and neutropenia are substantial in economic terms and may result in adverse patient outcomes. Therefore, it is critically important that a rationale approach be taken to growth factor use and transfusion support. This requires both an understanding of the literature and clinical judgment. This chapter will review much of this data as well as the current clinical treatment guidelines for anemia, neutropenia, and thrombocytopenia caused by cancer and its treatment.
Chemotherapy can cause both transient and sustained cytopenia. The storage compartment of the marrow contains enough maturing cells to maintain peripheral counts for approximately 8–10 days after stem cell production ceases. Therefore, the effect of cell cycle–specific chemotherapy will be notable by the tenth day after treatment, nadir counts are manifest between days 14 and 18, and recovery generally occurs between days 21 and 28. In contrast, G0 active agents are characterized by delayed nadir counts (4 weeks) and prolonged recovery time (6 weeks) owing to the preferential effect on resting stem cells. Scheduling of chemotherapy is also important as repeated dosing of chemotherapy, especially during early marrow recovery, may result in sustained toxicity and persistent pancytopenia. Likewise, radiation therapy further contributes to myelosuppression, particularly when the marrow is already suppressed from chemotherapy or malignant involvement. Recent trends in medical oncology have focused on providing therapy in more dose intense (autologous stem cell transplantation) and dense fashions (adjuvant breast cancer given every 2 weeks). These regimens along with more myelotoxic agents such as the taxanes have increased the incidence of myelosuppression in commonly treated tumors.
Anemia
Anemia is a frequent finding and a major cause of morbidity in oncology patients. Rates of anemia depend on the tumor type, the myelotoxic treatment given, and the patient’s comorbidities. Anemia is almost universal in patients with hematologic malignancies even prior to treatment. A retrospective study of over 500 patients with lymphoma, lung, breast, colon, or ovarian cancer showed high rates of anemia, from 44 to 95%, depending on tumor type (Table 66.1), but severe anemia was infrequent (<10%). In this study, age, gender, and disease stage were all important predictors of anemia. Although estimates of anemia rates are imprecise, it is obvious that anemia occurs more frequently as medical oncologists adopt more aggressive regimens. Rates vary by regimen but combinations regimens such as CHOP (cyclophosphamide, doxorubicin, vincristine, and prednisone) have rates of grade III anemia (Hb <8.0 g per dL) as high as 70% (3). It is difficult to estimate the actual rates of chemotherapy-induced anemia (CIA) based on newer regimens as many studies only report profound, grade III anemia, and study populations are often healthier and less prone to anemia than the typical patient in the community setting. (See Table 66.2 for explanations of the common toxicity criteria for cytopenias.) Despite the difficulty in pinpointing precise rates, anemia is recognized as a common and treatable side effect of cancer and continues to require treatment. A large retrospective audit of cancer centers in the United Kingdom in the late 1990s found that more than a third of patients required at least one transfusion during the course of their treatment for cancer with high rates in those with lung or ovarian cancer (40%) (4).
The etiology of anemia in patients with cancer is multifactorial, often arising from both the cancer itself and its treatment. Tumor cells can invade the bone marrow or can suppress marrow and erythropoietin production by cytokine production. Blood loss, shortened red cell survival, nutritional deficits, and underlying infection (again causing cytokine-related suppression of the marrow) can be sources of anemia. Less common causes include acute hemorrhage from the malignancy itself, hemolytic states such as disseminated intravascular coagulation associated with promyelocytic leukemia and sepsis, and immune hemolytic anemia from chronic lymphocytic leukemia and lymphomas. Anemia of chronic disease occurs frequently in patients with cancer and is characterized by normocytic and normochromic morphology on peripheral and bone marrow examination, low serum iron and iron binding capacity,
normal or increased marrow iron stores, and blunted erythropoietin levels and response. CIA is common in patients with cancer and the focus of much research and treatment. The continued bombardment of the marrow by myelotoxic regimens can cause significant and prolonged anemia with repeated cycles causing a cumulative injury to production.
normal or increased marrow iron stores, and blunted erythropoietin levels and response. CIA is common in patients with cancer and the focus of much research and treatment. The continued bombardment of the marrow by myelotoxic regimens can cause significant and prolonged anemia with repeated cycles causing a cumulative injury to production.
