Fig. 10.1
Nasopharyngeal carcinoma. (a) H&E-stained section of tumor cells-associated lymphocytes, 600× magnification. (b) CISH for EBER from the same tissue block as (a), 600× magnification
Additional Applications
Another scenario in which CISH for EBER may be helpful is the investigation of metastatic carcinoma of unknown primary (CUP). Frequently these cases present as a cervical lymph node or nodes positive for a poorly differentiated carcinoma without clear evidence of the tissue of origin. The differential diagnosis includes squamous cell carcinoma of a head and neck mucosal site, squamous cell carcinoma of the skin, nasopharyngeal carcinoma, sinonasal carcinoma, tumors of salivary gland origin, and even lymphoma when a particularly exuberant lymphoid background is present. A positive EBER CISH directs one to focus the clinical workup toward the nasopharynx. This application is also recommended in the CAP cancer protocol for carcinomas of the pharynx and NCCN guidelines for cancer of the head and neck [51, 52].
Investigational Applications of EBV Testing in NPC
Quantitative EBV DNA viral load testing from serum or plasma has been examined in a number of contexts ranging from screening through post-treatment follow-up. Many studies were undertaken in the context of current serologic tests for EBV which target viral capsid antigen (VCA) IgA most commonly. EBV DNA can be reliably detected in either serum or plasma specimens however, a comparable clinical specificity but higher clinical sensitivity is reported when using plasma as the initial sample source [53–64]. Most of the studies examining EBV viral load have used quantitative real time PCR targeting BamHI-W DNA.
Effective screening for nasopharyngeal carcinoma from plasma is attractive as the disease is often not diagnosed until a late stage because of the delayed observation of recognizable symptoms. Additionally, the difference in cure rate is pronounced with reports of 85 % cure rate in early stage disease versus lower than 30 % in advanced stage disease [65]. One study showed sensitivity of 90.6 % and specificity of 93.5 % in a case control study using real time PCR targeting EBV DNA from plasma specimens to predict the presence of NPC in newly diagnosed individuals (cases) and high-risk individuals (control group). They showed an increase in sensitivity when coupling the assay with a serologic EBV VCA IgA assay. This seems a reasonable and promising line of investigation in areas with high NPC incidence (up to 20–30 cases per 100,000 people in endemic areas) [62, 66]. However, in the west, this is not a feasible screening technique as our pre-test probability is distorted and the cost would be great to identify very rare benefit.
EBV viral load does roughly correlate with clinically assigned stage of NPC. Stages II–IV are reliably detected with sensitivities of 91–100 % reported. Stage I shows false negative rates from 14 to 50 % depending on the study [67–69]. Attempts to use viral load for prognostic information have had limited success. Some groups have attempted to identify dichotomous groups of high risk or low risk. Using viral load data to this end generally clusters patients by stage as I–II (low risk) and III–IV (high risk) which is not clinically useful as treatment is not affected by this information. However, rare stage I–II patients were identified with a high-risk viral load. Patients of this type could potentially be steered into a clinical trial with intensified treatment such as adding chemotherapy to the standard radiotherapy given to patients of this stage [70–73].
Monitoring for treatment response and relapse is another area with past and presumably future investigational interest. The NCCN guidelines for cancers of the head and neck has a recommendation within the section outlining follow-up procedures that advises consideration of EBV DNA monitoring in the setting of NPC [51]. No further explanation or references are present in association with this statement.
Currently, some general trends have been observed, but sufficient evidence to offer specific guidance has not yet been generated. In broad terms, a dramatic decrease in viral load to 0 or at least levels below the detection limit of a given assay corresponds to treatment response or remission, but does not indicate that the response will be sustained. A subsequent elevation in viral load generally indicates disease progression and is usually detected earlier than clinical signs of progression. A drop in viral load with significant residual virus detected generally corresponds to inadequate treatment response and likely progression of disease [74–77].
Expected goals of additional studies would be to clearly define testing parameters such as optimal timing of test performance as well as consensus viral load values for decision points. For example, one group suggests reproducible data with testing pretreatment and at 6 weeks post treatment. Viral load threshold for positive versus negative should be established as well as one to dichotomize high-risk and low-risk subgroups within the positive category [78]. Extensive validation of the findings with large scale studies and interlaboratory comparisons is necessary before interpreting data for clinical use. A major challenge in studies of this type is interlaboratory variability in viral load determination. Comparison using the same samples across labs or a predetermined calibrator to calculate an international standard value is necessary to validate the reproducibility of the assay in individual laboratories. Applications of an assay of this type would be indication of early relapse and subsequent radiologic workup to identify tumor growth or additional treatments such as addition of adjuvant chemotherapy [74, 79, 80]. Also of note, false negative rates from 48 to 14 % for local/regional recurrence versus distant metastasis respectively have been reported [80–82].
HPV Testing for Squamous Cell Carcinomas of the Head and Neck
Molecular oncology testing for the presence of high-risk HPV (human papilloma virus) subtypes in squamous cell carcinoma of the head and neck (HNSCC) discernibly affects prognosis and, in selected clinical circumstances, diagnosis. Consensus opinion regarding alteration of treatment regimens on the basis of HPV test results has not yet been achieved. However, the possibility of altered treatment regimens should enter in to the selection of HPV evaluation in this setting.
Squamous cell carcinoma of the head and neck is a malignant epithelial neoplasm with squamous differentiation and a tendency for early regional nodal metastasis [1]. Traditionally, cases of HNSCC were considered related to exposure to tobacco and alcohol. This is still true in many cases. However, a subset of cases morphologically, anatomically, and mechanistically, trend toward distinction from the classic cases. Within this subset, tumorigenesis is secondary to persistent infection with high-risk HPV. Alcohol and tobacco-related cases tend to occur in the fifth–sixth decades, the HPV-related HNSCC (HPV-HNSCC) cases tend to occur at a younger age, particularly less than age 60 [83]. Additional risk factors identified predominantly in young men diagnosed with HNSCC include young age at first sexual intercourse, a history of multiple sexual partners, and a history of genital warts. These risk factors mirror those observed in women with cervical carcinoma and known HPV exposure [83, 84].
