Anemia frequently accompanies and plays a minor role in the presentation and course of infection, whether parasitic, bacterial, or viral. However, a variety of infections, many of which are common in Africa and Asia, cause specific hematologic syndromes. The pathophysiology of these syndromes is complex, and to some extent, reduced red cell production may form part of an innate protective host response to infection. Across the world and in endemic areas, malaria is the most important among this group of infections and forms a major part of everyday practice.
Key points
- •
Malaria is responsible for approximately 600,000 deaths each year, although the epidemiologic picture over the last decade has been one of a substantial reduction in the burden of malaria.
- •
Malaria causes severe and life-threatening anemia by reducing erythropoiesis and increasing red cell destruction.
- •
Blood transfusion is beneficial in the group of children with malaria who have both anemia and respiratory distress, but bolus fluid supplementation of severely ill children on admission may result in higher mortality.
- •
Ill children should be carefully assessed clinically, and appropriate fluid or blood transfusion should be given to correct hypovolemia or severe anemia.
- •
Visceral leishmaniasis can cause considerable diagnostic difficulty and may be mislabeled as leukemia or myelodysplasia when the diagnosis of leishmaniasis was not considered.
Anemia frequently accompanies and plays a minor role in the presentation and course of parasitic, bacterial, or viral infection. However, a variety of infections, many of which are common in Africa and Asia, cause specific hematologic syndromes. The pathophysiology of these syndromes is complex and, to some extent, reduced red cell production may form part of an innate protective host response to infection. Across the world and in endemic areas, malaria, the most important among this group of infections, forms a major part of everyday practice across all clinical specialities and laboratory work. Several other parasitic diseases and bacterial infections, including visceral leishmaniasis, schistosomiasis, trypanosomiasis, hookworm, and bartonellosis, may present with major hematologic syndromes. These diseases may have restricted geographic distribution, but through travel, may present anywhere and must be recognized, diagnosed, and treated.
Malaria
Malaria is the most important parasitic illness of humans. The total burden of disease in 2013 was estimated to be 200 million episodes annually, and malaria is responsible for approximately 600,000 deaths each year. Despite the huge number of cases and deaths, the epidemiologic picture over the last decade has been one of a substantial reduction in the burden of malaria. The prevalence of infection and malaria-related mortality decreased dramatically in sub-Saharan Africa during the period 2000 to 2013. Across Africa, the average infection prevalence in children aged 2 to 10 years decreased from 26% in 2000 to 14% in 2013, a relative decline of 46%. Nevertheless, substantial problems remain for successful malaria control. There remains the perennial problem of increasing drug resistance of the malarial parasite and of resistance of the mosquito vector to insecticides used to impregnate bed nets, and malaria still remains one of the major global problems of public health.
The Life Cycle of Malaria Infection and Human Infection
Because of its peculiar life cycle ( Fig. 1 ), the malarial parasite is particularly prone to cause hematologic manifestations. Female anopheline mosquitoes inject sporozoites that enter liver parenchymal cells, where they proliferate into thousands of merozoites. Merozoites rupture from liver cells, pour into the bloodstream, and invade erythrocytes. Further development of the intraerythrocytic parasite follows 1 of 2 pathways: asexual differentiation or differentiation into sexual parasites called gametocytes. Asexual parasites develop from young ring forms through trophozoites to dividing forms called schizonts. On rupture of infected erythrocytes, forms called merozoites are released, invade other erythrocytes, and thus continue the erythrocyte cycle. When billions of schizonts rupture simultaneously and release cytokine-inducing toxins, they cause paroxysms of malarial fever.
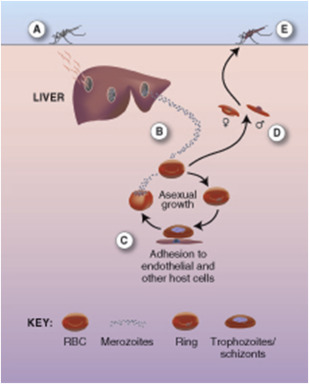
When a child is infected with malaria for the first time, the result is usually an intermittent febrile illness lasting a few weeks, although a significant proportion of children and a high proportion of nonimmune adults experience severe disease (see later discussion). In the phase between cessation of the fever and final resolution of the infection, the child may appear well, but the destruction of red cells continues. From a hematologic viewpoint, the major question is how soon will the child be reinfected, for in some communities reinfection occurs almost every day, and immunity to malaria is slowly acquired and never complete. In regions of high transmission, children eventually acquire the ability to maintain a parasite density below the level that causes fever, but chronic or repeated infections cause a state of chronic anemia.