Table 66.1 Rates of Anemia in a Retrospective Study of Patients Undergoing Chemotherapy. Pre- and Post-Treatment Incidence of Anemia | ||||||||||||||||||||||||||||||||
---|---|---|---|---|---|---|---|---|---|---|---|---|---|---|---|---|---|---|---|---|---|---|---|---|---|---|---|---|---|---|---|---|
|
Symptoms and Signs
Fatigue is an all too frequent symptom of cancer, and anemia is often the only treatable cause of cancer-related fatigue. In a survey of 1171 patients, fatigue was identified as the leading complaint of patients with cancer—greater than pain, nausea, or emesis (6). Although the physicians’ major focus is often on treating pain, patients often note that fatigue is a greater concern. In addition to fatigue, anemia may be associated with other symptoms: shortness of breath, tachycardia, chest pain, dizziness, headache, claudication pain, angina, and even depression. The pattern of symptoms and the individual’s ability to tolerate them is significantly dependent on the rapidity of onset of the anemia, the patient’s baseline functional status, and comorbid conditions. Although patients with anemia may show pallor, orthostasis, and hypoxia, these are usually present in patients with large acute hemorrhages who have not had sufficient time for volume expansion. It is the gradual onset of anemia that is much more characteristic of oncology patients and is more often associated with the insidious symptoms of fatigue, decreased exercise tolerance, and sometimes confusion, particularly in the elderly. Indeed, elderly patients have a lower reserve capacity and may display any of these symptoms even at higher hemoglobin levels. With laboratory testing, the diagnosis of anemia is rarely difficult. The history and examination clarifies how well a patient is tolerating the anemia. Physical examination findings are highly variable. Pallor can be difficult to recognize and is very dependent on pigmentation but mucosal membranes, nail beds, and palmar creases are often areas where pallor is more consistently noted. The decreased blood volume due to anemia may cause widened pulse pressure, a hyperdynamic precordium, and systolic flow murmurs loudest at the apex with radiation along the sternum to the neck. Finally, patients with severe anemia can show signs of high output cardiac failure with peripheral edema and an S3 gallop.
Table 66.2 National Cancer Institute Common Terminology Criteria for Adverse Events | ||||||||||||||||||||||||
---|---|---|---|---|---|---|---|---|---|---|---|---|---|---|---|---|---|---|---|---|---|---|---|---|
|
Thresholds for Treatment
Oxygen delivery is maximized at hematocrits between 30 and 40%, but observational studies have shown no difference in mortality in patients with hematocrits as low as 10%—provided sufficient intravascular volume is maintained. In the intensive care medicine literature, there remains a debate over what transfusion threshold should be used. A multicenter randomized trial of 838 intensive care unit patients showed that a restrictive transfusion strategy, using 7 g per dL of hemoglobin versus 10 g per dL in the control, yielded similar 30-day mortality rates and had statistically significant lower in-hospital mortality and transfusion rates (7). Although the higher threshold did not increase mortality in this acute
setting, we do not know long-term effects including mortality and QoL.
setting, we do not know long-term effects including mortality and QoL.
This intensive care population is also very different from the patients usually seen in oncology. The patient with cancer often has a chronic anemia, and the focus is generally not on short-term mortality but rather on QoL and symptom management. It had been thought that chronically anemic patients fare better than others through compensatory mechanisms of maintenance of plasma volume, increased cardiac output, and shifts in the oxygen–hemoglobin dissociation curve from 2,3-diphosphoglycerate production. However, there is now mounting evidence that oncology patients with chronic anemia may benefit significantly from hemoglobin levels near normal, which are far greater than that we have sought to maintain in oncology patients in the past or currently in other patient populations. The decision to transfuse a patient with blood products must take into consideration the degree of physiologic stress from the anemia, comorbid illness, the potential of recovery without transfusion, and finally, transfusion risks. Transfusion thresholds vary but are often carried out only when hemoglobin levels dropped substantially, sometimes below 8 g per dL. New data arising from the use of erythropoietin agents suggest that QoL may be better maintained at much higher hemoglobin levels (near 12 g per dL), suggesting that many patients may be undertreated. With the availability of recombinant erythropoietin, there is now an alternative to transfusion, allowing for these higher treatment goals.