Anatomically, the majority of HPV-HNSCC cases involve the oropharynx with a strong predilection to the palatine and lingual tonsils and the base of the tongue [85]. Primary cases of HPV-related HNSCC outside of the oropharynx are significantly less common and not routinely subjected to further testing. The typical HNSCC arises from progressive dysplasia of the mucosal surface. This results in an invasive epithelial tumor with nests of cells with some degree of keratinization. Although, there is clearly morphologic variability, features consistent with HPV-HNSCC include those mimicking the reticular epithelium of the tonsils from which they commonly arise. The HPV-associated tumors frequently appear to originate from the deep crypt epithelium rather than the surface epithelium. Additionally, the transition between tumor and normal tissue tends to be abrupt in HPV-HNSCC rather than transitional from dysplasia to carcinoma as is frequently seen in HNSCC unrelated to HPV. The HPV-HNSCC tumors are frequently composed of nests and sheets of cells with abundant tumor-infiltrating lymphocytes [85, 86]. Cytologically, the cells have large nuclei and scant cytoplasm giving the tumors a basaloid appearance [85]. The basaloid phenotype of HPV-HNSCC can overlap with that of basaloid squamous cell carcinoma. The presence of HPV can be used to distinguish the two which is important as they lie on opposite ends of the prognostic spectrum. The presence of HPV generally denotes a favorable prognosis relative to that of HNSCC of other etiologies. An important exception is that of HPV-HNSCC with small cell features, aptly dubbed human papilloma virus-related small cell carcinoma of the oropharynx [87]. The degree of improvement of prognosis in individuals with oropharyngeal HNSCC ascribed to HPV positivity can be most clearly outlined by dividing the patients into high, intermediate, and low-risk groups. The low risk group is composed individuals positive for HPV with less than or equal to a ten pack/year smoking history or greater smoking history with nodal stage of N2a or lower. Those positive for HPV with greater than a ten pack/year smoking history and nodal status of N2b or greater are considered intermediate risk group members. The other component of the intermediate group is composed of HPV negative individuals with less than or equal to ten pack/years of smoking history and stage T2 or T3 disease. The high-risk group is composed of individuals with greater than ten pack/years smoking history or less smoking history with stage T4 disease. Defined as such, the 3-year survival estimate is 46 % for the high-risk group, 71 % for the intermediate risk group, and 93 % for the low risk group [88].
HPV is a double-stranded DNA virus in a circular configuration. The high-risk subtypes are those associated with tumor formation. Types 16 and 18 represent the most commonly observed high-risk subtypes, however, many other high-risk subtypes including 31, 32, 33, 34, 35, 45, 51, 52, 56, 58, 66, 68, 70, and 73 have been identified [89]. The virus genome is composed of six early genes (E1, E2, and E4-E7) along with two late genes (L1 and L2) [90]. The viral L1 and L2 genes are involved in producing the viral capsid and have not been shown to be directly important in carcinogenesis. The viral E1 and E2 early genes directly interact and function in regulating viral DNA replication [91–93]. E2 has additional functions in regulation of gene transcription. At low concentrations, the presence of E2 protein leads to promotion of transcriptional activity. However, at higher concentrations, the effect of E2 is inhibitory to transcription of genes including E6 and E7 [93]. Of note, the virus can reside in the cell in an episomal form or be integrated into the host cell’s genetic material. During the process of integration, an open reading frame of E2 is disrupted leading to the loss of E2 function and in theory the inhibitory effect on the transcription of E6 and E7 [93]. The details of the functions of E4 and E5 are not completely elucidated. The most important genes in relation to tumorigenesis are E6 and E7 genes from high-risk HPV subtypes. Likely the most influential and most commonly cited tumorigenic function of the E6 protein is binding of the E6-associated protein and subsequent binding and degradation of the p53 protein via the ubiquitin pathway [94]. E6 has at least 14 other well-described binding partners leading to enhanced proliferative effects via activation of telomerase and SRC kinases along with antiapoptotic effects via interaction with BAK [95, 96]. The dominant tumorigenic effect of the E7 protein is the ubiquitin mediated degradation of pRB leading an increase in transcription secondary to the release of transcription factor E2F [96, 97]. E7 interacts with at least ten other substrates resulting in additional effects leading to increased proliferation by escaping cell cycle controls and contributing to the induction of aneuploidy [97, 98]. Additionally, there is an element of synergy between the effects of E6 and E7 which have ramifications on testing methods. The increase in free E2F leads to upregulation of p16. The increase in E2F would, under normal circumstances, trigger cell death. However, this trigger is subverted by the downstream antiapoptotic effects of E6. The p16 protein has an inhibitory effect on E6. However, this effect on E6 is abrogated as E7 inactivates this function of p16 [96, 97]. Detection of the increase in p16 is exploited as a surrogate marker of high-risk HPV transcriptional activity.
When to Test
Testing is recommended for diagnostic and prognostic purposes in HNSCCs of the oropharynx. Additionally, testing is recommended in the context of metastatic carcinoma of the cervical lymph nodes with unknown primary. Testing in these situations is supported in both the CAP cancer protocols and NCCN guidelines [52, 99]. In both of the above cases, diagnostic information is gained. In the case of carcinoma of unknown primary, the site of origin of oropharynx is suggested leading to a more focused search. Additionally, NCCN guidelines suggest that in the future, HPV positivity may be used to define radiation therapy fields in the absence of grossly identified tumor [99].
Methodology
The goal of testing for HPV in HNSCC is to identify a clinically relevant presence rather than a benign viral passenger. Therefore, the testing is directed specifically at high-risk subtypes. A clinically relevant infection is generally represented by virus which has integrated into the host genome and is transcriptionally active, especially with regard to the oncogenic products of E6 and E7. Detection of genetic material has been examined extensively in basic science and clinical laboratories. These techniques can be directed at a specific subtype or pools of subtypes based on the selection of primers. Detection of E6 and E7 mRNA via reverse transcriptase PCR is considered the gold-standard technique for determination of clinically significant high-risk HPV detection. However, this technique is rarely employed clinically as surrogate techniques have shown comparable clinical performance and wider accessibility in smaller laboratories.