Four species of Plasmodium infect humans— Plasmodium falciparum , Plasmodium vivax , Plasmodium ovale , and Plasmodium malariae —and a fifth species, Plasmodium knowlesi , normally restricted to macaque monkeys, has recently been discovered in the human population in Borneo. P falciparum is the predominant cause of clinical malaria in Africa and much of Southeast Asia, whereas P vivax tends to predominate in Central America and the Indian subcontinent. P vivax is essentially absent in populations of Central and West Africa because their erythrocytes fail to express the Duffy antigen or interleukin-8 (IL-8) receptor, to which the merozoites of this species attach during invasion.
Another peculiarity of P vivax , shared also with P ovale , is the ability to form hypnozoites, which can remain dormant in cells for months or years. This ability results in relapsing infections that are often associated with mild chronic anemia. However, profound anemia and other grave complications are almost always seen with P falciparum malaria, and the following sections apply mainly to this particular species.
Malaria
Malaria is the most important parasitic illness of humans. The total burden of disease in 2013 was estimated to be 200 million episodes annually, and malaria is responsible for approximately 600,000 deaths each year. Despite the huge number of cases and deaths, the epidemiologic picture over the last decade has been one of a substantial reduction in the burden of malaria. The prevalence of infection and malaria-related mortality decreased dramatically in sub-Saharan Africa during the period 2000 to 2013. Across Africa, the average infection prevalence in children aged 2 to 10 years decreased from 26% in 2000 to 14% in 2013, a relative decline of 46%. Nevertheless, substantial problems remain for successful malaria control. There remains the perennial problem of increasing drug resistance of the malarial parasite and of resistance of the mosquito vector to insecticides used to impregnate bed nets, and malaria still remains one of the major global problems of public health.
The Life Cycle of Malaria Infection and Human Infection
Because of its peculiar life cycle ( Fig. 1 ), the malarial parasite is particularly prone to cause hematologic manifestations. Female anopheline mosquitoes inject sporozoites that enter liver parenchymal cells, where they proliferate into thousands of merozoites. Merozoites rupture from liver cells, pour into the bloodstream, and invade erythrocytes. Further development of the intraerythrocytic parasite follows 1 of 2 pathways: asexual differentiation or differentiation into sexual parasites called gametocytes. Asexual parasites develop from young ring forms through trophozoites to dividing forms called schizonts. On rupture of infected erythrocytes, forms called merozoites are released, invade other erythrocytes, and thus continue the erythrocyte cycle. When billions of schizonts rupture simultaneously and release cytokine-inducing toxins, they cause paroxysms of malarial fever.
When a child is infected with malaria for the first time, the result is usually an intermittent febrile illness lasting a few weeks, although a significant proportion of children and a high proportion of nonimmune adults experience severe disease (see later discussion). In the phase between cessation of the fever and final resolution of the infection, the child may appear well, but the destruction of red cells continues. From a hematologic viewpoint, the major question is how soon will the child be reinfected, for in some communities reinfection occurs almost every day, and immunity to malaria is slowly acquired and never complete. In regions of high transmission, children eventually acquire the ability to maintain a parasite density below the level that causes fever, but chronic or repeated infections cause a state of chronic anemia.
Four species of Plasmodium infect humans— Plasmodium falciparum , Plasmodium vivax , Plasmodium ovale , and Plasmodium malariae —and a fifth species, Plasmodium knowlesi , normally restricted to macaque monkeys, has recently been discovered in the human population in Borneo. P falciparum is the predominant cause of clinical malaria in Africa and much of Southeast Asia, whereas P vivax tends to predominate in Central America and the Indian subcontinent. P vivax is essentially absent in populations of Central and West Africa because their erythrocytes fail to express the Duffy antigen or interleukin-8 (IL-8) receptor, to which the merozoites of this species attach during invasion.