Role of Erythropoietin
Erythropoietin plays a key role in red blood cell (RBC) production and in the anemia due to cancer. An obligatory growth factor and regulatory molecule, erythropoietin affects RBC production by stimulating the burst forming units–erythroid (BFU-E) and the colony forming units–erythroid (CFU-E). The BFU-E cells are early cells within erythropoiesis, which proliferate rapidly and have a low number of erythropoietin receptors. Therefore, a higher level of erythropoietin is necessary to stimulate entry into the cell cycle for BFU-E cells. The later CFU-E cells have a high number of erythropoietin receptors and require a constitutive level of erythropoietin for continued survival, growth, and production. Erythropoietin therefore shows a keen regulatory mechanism for red cell production. In the adult, approximately 90% of erythropoietin is produced within the peritubular fibroblastoid cells of the inner cortex of the kidney, and the remainder is produced in the liver. Within the peritubular interstitial cells, erythropoietin production is constitutive and inducible with baseline levels produced from each cell and additional cells recruited to increase production for hypoxic states. As arterial PO2 levels decrease and tissue hypoxia increases, erythropoietin levels increase, acting as a key regulatory mechanism. Erythropoietin has been shown to be responsive to hypoxic states, and as seen in the setting of anemia, it increases committed red cell progenitors and the rate of maturation of the reticulocytes. The erythropoietin level is fairly constant for an individual with stable hemoglobin level, does not vary significantly with gender or age, and has no biologically inactive precursor or stores.
Cancer can affect erythropoietin by decreasing the marrow responsiveness to erythropoietin or by decreasing erythropoietin production, as tumor necrosis factor and some nephrotoxic agents can. In both a rat model and in a group of patients with lung cancer, cisplatin was shown to cause depressed erythropoietin levels from the expected compared to controls with similar levels of anemia (8). These patients did not mount the usual increased erythropoietin response levels to anemia after receiving cisplatin but were capable of producing increased levels once cisplatin treatment had been completed and renal function improved. Although this study suggests that some patients have insufficient erythropoietin production, others show that marrow of patients with cancer may be unable to respond to the physiologically increased levels in response to cancer (9).
Epoetin α and β
There has been rapid progress during the last two decades as biotechnology has changed our treatment of anemia. It was only in 1985 that recombinant human erythropoietin (rHuEPO) was purified and cloned. In 1987, it was approved for treatment of anemia related to renal disease and then, in 1990, it was approved for Human Immunodeficiency Virus (HIV)–related anemia. A number of small phase I and II trials in the early 1990s proved efficacy in the treatment of anemia related to cancer or chemotherapy. In a phase I and II trial of 21 patients receiving cisplatin with hemoglobin levels <11 g per dL, patients were randomized to 100 units per kg or 200 units per kg five times per week and the overall response rate was >50%, with a mean rise in hemoglobin of 2.5 g per dL (10). A number of nonrandomized phase II trials confirmed these findings. Suddenly, mild to moderate anemia (hemoglobin of 8–11 g per dL) was now treatable. Recombinant erythropoietin in the form of epoetin α was approved for treatment of CIA in 1993. However, it had not yet been shown to be effective in large trials with a variety of tumor types. Three large community-based, observational studies of epoetin α were completed during the late 1990s. Each prospectively examined the open-label use of erythropoietin in anemic patients with cancer undergoing chemotherapy and evaluated changes in hemoglobin, transfusion requirements, and QoL measurements. All three studies reached similar conclusions regarding the effectiveness of erythropoietin in increasing both hemoglobin levels and QoL scores (Fig. 66.1)).
Demetri et al. (11) examined 2370 patients with nonmyeloid malignancies, the majority (78%) of whom had solid tumors (24% lung, 17% breast, and 13% gynecologic). Roughly one-fourth of these patients received a platinum-based therapy—a drug that causes significant anemia. Each had a hemoglobin
level <11 g per dL, with an overall mean hemoglobin of 9.3 g per dL, and were given 10,000 units of epoetin α three times weekly with increased doses to 20,000 units if unresponsive at week four. Sixty-three percent of patients responded by an increase of at least 1 g per dL after 4 weeks of treatment. After 16 weeks, the mean hemoglobin had risen by 2 g per dL, and 61% of patients had achieved either a 2-g per dL response or a hemoglobin >12 g per dL. The transfusion rate had decreased from 29% the month before treatment to 5% at week 16. These results were consistent with a similar study by Glaspy et al. (12) of 2352 patients and a third study by Gabrilove et al. with 3012 patients (13). The main difference in these trials was the dosing schedule—weight-based dosing three times weekly (Glaspy), flat dosing at 10,000 units three times per week (Demetri), and weekly dosing with 40,000 units per week (Gabrilove). Each had hemoglobin response rates from 53.4–68%, mean hemoglobin rise of 1.8 g per dL to 2.0 g per dL, and significant decreases in transfusion requirements. The use of three times weekly dosing schedule is somewhat inconvenient, but a recent randomized, blinded, placebo-controlled study confirmed that weekly epoetin α as studied in Gabrilove et al. is a reasonable treatment choice for patients with CIA (14) and resulted in a significant difference in mean hemoglobin and RBC transfusions compared to placebo (Fig. 66.2)). A recent observational study of community use of epoetin α showed that >72% of those examined used epoetin α dosed weekly at 40,000 units with more frequent dosing now uncommon in the community setting (15).