Some controversy exists as to the algorithmic approach to testing and which tests should be employed. One line of thought hinges on a small subgroup of cases which are p16 positive but negative for HPV by DNA or RNA methods. This problem can be treated by beginning with p16 immunohistochemistry. If positive, one can then perform ISH directed at HPV subtype 16 or a combination of high-risk subtypes for confirmation [100]. This approach is reasonable, but requires multiple tests and increased expense. As noted above, many believe the gold standard in HPV detection to be detection of mRNA from E6 or E7. Another group rejects this concept and uses overall and progression free survival outcome data as the gold standard. This notion is based on the practical information that is sought by testing. Additionally, this approach is advocated because of the poor characterization of the p16 positive/HPV mRNA or DNA negative subgroup in the current literature. This group shows that p16 positivity corresponds to the positive prognostic information independently. Additionally, other methods including mRNA detection or RNA ISH, DNA ISH, or PCR for DNA do not show a statistically significant improvement in correlation with positive prognosis [101]. Therefore, there is reasonable data to suggest p16 immunohistochemistry alone is sufficient to assess HPV status in the workup of squamous cell carcinoma of the oropharynx or metastatic carcinoma of unknown origin in the cervical lymph nodes. It is left up to individual labs whether they want to perform follow-up testing most commonly in the form of CISH. The use of either technique is supported by CAP protocols and NCCN guidelines [52, 99]. At this point additional DNA or RNA testing is usually not necessary.
In fact, detection of HPV DNA, frequently employing primers specific to L1, have frequently been shown to be too analytically sensitive leading to decreased clinical specificity. In other words, the test frequently identifies clinically insignificant HPV DNA which could lead to false assignment of HNSCC cases as HPV related. In studies comparing DNA detection methods to E6/E7 mRNA expression, a range from 14 to 50 % of HPV DNA-positive tumors proved negative for E6/E7 expression [102, 103].
IHC and CISH methods are typically preferred by surgical pathologists when applicable as the technique blends easily into workflow, is commonly available in a wide variety of labs, and allows the localization of the result within the architectural context of the tissue.
Interpretation of Results
Interpretation of p16 staining is straightforward and reproducible. Tumor tissue is considered positive when ≥70 % of cells demonstrate strong cytoplasmic and nuclear staining (Fig. 10.2) [100]. Note that these criteria differ from those used in the interpretation of p16 IHC in the setting of cervical or anogenital squamous cell carcinoma cases.
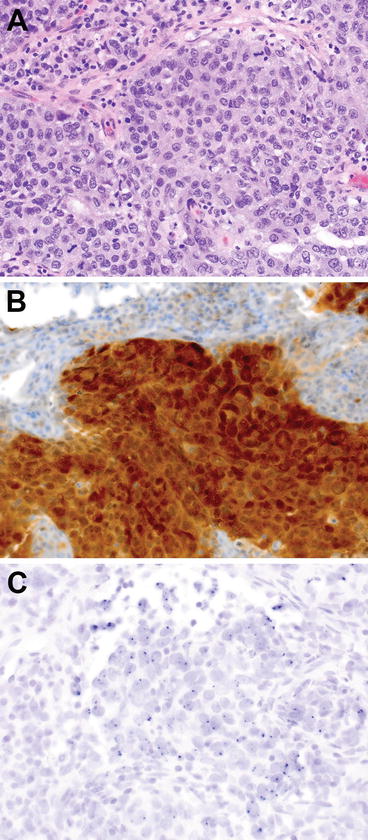
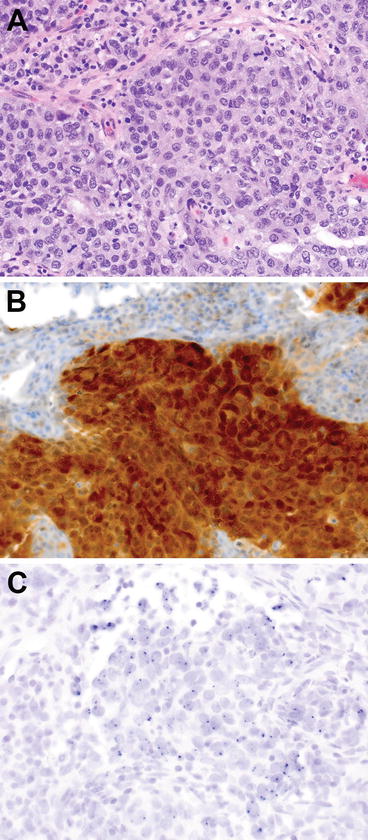
Fig. 10.2
Oropharyngeal carcinoma. (a) H&E-stained section of tumor cells, 600× magnification. (b) Same tissue an immunohistochemical stain for p16. Note the strong nuclear and cytoplasmic staining in greater than 70 % of cells, 600× magnification. (c) CISH for HPV subtype 16 DNA. Note the dot-like nuclear positivity, 600× magnification
ISH is generally interpreted as positive or negative. Either a punctate pattern or diffuse nuclear chromogen pattern is considered positive. Discrete, dot-like staining is considered indicative of integration of the virus into the host genome (Fig. 10.2). A truly diffuse nuclear pattern is likely to represent an episomal viral pattern of infection. Absence of both patterns within lesional cells is necessary to consider a sample negative [104].
Future Perspectives
As continued development of RNA ISH techniques progresses and more samples are compared, this technique may be a contender to surpass p16 IHC. Additional studies are necessary to determine this outcome.
An intermediate prognostic category exists between that of traditional alcohol/tobacco-related HNSCC and HPV-associated HNSCC. In addition to p16 positivity, these tumors frequently harbor inactivating mutations in TP53 (p53) and overexpression of EGFR, changes usually associated with alcohol/tobacco-related HNSCC. Investigation to evaluate whether additional testing for these molecular changes, or detailed historical information pertaining to tobacco consumption and alcohol intake may be adequate to alter the assignment of prognostic group are likely future areas of investigation.
Although improved prognosis is well documented, it is currently not recommended to alter treatment protocols based on p16 or HPV testing status alone. The only setting in which this is considered reasonable is that of well-controlled clinical trials which will likely be forthcoming.