Another peculiarity of P vivax , shared also with P ovale , is the ability to form hypnozoites, which can remain dormant in cells for months or years. This ability results in relapsing infections that are often associated with mild chronic anemia. However, profound anemia and other grave complications are almost always seen with P falciparum malaria, and the following sections apply mainly to this particular species.
Severe malaria
Life-threatening complications are estimated to occur in about 1% of episodes of P falciparum infection in African children, and it has been estimated that a child in rural Africa has a 15% lifetime risk of a malaria infection requiring hospital admission as a result of complications. Such complications include profound anemia, cerebral malaria (a syndrome of unarousable coma, often accompanied by severe convulsions), hypoglycemia, jaundice, renal failure, pulmonary edema, and coagulation abnormalities. In Southeast Asia, all the aforementioned complications are commonly seen, whereas in Africa, cerebral malaria, severe malarial anemia, and hypoglycemia are common, but the other complications of P falciparum malaria are surprisingly rare. Malarial illness affects mostly children in Africa, where transmission rates are high, whereas adults are more commonly affected in some other parts of the world. It appears that higher rates of malarial transmission lead to a greater prevalence of anemia but, paradoxically, a low incidence of cerebral malaria.
The lethality of P falciparum as compared with other species of Plasmodium probably stems from 2 biologic properties. First, the parasite density that it achieves is typically a hundred times higher than that of other species before its growth is curtailed by host defense mechanisms. Second, mature intraerythrocytic forms of P falciparum adhere to the endothelium of postcapillary venules and thus sequester in tissues. Parasite sequestration is mediated by a parasite-derived molecule known as PfEMP1 that is expressed on the surface of a mature infected erythrocyte and binds to a variety of endothelial adhesion molecules, including CD36, intracellular adhesion molecule-1, E-selectin, and vascular cell adhesion molecule-1.
Another potentially important pathophysiologic mechanism is vascular sludging, which results from the clumping of parasitized erythrocytes, with either unparasitized erythrocytes (“rosettes”) or platelets (“autoagglutination” or “clumps”).
There is an association between salmonellosis and malarial infection. This association goes beyond the growing recognition that a significant proportion of cases of severe malaria in the tropics is bacteremic in that salmonellosis in Africa seems to be specifically associated with malarial anemia.
Malarial Anemia
The pattern of hematologic changes in malaria varies considerably, depending on the type of patient. During an acute attack of P falciparum malaria in a nonimmune person, the hematocrit starts to decrease after 1 or 2 days and continues to decrease for about 1 week after antimalarial treatment. Subsequently, there is usually a steady increase in the hematocrit, although it may take several weeks before the hematologic picture is back to normal. The anemia, which is not usually life-threatening, is characterized by both hemolysis and an ineffective marrow response, features that have also been documented in acute P vivax infection. When profound anemia occurs with acute P falciparum malaria, it is often associated with multi-organ failure, although it can occur as an isolated complication, particularly in African children.
From the perspective of tropical child health, the most important hematologic problem is seen in a child chronically infected with P falciparum , whose anemia is debilitating and sometimes fatal. The most striking pathophysiologic findings are hemolysis, hypersplenism, and a suboptimal bone marrow response ( Fig. 2 ). In the sections that follow, some of the mechanisms for these components of the acute and chronic anemia of malaria are discussed in more detail.