level <11 g per dL, with an overall mean hemoglobin of 9.3 g per dL, and were given 10,000 units of epoetin α three times weekly with increased doses to 20,000 units if unresponsive at week four. Sixty-three percent of patients responded by an increase of at least 1 g per dL after 4 weeks of treatment. After 16 weeks, the mean hemoglobin had risen by 2 g per dL, and 61% of patients had achieved either a 2-g per dL response or a hemoglobin >12 g per dL. The transfusion rate had decreased from 29% the month before treatment to 5% at week 16. These results were consistent with a similar study by Glaspy et al. (12) of 2352 patients and a third study by Gabrilove et al. with 3012 patients (13). The main difference in these trials was the dosing schedule—weight-based dosing three times weekly (Glaspy), flat dosing at 10,000 units three times per week (Demetri), and weekly dosing with 40,000 units per week (Gabrilove). Each had hemoglobin response rates from 53.4–68%, mean hemoglobin rise of 1.8 g per dL to 2.0 g per dL, and significant decreases in transfusion requirements. The use of three times weekly dosing schedule is somewhat inconvenient, but a recent randomized, blinded, placebo-controlled study confirmed that weekly epoetin α as studied in Gabrilove et al. is a reasonable treatment choice for patients with CIA (14) and resulted in a significant difference in mean hemoglobin and RBC transfusions compared to placebo (Fig. 66.2)). A recent observational study of community use of epoetin α showed that >72% of those examined used epoetin α dosed weekly at 40,000 units with more frequent dosing now uncommon in the community setting (15).
A number of randomized trials using various dosing regimens have now been performed. A 2005 meta-analysis of 27 randomized trials (3287 patients) examining treatment with epoetin α or epoetin β from 1985 to present showed that patients treated with erythropoietin had lower transfusion rates and increased hematologic responses. Those treated with erythropoietin had transfusion rates 33% lower than untreated patients [RR = 0.67 (0.62, 0.73)] (1). In a sub analysis of 14 trials in this meta-analysis, 48% of patients receiving erythropoietin had a hemoglobin response [RR = 3.60 (3.07, 4.23)].
Quality of Life
Although clinical parameters such as hemoglobin level and transfusion requirements are important, much of the drive to treat CIA lies in the effects on QoL. Each of the community-based trials examined patient self reported QoL parameters; all three used a Linear Analog Scale Assessment (LASA), a 100-mm visual analog scale with questions on energy, activity, and overall QoL. The Demetri and Gabrilove studies also used a 20-item questionnaire that examines well being associated with fatigue and anemia (FACT-An). Each trial confirmed a correlation between increase in hemoglobin levels and improvement in QoL (Fig. 66.1)). In the Demetri trial, the difference between those treated and those untreated was statistically different (p <.001) with those with the greatest increase in hemoglobin showing the most improvement. Those with a <2 g per dL response also had improvement in QoL measurements if the disease was stable or responsive to chemotherapy. As might be expected, patients did not have an improvement in QoL if hemoglobin level did not increase or the QoL effect was blunted if they had disease progression. Each of these large trials was open labeled; so, self reported QoL changes could be suspect due to patient bias. However, a blinded, randomized trial of thrice weekly epoetin α in patients receiving nonplatinum based chemotherapy showed QoL benefits in three primary QoL scales (FACT-General, FACT-An, and CLAS subscales) with trends in two other scales (SF-36 Physical Component Summary and Mental Component Summary). This confirmed in a blinded study the QoL benefits of treating CIA with epoetin α (16). In addition, there are additional studies that support the use of erythropoietin agents in patients who have anemia secondary to cancer, but not resulting from chemotherapy. These included epoetin α, epoetin β, and darbepoetin treatments.