Pathogenic Translocations in Salivary Gland Cancers
Background
Translocations and other chromosomal rearrangements resulting in fusion oncogenes have been identified in salivary gland tumors. Rearrangements may result in overexpression of one of the involved genes or expression of an abnormal chimeric gene. The major targets involved in these rearrangements are transcription factors, transcriptional coactivators, and tyrosine kinases. As a group, the translocations result in activation of growth factor signaling, transcriptional activation, and cell cycle progression. Knowledge of these recurrent chromosomal abnormalities is useful diagnostically and could have therapeutic implications in the future.
Pleomorphic adenoma is the most common salivary gland tumor and occurs most frequently in the parotid gland. Pleomorphic adenoma is associated with gene rearrangements involving PLAG1 and HMGA2. PLAG1 is a transcriptional activator located at 8q12.1. Translocations of PLAG1 result in gene overexpression and ultimate activation of the Insulin-like growth factor-2 signaling pathway [105]. Common translocation partners with PLAG1 are the β-catenin gene (CTNNB1) on 3p21 and the leukemia inhibitory factor receptor (LIFR) gene on 5p13-12 [106–108]. The t(3;8)(p21;q12) results in activation of PLAG1 and reduced expression of β-catenin [107]. Variant, complex, rearrangements such as t(1;4;8), t(1;5;8), and ins(9;8) involving PLAG1 one have also been described [106, 108]. Rearrangements of 8q12 telomeric or centromeric to PLAG1 have also been identified [106, 109, 110]. Some of these cases have been found to harbor intrachromosomal translocations resulting in CHCHD7-PLAG1 or TCEA1-PLAG1 fusions [109]. Amplifications of PLAG1 have also been reported [106]. Rearrangements of HMGA2, located at 12q15, result in fusions that cause overexpression of HMGA2 and its cell cycle regulator target genes, CCNA1 and CCNB2 [111, 112]. Amongst salivary gland tumors, PLAG1 and HMGA2 rearrangements are specific for pleomorphic adenoma and therefore diagnostically useful [113].
Mucoepidermoid carcinoma is the most common salivary gland malignancy, occurs in major and minor salivary glands, and can occur across a broad age range. Nearly two-thirds harbor a recurrent translocation, t(11;19)(q21–22;p13), which can be diagnostically useful [114, 115]. The translocation results in fusion of exon 1 of CRTC1 (also known as MECT1 and TORC1) on 19p13 to exons 2–5 of MAML2 on 11q21–22 [113, 116, 117]. Variant translocations resulting in CRTC3-MAML2 fusions have been identified [118, 119]. Seventy-five percent of mucoepidermoid carcinomas have been found to harbor a MAML2 translocation, and 60 % to harbor the CRTC1-MAML2 specifically [120]. Both CRTC1 and MAML2 genes are involved in cell cycle regulation and the fusion results in activation of c-AMP response element binding (CREB) mediated transcription and notch signaling pathways [116, 117, 121, 122]. Although earlier studies reported the translocation to be limited to low- and intermediate-grade mucoepidermoid carcinoma, more recent studies have confirmed high-grade cases to also be positive for t(11;19)(q21–22;p13) [115, 120, 123, 124]. The translocation has not been identified in other salivary gland malignancies [115, 123, 124]. Data is conflicting regarding the presence of the translocation in benign Warthin tumor [115, 117, 123–126]. Oncocytic mucoepidermoid carcinoma is in the differential with Warthin tumor as is mucoepidermoid carcinoma with a brisk lymphocytic infiltrate. Warthin tumor cases with CRTC1-MAML2 fusions may actually be misclassified mucoepidermoid carcinomas [125].
Adenoid cystic carcinoma, an aggressive yet slow growing malignancy, is the second most common salivary gland malignancy, and is the most common malignancy affecting the minor salivary glands. A t(6;9)(q22–23;p23–24) results in a MYB-NFIB gene fusion and is a recurrent translocation identified in adenoid cystic carcinoma [127, 128]. This fusion is highly specific for adenoid cystic carcinoma as it has not been identified in other head and neck tumors and is therefore potentially useful in distinguishing adenoid cystic carcinoma from other tumors with basaloid morphology [113, 129]. MYB, located at 6q22–23, functions as a transcriptional activator and influences cell proliferation, apoptosis, and differentiation [130]. NFIB, located at 9p24.1, is also a transcription factor. The t(6;9)(q22–23;p23–24) results in NFIB fusing with the 5′ portion of MYB and subsequent loss of the 3′ portion of MYB which contains conserved target regions for microRNAs that suppress MYB expression [127, 131]. The result is activation of MYB with overexpression of MYB target genes including BCL2, KIT, CD34, BIRC3, MYC, and MAD1L1 [127]. As many as 14 different variants of the fusion transcript have been identified [127, 132]. Adenoid cystic carcinomas negative for t(6;9)(q22–23;p23–24) have been found to harbor insertions of 9p (including the NFIB gene region) adjacent to the MYB locus with subsequent disruption of MYB regulation [133, 134]. Overall, 80–90 % of adenoid cystic carcinoma cases have MYB activation due to one of these mechanisms [113]. Ninety-seven percent of adenoid cystic carcinomas have been found to overexpress MYB mRNA, and MYB expression is higher in fusion positive tumors as compared to fusion negative tumors [133, 134]. Other tumors display deletions of the terminal region of 6q distal to MYB, particularly 6q24.1–27 [133, 135, 136].
Fusion oncogenes are also associated with less common salivary gland tumors. Mammary analogue secretory carcinoma of the salivary glands is morphologically similar to secretory carcinoma of the breast and is associated with t(12;15)(p13;q25) resulting in a ETV6-NTRK3 gene fusion [137, 138]. The translocation can be useful diagnostically to distinguish this tumor from low-grade cystadenocarcinoma and acinic cell carcinoma [113]. This fusion has been shown in other tumors to result in activation of a tyrosine kinase with downstream activation of the MAPK and PI3K-AKT pathways [139, 140]. Detection of EWSR1-ATF1 gene fusion is a useful diagnostic biomarker for hyalinizing clear cell carcinoma [141]. The t(12;22)(q13;q12) and subsequent EWSR1-ATF1 fusion is identified in >80 % of these rare tumors and has not been reported in histologic mimics such as epithelial-myoepithelial carcinoma, myoepithelial carcinoma, or mucoepidermoid carcinoma [113]. Nuclear protein in testis (NUT)-associated midline carcinomas are rare, poorly differentiated, highly aggressive tumors. These tumors affect the midline structures of the head and neck and can also be found in the mediastinum. There are characterized by rearrangements of the NUTM1 (NUT) gene, located at 15q14. The BRD4-NUT (BRD4-NUTM1) fusion due to t(15;19)(q14;p13.1) is the most common rearrangement [142–144]. BRD4, located at 19p13.12, is a regulator of cell cycle progression. Cribriform adenocarcinoma of minor salivary glands (CAMSG) is a recently described entity [145]. The tumor is associated with rearrangements of ARID1A and PRKD1 [146]. These rearrangements are identified in tumors classic for CAMSG and in cases with features intermediate between CAMSG and pleomorphic low grade adenocarcinoma [146]. Rearrangement of PRKD2 and PRKD3 were also discovered in CAMSG and intermediate cases. Seventy-five percent of classic CAMSG harbored a rearrangement of one of the PRKD genes [146].