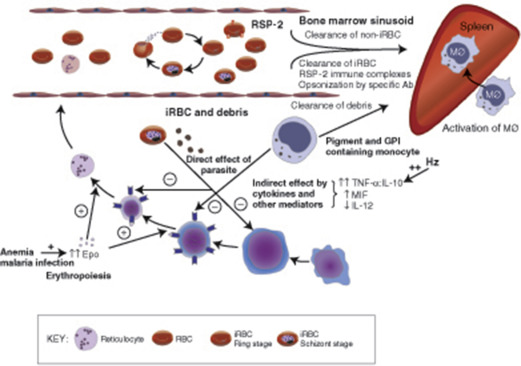
Role of Hemolysis
Normal children living in rural Africa typically have extremely low levels of haptoglobin that increase significantly after reduced transmission, thus providing some indication of the burden of chronic hemolysis that malaria causes in many tropical communities. The mechanisms of red cell destruction in P falciparum malaria are complex and not fully understood. Clearly, erythrocytes are destroyed when schizonts rupture and release their progeny, and a proportion of the parasitized erythrocytes are destroyed by the host before schizont rupture can take place. In most infections, less than 1% of erythrocytes are infected, a loss that is important in the context of chronic infection but would not account for the severity of anemia that is commonly observed. Both mathematical modeling and clinical observation suggest that 10 times as many uninfected erythrocytes are removed from the circulation for each infected erythrocyte. Indeed, cross-transfusion experiments clearly indicate that erythrocyte survival is shortened both in the acute phase of malaria and during convalescence.
Role of the Spleen
Some degree of splenomegaly is a normal feature of malarial infection, and the prevalence of splenomegaly in regions of malarial transmission is used as a major indicator of the level of malarial endemicity. The phenomenon of parasitic sequestration, discussed earlier, is thought to have evolved primarily as an immune evasion strategy whereby the mature parasite can avoid passing through the spleen.
Several studies have attempted to define the pathophysiologic changes in the spleen during acute malaria. In studies of human malaria, it has been found that increased splenic clearance of heated red cells occurs during acute attacks. Reduced deformability of uninfected red cells is observed in P falciparum malaria and is a significant predictor of the severity of anemia, consistent with the notion that these cells are being removed by the spleen. It has also been found that immunoglobulin G (IgG) -sensitized red cells are rapidly removed from the circulation by the spleen and that unusually rapid clearance persists well into the convalescent phase. Undoubtedly, the activity and number of macrophages are increased during human malarial infection, and this may therefore also contribute to the increased removal of uninfected cells.
Clinical studies have also uncovered a curious phenomenon whereby some erythrocytes appear to be returned to the circulation after having had their parasites removed, but killing parasites usually means destroying erythrocytes, and it appears that the survival of uninfected red cells is reduced in the process.
Experimental evidence supports the notion that with relatively nonspecific effector mechanisms such as erythrophagocytosis within the spleen, anemia is part of the price that the host has to pay for protection against overwhelming parasitemia.
Massive Intravascular Hemolysis
A peculiar and striking example of malaria-associated hemolysis is blackwater fever. The classic form of this syndrome, often reported in colonial times among Europeans living in Africa, was characterized by fever and massive intravascular hemolysis associated with low or no parasitemia. It was suspected that intermittent quinine ingestion might have led to a drug-induced immune hemolysis, although this mechanism was never proved and recent reports have implicated newer antimalarial drugs, such as halofantrine and mefloquine/artemisinin combinations. Classic blackwater fever is now much less common, but massive intravascular hemolysis remains an important complication of P falciparum malaria in Southeast Asia in both children and adults. A survey of cases in Vietnam found considerable overlap of quinine ingestion, glucose-6-phosphate dehydrogenase deficiency, and concurrent malaria, thus suggesting that these different factors may interact but certainly do not all have to be present for blackwater fever to occur.
Decreased Erythrocyte Deformability
The increased clearance of uninfected erythrocytes is due, not only to the activation of splenic macrophages, but also to extrinsic and intrinsic factors that enhance their recognition and phagocytosis. Uninfected erythrocytes have reduced deformability, although the mechanism responsible for the loss of deformability is not completely understood. However, increased oxidation of membranes in uninfected erythrocytes has been demonstrated in children with severe P falciparum malaria, and the ongoing inflammatory insults associated with acute malaria (proinflammatory cytokines) or the direct effects of parasite products have been shown to cause loss of red cell deformability. Intriguingly, a severe reduction in red cell deformability measured on admission is also a strong predictor, not just for anemia, but also for mortality in both adults and children with severe malaria.