Darbepoetin
Darbepoetin α is an analog of erythropoietin with two additional oligosaccharide chains; it is a larger molecule (38,500 vs. 30,400 d) with a slightly different amino acid structure (five amino acid substitutions). With the increased glycosylation, its half-life is far greater. Intravenously administered darbepoetin’s half-life measures 25.4 hours, and the subcutaneous half-life is even longer at >48 hours (17). Although its affinity for the erythropoietin receptor is somewhat less than rHuEPO’s affinity, this does not seem to impact its effectiveness. In 1999, darbepoetin was approved for patient with renal failure. In July 2002, the U.S. Food and Drug Administration (FDA) approved darbepoetin for treatment of CIA. Five randomized clinical trials have shown that treatment with darbepoetin reduces transfusions requirements and increases hemoglobin levels in patients with CIA, anemia caused by lymphoproliferative disease, and patients with anemia caused by solid tumors (18, 19, 20, 21, 22). Hemoglobin response rates of 40–70% were seen. Darbepoetin therapy has also been shown to improve QoL with FACT-F scores showing significant improvement at 4 weeks and continuing through treatment (20). Two large head to head trials comparing darbepoetin to epoetin α have now been completed and show few differences in clinical response between the two agents (23, 24). Both were industry-sponsored trials that have differences in structure and analysis that make decisions regarding differences in efficacy difficult. Waltzman et al. (23) examined 352 patients randomized to epoetin α or darbepoetin; this study reported higher mean hemoglobin levels (1.2 vs. 0.8), higher rates of hemoglobin response (>2 g per dL) (57 vs. 41%), and shorter median time to 1 g increase in hemoglobin for epoetin α treated patients compared to darbepoetin (35 vs. 48 days). The trial by Glaspy et al. had a noninferiority design with similar dose adjustment rules between the two drugs (24). This showed no statistically significant differences between the two drugs in regard to transfusion requirements and percentage of patients achieving the hemoglobin target. At this point, much of the decision of which erythropoietic agent is driven by patient
convenience and economics which are specific to the patient and clinical setting.
convenience and economics which are specific to the patient and clinical setting.
Table 66.3 Common Dosing Options for Epoetin Alpha and Darbepoetin Alpha | ||||||||||||||||||||||||
---|---|---|---|---|---|---|---|---|---|---|---|---|---|---|---|---|---|---|---|---|---|---|---|---|
|
Recent and ongoing trials continue to examine alternative dosing regimens including the use of so-called front loading regimens. The front loading studies have looked at higher initial doses of epoetin α and darbepoetin followed by either lower or less frequent dosing as maintenance. Pilot studies of epoetin α have shown increased response rates of 86% (Hb increases of 2 g per dL by week 8) (25); this is far superior to those results seen in the standard 40,000 units per week community-based trials. Darbepoetin dosed weekly initially, then on an every 3-week maintenance schedule has also been studied and also shown to have similarly high rates of response, 84–86% (26). However, it is important to note that these dosing strategies have not been studied in a large community setting and that response rates are generally higher in pilot trials than in larger phase III trials or in actual practice.
Erythropoietin and Myelodysplasia
The most common morbidity of myelodysplastic syndrome is anemia, and it is often difficult to manage. Repeated transfusions are often necessary and eventually lead to iron overload. For those with symptomatic cytopenias, the use of growth factors [epoetin α, darbepoetin, and sometimes, granulocyte colony-stimulating factor (G-CSF)] has improved management of anemia in myelodysplastic syndromes (MDS). However, response rates remain low. Other causes of anemia such as concurrent iron or B12 deficiencies or hemolytic anemia should be been ruled out or treated prior to considering the use of recombinant factors. In general, patients with myelodysplastic syndrome lack normal marrow response to erythropoietin and are less responsive to elevations in native erythropoietin often seen in these patients. However, the use of erythropoietin (40,000 units per week or 150 units per kg three times weekly) (27) or darbepoetin (150 μg per wk) (28) has been shown to have responses in up to 45% of patients. Those with lower levels of native serum erythropoietin (<100 mU per mL) often have higher response rates. National Comprehensive Cancer Network (NCCN) guidelines, however, call for consideration of Erythropoietic agents in patients with serum erythropoietin levels <500 mU per mL. Responses may be delayed, so 2–3 months of treatment are often recommended. For patients unresponsive to erythropoietin, the addition of G-CSF can be considered, particularly in those with sideroblastic anemia and relatively low levels of baseline erythropoietin. In patients who fail to respond after 2 to 3 months, it may be time to consider more aggressive therapy such as the DNA methylation inhibitors.