Role of Molecular Testing
For all of the conditions described above, the characteristic translocations are useful diagnostic biomarkers. PLAG1 and HMGA2 rearrangements can help in the diagnosis of pleomorphic adenoma whereas detection of MYB rearrangements can aid in the diagnosis of adenoid cystic carcinoma. Detection of these molecular events can be particularly useful in the differential diagnosis of basaloid salivary gland neoplasms on fine needle aspiration (FNA) material. To distinguish adenoid cystic carcinoma from pleomorphic adenoma on FNA material, MYB break apart fluorescence in situ hybridization (FISH) has a sensitivity of 50 %, specificity of 100 %, positive predictive value of 100 %, and negative predictive value of 72 % [147]. The CRTC1-MAML2 fusion features in a proposed classification for mucoepidermoid carcinoma which includes (a) fusion positive tumors with no or few genomic imbalances and a favorable prognosis, (b) fusion positive tumors which are high grade, harbor multiple genomic imbalances, and have unfavorable prognosis, and (c) fusion negative tumors that are high-grade adenocarcinomas with mucoepidermoid-like morphology [148]. The diagnosis of NUT-associated midline carcinoma is defined by the presence of a NUT rearrangement.
The College of American Pathologists cancer protocol for major salivary glands mentions the favorable prognosis associated with the CRTC1-MAML2 translocation in the ancillary testing notes section [149]. While some studies have found that detection of the CRTC1-MAML2 fusion may be indicative of a more favorable prognosis, prospective studies will ultimately be necessary to confirm the association [115, 123, 150]. For adenoid cystic carcinoma, high MYB expression as detected by reverse transcriptase polymerase chain reaction (RT-PCR) has been associated with less favorable prognosis [134].
The role of testing for these translocations as therapeutic targets is emerging. One clinical trial, “A study of drug therapies for salivary gland cancers based on genes”, NCT02069730, is currently recruiting for molecular profiling of salivary gland cancers and administration of targeted interventions based upon molecular results. Trial NCT01587703 is recruiting as a Phase I/II trial for safety and clinical activity of a BRD4 inhibitor (GSK525762) against NUT-associated midline carcinomas. Study criteria include NUT (NUTM1) rearrangement as detected by FISH or NUT positivity as detected by immunohistochemistry (IHC). While not salivary gland specific, trials for TORC1/2 inhibitors in patients with advanced solid tumors unspecified are being conducted [151]. These drugs may be useful in the future against mucoepidermoid carcinomas with CRTC1-MAML2 fusions. In the future, MYB may also emerge as a therapeutic target [130, 152].
When to Test
Testing for fusion oncogenes in salivary gland tumors will be predominantly performed at the time of pathologic examination to aid in the differential diagnosis. There are currently no recommendations or guidelines for testing. Current guidelines for head and neck cancer from the NCCN do not include testing for any of the pathogenic translocations associated with salivary gland tumors [99]. The current cancer protocol for major salivary glands from the College of American Pathologists includes optional reporting of the translocations as ancillary studies [149]. Notes included with the protocol refer to the CRTC1-MAML2 fusion associated with mucoepidermoid carcinoma, the MYB-NFIB fusion associated with adenoid cystic carcinoma, the EWRS1-ATF1 fusion associated with hyalinizing clear cell carcinoma, and the ETV6-NTRK3 fusion associated with mammary analogue secretory carcinoma [149]. The notes in this checklist also comment that detection of the CRTC1–MAML2 fusion in mucoepidermoid carcinoma is associated with more favorable prognosis [149].
Methodology
FISH and RT-PCR are both options for detecting these pathogenic translocations. FISH can be performed on cell suspensions from fresh tissue, touch preps, and FFPE tissue. For salivary gland tumors, it is anticipated that the most common sample types will be FFPE tissues and cell blocks generated from FNA specimens. RT-PCR can also be performed on fresh tissue, cell blocks, and FFPE tissue although it is not always possible to obtain quality RNA from FFPE tissues. Also acceptable for RT-PCR are needle rinses or smears from the FNA procedure. FISH for MYB rearrangements has been successful on air-dried modified Wright-Giemsa-stained slides [147]. In general, Giemsa-stained slides are preferable to pap-stained slides when testing smears previously stained for cytologic evaluation.
FISH testing to detect the pathogenic translocations discussed above involves targeting the DNA of interphase nuclei with fluorescently labeled probes. The most commonly used fluorophores emit in the orange (O) and green (G) spectra. When the orange and green probes are adjacent to one another, a yellow fusion (F) signal is visualized. The probes range in size but may be as large as 200 kb. Probes are commercially available and designed to be complementary to specific chromosomal regions. Briefly, the FISH procedure involves pre-treatment steps, probe hybridization, post-hybridization washing, and enumeration of probe signals per nucleus. If starting with a fresh tissue sample, the cells must first be fixed then pre-treated with ethanol. If FFPE tissue is the starting material, deparaffinization, typically using xylene, is the first step. The tissue is re-hydrated and pre-treatment is then necessary prior to adding a protease solution. After treatment with the protease solution, the tissue is dehydrated using a series of ethanol washes. Probe and hybridization buffer are then applied to pre-treated slides. The probe and nuclear DNA are denatured and then the slides are incubated overnight to allow for hybridization. Post-washes are necessary to clear away unbound probe. 4′,6-diamidino-2-phenylindole, dihydrochloride (DAPI) is then used to counterstain the nuclei. DAPI fluoresces blue. Fluorescent microscopy is then used to visualize the nuclei and enumerate the signals identified in each nucleus using a 100× objective with oil-immersion. Typically 50–200 interphase nuclei are counted per probe set.