Immune Complex–Mediated Hemolysis
It is also possible that at least part of the hemolysis in P falciparum malaria might have an immune basis. A positive direct Coombs antiglobulin test (DAT) is seen in some patients with malaria. However, in Thai children or adults during their first attack or with subsequent attacks in those who live in areas where a degree of immunity has not developed, DAT results are invariably negative.
The position is different in African children who have suffered repeated attacks of malaria. Positive DAT results have been found in up to 50% of these children, and the incidence is significantly higher in children with active malarial infection than in those who are not infected. DAT results may remain positive for several weeks after an acute infection. The most common type of red cell sensitization occurs with C3. The IgG that can be eluted from these cells has specific activity against P falciparum schizont antigen, which suggests that the erythrocyte coating results from passive attachment of circulating complement-fixing malarial antigen-antibody complexes. It is therefore likely that the development of positive DAT results is part of the immune response to P falciparum .
Other studies have suggested that the P falciparum ring surface protein 2 (RSP-2) may be one component of the immunoglobulin-antigen complexes deposited on uninfected erythrocytes. This protein is expressed on infected erythrocytes shortly after merozoite invasion. RSP-2 is also deposited on uninfected erythrocytes and forms immune complexes that contribute to the phagocytosis of uninfected erythrocytes. Limited clinical studies have shown that high levels of anti-RSP-2 antibodies are found in the sera of immune adults and children with severe anemia.
However, it is far from clear whether the presence of positive DAT results indicates that immune destruction of red cells occurs in African children with malaria. Several studies have shown that there is no correlation between the degree of anemia and positive DAT results, and other measured parameters of hemolysis have not correlated with coating of red cells.
In short, there is little evidence for immune destruction of red cells in P falciparum malarial infections in nonimmune adults. Although a varying proportion of children with chronic malaria in Africa has positive DAT results, only occasionally is evidence of genuine immune destruction of red cells seen. However, the persistence of shortened red cell survival of nonparasitized cells in the absence of any consistent serologic abnormalities suggests that there must be another factor involved in the shortened red cell survival in malaria. This factor appears to be nonspecific over-activity of the monocyte/macrophage populations of the spleen and liver, as discussed earlier.
Defective Marrow Response
In addition to the hemolytic components of the anemia of P falciparum malaria, there is undoubtedly an inappropriate marrow response. The reticulocytosis in response to the decrease in hematocrit is often inappropriately low and delayed (see Fig. 2 ). It remains unclear whether defective erythropoietin production is a significant cause, with some studies suggesting an appropriate increase or even enhanced response and others observing a blunted response, but there is no question that significant dysfunction of marrow takes place and appears to have a complex, multifactorial basis.
Some of the features of iron metabolism and bone marrow morphology and function in acute malaria resemble those of other acute infections. Thus, the serum iron level rapidly decreases and iron appears to be sequestrated into the storage compartments of the marrow. It is now clear that disturbances in iron metabolism are mediated by hepcidin. Hepcidin is regulated by pro-inflammatory mediators, such as tumor necrosis factor (TNF) and IL-6, which are elevated in both murine infections and in patients presenting with severe falciparum malaria, although other parasite-derived and host factors may be involved in stimulating hepcidin production. High hepcidin levels stimulated by blood stage malaria infection are associated with inhibition of liver stage malaria infection in mice and reduced incorporation of iron into red cells in humans. Hepcidin levels decrease in the most severely ill children as hypoxia inhibits hepcidin production. Evidence from murine malaria suggests that low serum iron caused by elevated hepcidin reduced parasite growth and progression to severe disease. These experimental and clinical findings are consistent with a role for iron as a growth factor for malaria parasites and a role for raised hepcidin and reduction in available iron as a protective innate response to malaria infection.
During acute malarial infections, there are marked dyserythropoietic changes in the bone marrow. These morphologic abnormalities, which have been studied by both light and electron microscopy, consist of erythroblast multinuclearity, karyorrhexis, incomplete and unequal amitotic nuclear divisions, and cytoplasmic bridging ( Fig. 3 ).