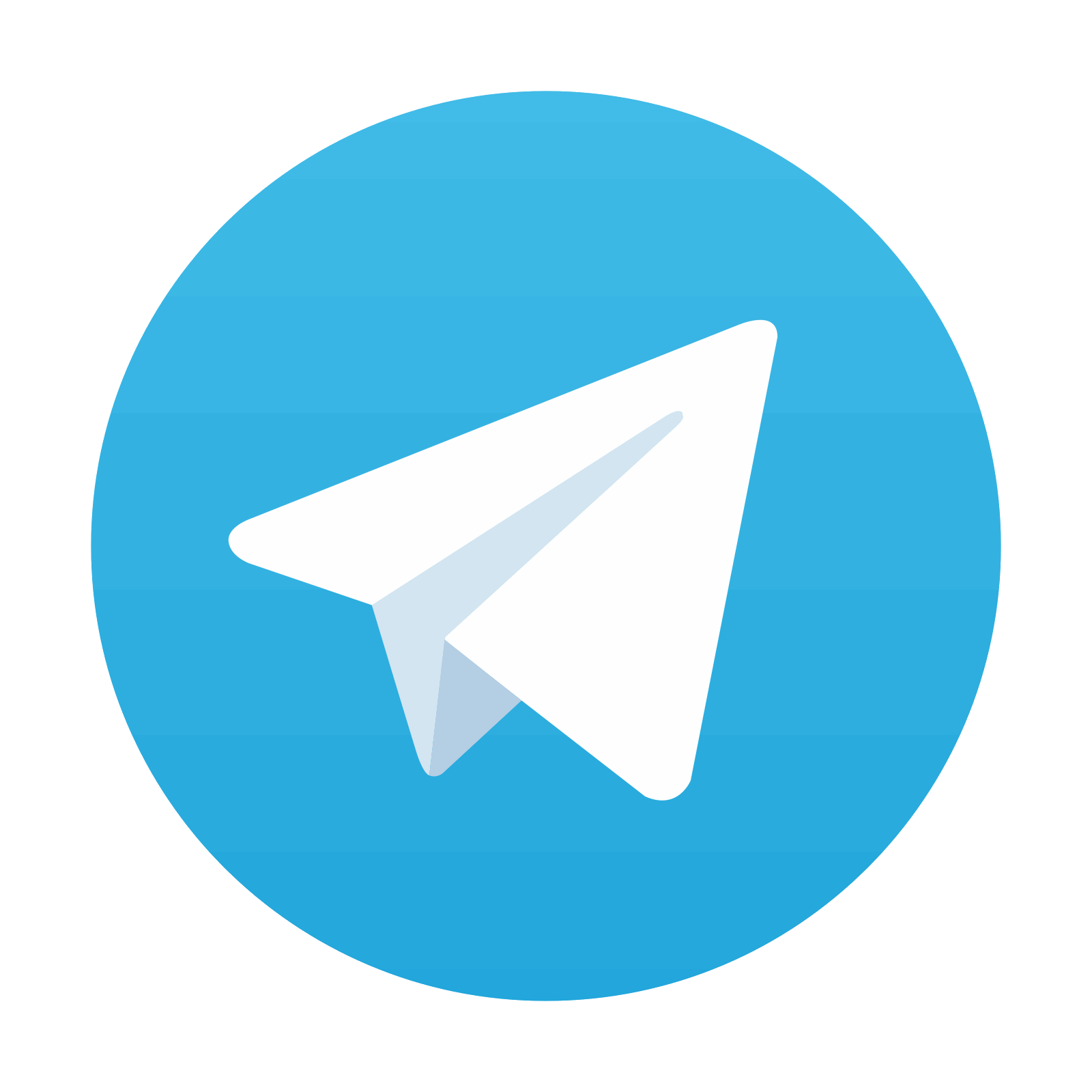
Stay updated, free articles. Join our Telegram channel

Full access? Get Clinical Tree
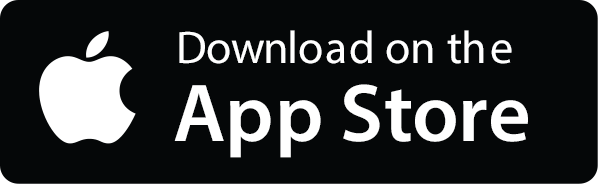
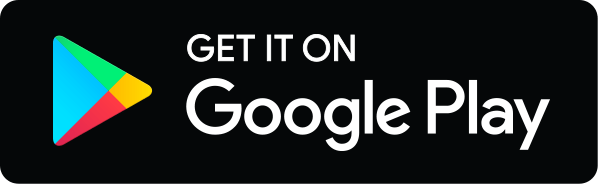