Different probe strategies commonly used to detect translocations include break-apart probes, dual color single fusion probes, and dual color dual fusion probes. In break-apart FISH tests, a probe labeled with one color binds the 5′ side of the gene breakpoint and a probe labeled with a different color fluorophore hybridizes to the 3′ side of the gene breakpoint. Break-apart probes are particularly useful for genes with multiple translocation partners. When a translocation involves the gene flanked by the break-apart probes, the signals separate. A normal cell will have two fusion signals (2F) whereas a cell positive for a rearrangement of the targeted gene will reveal a one orange, one green, one fusion (1O1G1F) pattern (Fig. 10.3). With single fusion FISH probe assays, probes target each gene implicated in a characteristic translocation. The probes are labeled with different fluorophores such that the signal pattern in a normal cell is 2O2G (Fig. 10.4a). When the translocation is present in the nucleus, the two probes will be located adjacent to one another resulting in a fusion signal. The abnormal cell will therefore have a 1O1G1F signal pattern (Fig. 10.4b). Since the 1O1G1F signal pattern can result by chance spatial orientation of the genes, the percent of nuclei with this signal pattern necessary to diagnosis a case as positive is typically 10 % or higher. Dual fusion FISH utilizes probes that span the breakpoints of both genes involved in the translocation. The normal pattern is two orange and two green (2O2G) but the abnormal is one orange, one green, and two fusion (1O1G2F) signals (Fig. 10.5). Dual fusion FISH is highly sensitive and specific. Typically <1 % abnormal signal patterns are all that is necessary to interpret a case as positive for the translocation.
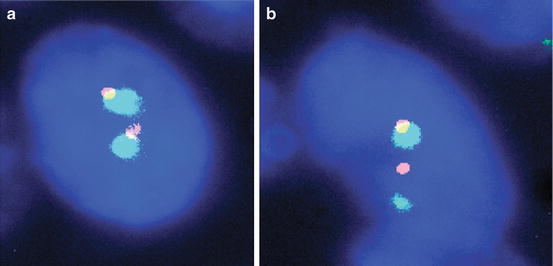
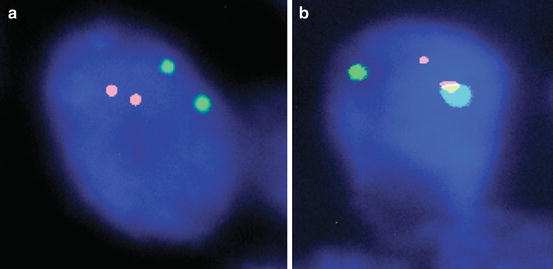
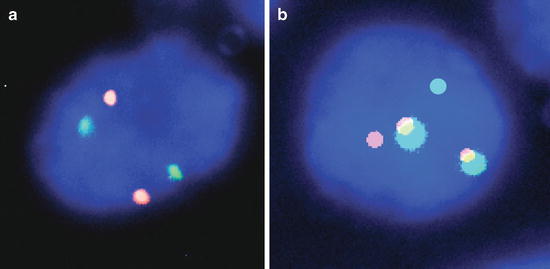
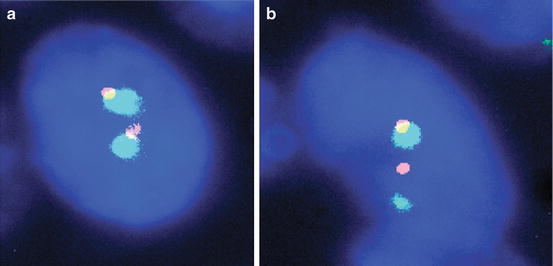
Fig. 10.3
Break-apart FISH. (a) Normal signal pattern (2 F) and (b) abnormal signal pattern (1O1G1F) indicative of a rearrangement, 1,000× magnification
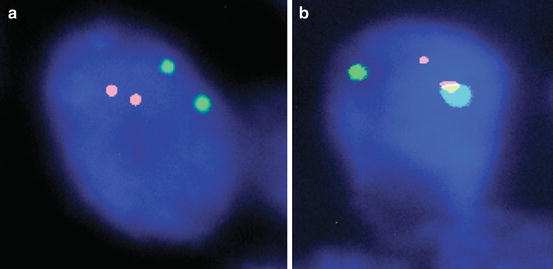
Fig. 10.4
Dual color single fusion FISH. (a) Normal signal pattern (2O2G) and (b) abnormal signal pattern (1O1G1F) consistent with a gene fusion, 1,000× magnification
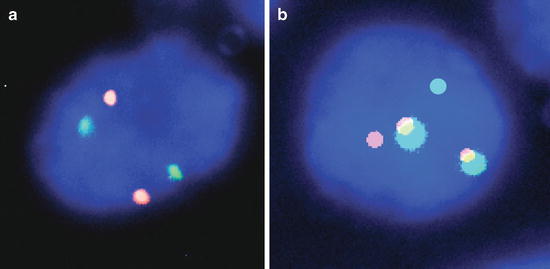
Fig. 10.5
Dual color dual fusion FISH. (a) Normal signal pattern (2O2G) and (b) abnormal signal pattern (1O1G2F) consistent with a gene fusion, 1,000× magnification
Of the genes involved in the pathogenic translocations discussed above, break-apart FISH probes are commercially available for HMGA2, MYB, MAML2, EWSR1, ETV6, and NTRK3 . Of note, break-apart probes for EWSR1 and ETV6 are available as analyte specific reagents. Single color FISH probes are also commercially available for PLAG1, LIFR, CTNNB1, CHCHD7, TCEA1, CRTC1, ATF1, ARID1A, and PRKD1. These probes could be utilized to create laboratory-developed single fusion FISH assays. FISH probes recently became commercially available for the NUT-BRD4 (NUTM1-BRD4) fusion and probes are also available for the MYB-NFIB fusion. Many companies also offer custom probe services.