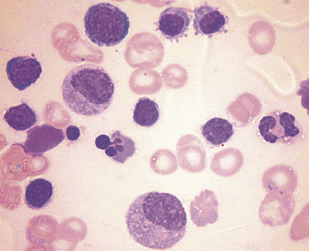
There is a significant abnormality of red cell proliferation in the bone marrow that occurs in acute malaria. Changes include an increased proportion of red cell precursors in the G2 phase and arrest in progress of cells in the S phase. Gene expression is profoundly altered in erythroid precursors exposed to malarial products, and this pattern of dyserythropoiesis is distinct from dyserythropoiesis induced by inflammatory cytokines.
In addition to these dyserythropoietic changes, erythrophagocytosis is particularly common in the bone marrow in P falciparum malaria. This phenomenon is not restricted to the marrow and may be seen in the spleen and other organs and, as mentioned earlier, may play a role in the hemolytic component of the disease.
Although the mechanism of dyserythropoietic changes is unknown, it was thought possible that they are an exaggerated example of the bone marrow suppression that occurs in other situations of chronic infection. An important factor may be the high levels of TNF production that occur during malarial infection because this cytokine has been strongly implicated in the anemia of chronic infection, and severe malarial anemia has been associated with certain TNF promoter polymorphisms. TNF suppresses the proliferation of erythroid progenitor cells in human marrow culture, although the effect declines as the cells differentiate. On the other hand, TNF stimulates fibroblasts to secrete growth factors for colony-forming-unit–granulocyte-erythrocyte-monocyte-macrophage and burst-forming-unit–erythrocyte. Furthermore, experimental findings in mice are consistent with a role for TNF in the dyserythropoietic changes of malaria.
IL-10 is an anti-inflammatory cytokine, and 2 clinical studies in African children with severe malarial anemia have found a low ratio of IL-10 to TNF in plasma, thus leading the investigators to propose that defective IL-10 production may pave the way to marrow suppression by TNF. Anti-inflammatory cytokines may be associated with a more favorable outcome. IL-12 protects against severe anemia in experimental murine malaria, which might reflect both its antiparasitic actions and its effects on erythropoiesis.
Several studies have suggested that a parasite byproduct of hemoglobin digestion, hemozoin, may have an indirect or direct role in impaired erythroid development. Hemozoin stimulates the secretion of biologically active endoperoxides from monocytes, such as 15(S)-hydroxyeicosatetraenoic acid and hydroxynonenal, via oxidation of membrane lipids, which may affect erythroid growth.
Hemozoin and TNF-α also have additive effects on erythropoiesis in vitro , and in a clinical study, hemozoin-containing macrophages and plasma hemozoin were associated with anemia and reticulocyte suppression. Moreover, bone marrow sections from children who died with severe malaria show a significant association between the quantity of hemozoin (located in erythroid precursors and macrophages) and the proportion of erythroid cells that were abnormal. These findings are consistent with a direct inhibitory effect of hemozoin on erythropoiesis.
Coagulation Abnormalities
Modest thrombocytopenia is not uncommon during acute P falciparum attacks, and occasionally, the platelet count decreases to as low as 10,000 to 20,000/mL. The mechanisms have not been determined but probably involve platelet activation and adhesion of infected erythrocytes to platelets, and experimental studies in mice suggest that platelets sequester in venules during malarial infection.
In some patients with severe P falciparum malaria, a severe bleeding diathesis may develop during the acute phase of the illness. Although it has been suggested that the diathesis results from disseminated intravascular coagulation, studies in Thailand have not substantiated this supposition. Rather, the bleeding appears to reflect gross thrombocytopenia together with liver damage. However, it is becoming apparent that approximately 10% of African children with severe malaria also have bacteremia, so when disseminated intravascular coagulation occurs, the cause may be complex.