Advantages of FISH include the variety of sample types that can be tested using the methodology. Viable, dividing cells are not required for testing. Because of the size of the probes, they will typically span many breakpoints within a gene and therefore detect common and uncommon fusion variants. Break-apart probes have a further advantage in that numerous reactions or tests are not required to detect rearrangements of genes with multiple translocation partners. Disadvantages of FISH include the need for fluorescent microscopy and qualified well-trained technologists to score the nuclei. It is a labor intensive procedure that is sensitive to environmental conditions such as humidity and temperature. In ideal circumstances, turnaround times of 3–5 days are achievable, but most laboratories cite turnaround times of 7–10 days. Turnaround time for FFPE tissue is typically on the longer end of that time range given the need to deparaffinize the tissue and pretreat it to break the cross-linking caused from formalin fixation.
Briefly, the RT-PCR procedure involves extraction of RNA from the specimen under conditions that limit exposure to RNases. RNA is reverse transcribed into cDNA. The cDNA is then amplified by PCR. If PCR is done using a real-time PCR instrument, the primers will be fluorescently labeled and detection of PCR products will occur with each PCR cycle. If end-point PCR is being performed, PCR products can be separated by gel or capillary electrophoresis and visualized. Custom designed primers based on those published in the literature for the pathogenic translocations discussed above can be purchased. Advantages of using RT-PCR instead of FISH include the increased analytic sensitivity. Disadvantages of using RT-PCR include the need for quality RNA which is particularly problematic with FFPE tissues. RT-PCR requires trained personnel and equipment not readily available in all laboratories. Workflow is important to limit the potential for contamination. In comparison to a FISH assay, RT-PCR is less desirable if numerous fusion variants exist because several reactions could be required. For example, one study found that nearly half of adenoid cystic carcinomas positive for MYB-NFIB by FISH were negative by RT-PCR due to the high number of fusion variants not targeted by the PCR primers [134].
Quality Assurance
Whether performing FISH or RT-PCR testing, quality assurance procedures similar to other routine laboratory tests need to be implemented. Inclusion of positive and negative controls is necessary and reagent lot-to-lot comparisons must be performed. Proficiency testing must be performed. Monitoring positive rates over time is another quality assurance step that can be used to monitor assay performance. Ensuring specimen adequacy is vital for optimal test performance. Regardless of whether FISH or RT-PCR is used, a stained slide should be evaluated by a pathologist for assessment of percent tumor cellularity and tumor viability.
All sample types accepted for clinical testing need to be included in validation studies. During validation of FISH assays, each laboratory must establish the percent of nuclei with an abnormal signal pattern required to interpret the result as abnormal. This is referred to as the cutoff value. When testing a case, if initial results are at or near the cutoff level, additional nuclei should be enumerated. However, this will be rare in testing salivary gland tumors. Most studies have shown that when a tumor harbors the genetic rearrangement, the majority of nuclei reveal signal patterns consistent with the translocation [115, 120, 147]. Technologists must be properly trained and competency assessments performed. Appropriate training for FISH includes cytologic identification of tumor cells so that normal nuclei are avoided when scoring the probe signals. Scored nuclei should be intact and counting of overlapping nuclei is to be avoided. Split signals are a potential pitfall when counting signal patterns and training should include an explanation of how to distinguish split signals from two distinct signals. A nucleus with no visible signals should not be counted unless surrounded by nuclei with visible signals. In general, if more than 2 % of the counted nuclei have zero signals, hybridization failure should be suspected and the case repeated. The use of two technologists to manually score a case is another quality assurance method labs can take. Technologist counts should not differ by more than 5 % from each other for any observed signal pattern.
Interpretation of Results
Results, for both FISH and RT-PCR assays, will be reported as either positive or negative for the rearrangement being tested. If a FISH break-apart probe is being used and the result is positive for a gene arrangement, the translocation partner will not be identified. For example, results will be reported as positive or negative for a MAML2 rearrangement. This result does not specify if the translocation partner is CRTC1 or CRTC3. If a dual color single fusion or dual color dual fusion probe strategy is being used, the result will be reported as positive or negative for the specific translocation being targeted. For example, if a fusion probe strategy is being utilized to target the MYB and NFIB genes, the result can be reported as positive or negative for the t(6;9)(q22;p24) (MYB-NFIB) fusion. RT-PCR results will be reported as positive or negative for the specific fusion transcript(s) targeted by the assay.
Results should always be interpreted in conjunction with the clinical presentation and histomorphologic findings. Knowledge of tumor cellularity is also important to the interpretation. Cases with a low percentage tumor cellularity could be falsely negative. Negative results should be interpreted with the knowledge that the targeted gene rearrangement could still be present in the tumor but is below the lower limit of detection of the assay. Negative results also do not exclude the possibility of a genetic rearrangement other than the specific rearrangement targeted by the assay. This is particularly important to remember when using RT-PCR to only detect common fusion transcripts. Results must also be interpreted with the knowledge that cutoff values can differ for FFPE tissue versus fresh samples. Nuclear truncation resulting from the cutting of FFPE sections is the primary reason for differing cutoffs.
Reporting Using Standard Nomenclature
When reporting results, correct gene names must be used. Synonyms or colloquial names can be provided in parentheses. Gene names should be italicized. Although it is not required, FISH results should be reported using ISCN approved nomenclature [153].
ERBB2 (HER2) Amplification in Salivary Duct Carcinoma
Background
Salivary duct carcinoma (SDC) is a high-grade aggressive neoplasm that most often affects men older than 50 years of age [155–157]. The tumor can occur as a primary neoplasm or as a component of carcinoma ex pleomorphic adenoma [157]. The parotid gland is the most common location [156, 157]. Histologically, SDC resembles high-grade ductal carcinoma of the breast. This tumor accounts for up to 2 % of primary salivary gland epithelial neoplasms [155]. SDC is fast growing, locally recurs, and is capable of metastasizing [156, 157]. The 5-year survival rate is <40 % [156, 157].