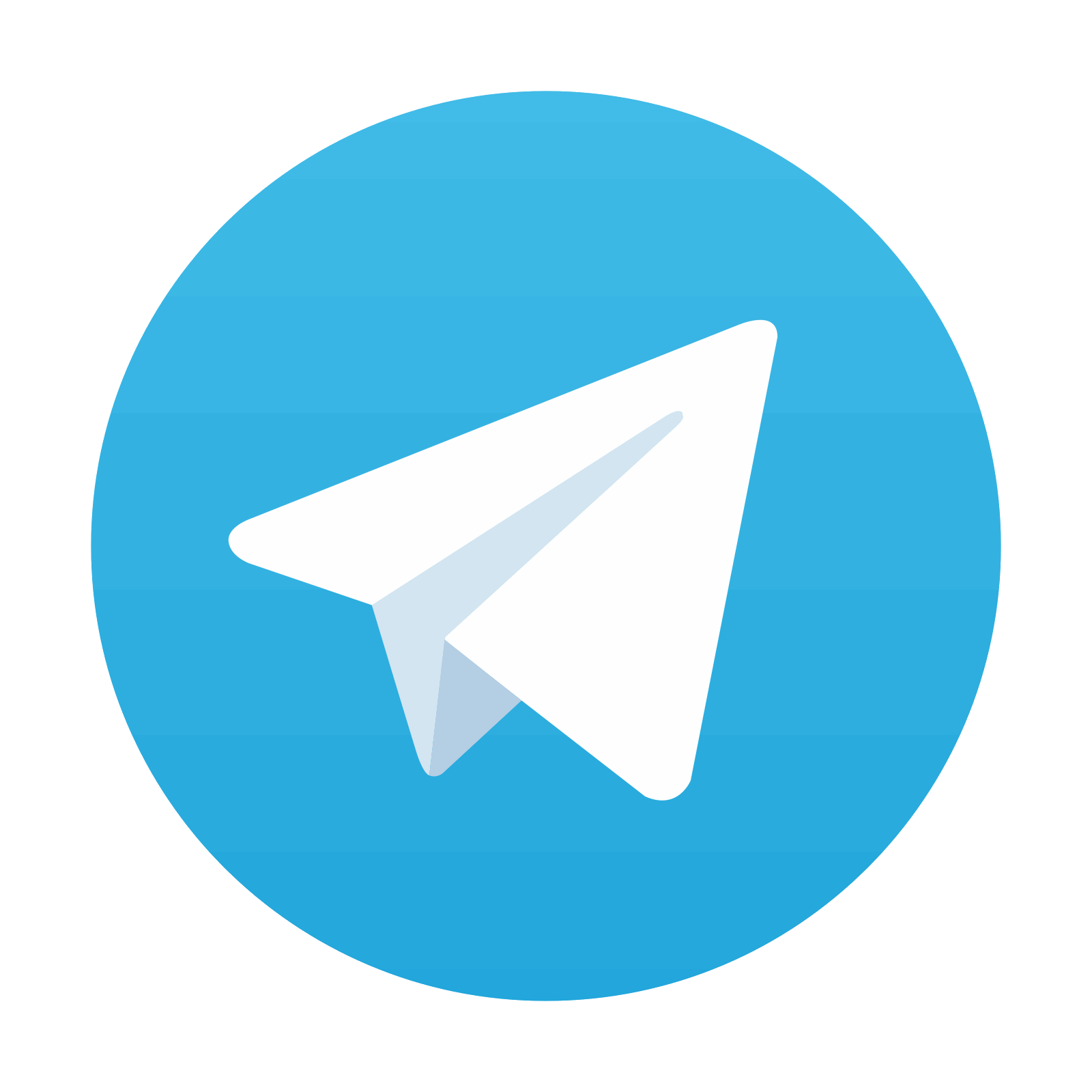
Stay updated, free articles. Join our Telegram channel

Full access? Get Clinical Tree
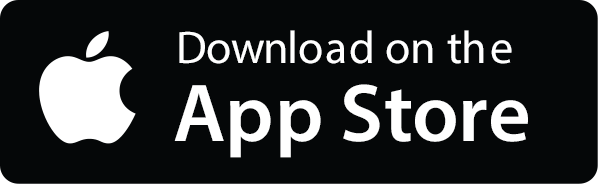
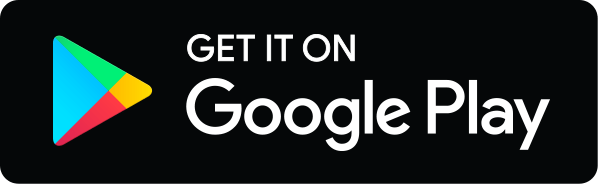