Similar to its counterpart in the breast, overexpression of ERBB2 (also known as HER2, HER-2, HER-2/neu) has been found in SDC [82, 158–163]. The ERBB2 gene is located at 17q12 and encodes a receptor tyrosine kinase. Ligand binding leads to dimerization of the receptor, autophosphorylation, activation of signaling cascades, and cell proliferation [164, 165]. Thus, ERBB2 is involved in cell growth, adhesion, and migration as well as differentiation and survival [166]. The percentage of cases positive for ERBB2 overexpression varies depending on methodology used and the definition of positivity. When 2007 ASCO/CAP scoring criteria are applied, 15–40 % of SDC are positive for ERBB2 amplification [155, 161, 163, 167]. Discordance between FISH and IHC exists and only about half of the cases considered positive by IHC harbor gene amplification [159, 163, 168]. A more recent study, however, found 13 of 14 positive IHC cases to also be FISH amplified [169].
Role of Molecular Testing
Overexpression of ERBB2 in SDC may be predictive of response to ERBB2 (HER2) inhibitors. Trastuzumab is a murine monoclonal antibody against ERBB2 [170]. Case reports or small series have shown that trastuzumab in combination with chemotherapy can stabilize disease and even prolong disease free survival [168, 171–175]. Patients selected for trastuzumab therapy in these cases were positive by either IHC or FISH. Patients have also responded to trastuzumab when used in the palliative setting [176]. A single case report has also demonstrated the ability of a trastuzumab, lapatinib, and bevacizumab (a monoclonal antibody targeting VEGF) to stabilize metastatic disease [177]. Lapatinib is a small molecule tyrosine kinase inhibitor of EGFR and ERBB2 and in breast cancer cells has been shown to work synergistically with trastuzumab [178–180]. A Phase II clinical trial investigating trastuzumab in advanced salivary gland tumors closed early due to the low numbers of screened tumors found to be 2+ or 3+ for ERBB2 (HER2) by IHC [181]. The study which did not specifically identify SDC among the salivary gland tumors concluded that trastuzumab had low activity against salivary gland tumors positive for ERBB2 (HER2) expression [181]. Stabilization of disease was obtained in a Phase II study of lapatinib in salivary gland tumors [182]. However, the study only included four salivary duct carcinomas, and the response in these cases was not specifically separated from the non-adenoid cystic carcinoma group [182]. One clinical trial, “A study of drug therapies for salivary gland cancers based on genes”, NCT02069730, is currently recruiting for molecular profiling of salivary gland cancers and administration of targeted interventions, including ERBB2 inhibitors, based upon molecular results.
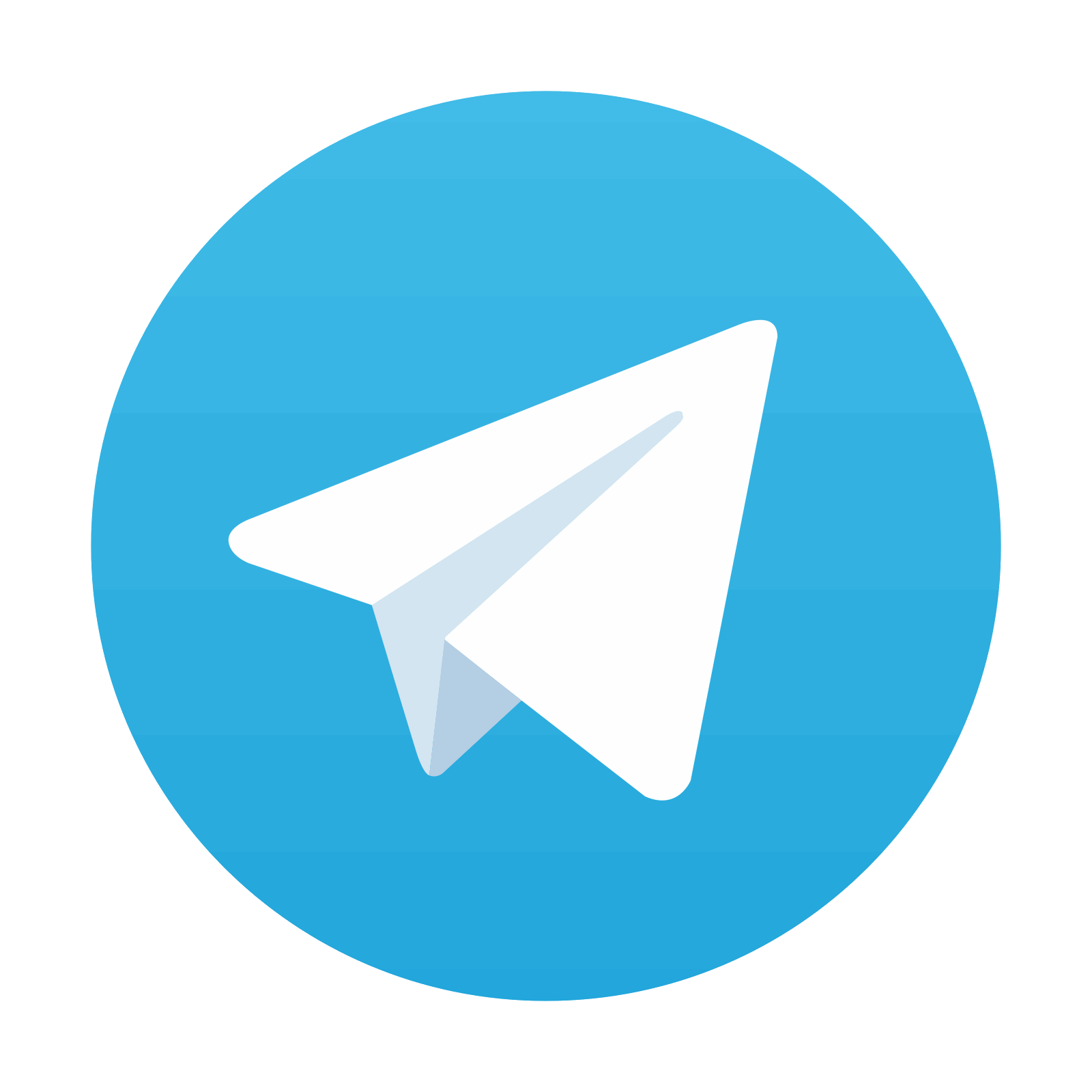
Stay updated, free articles. Join our Telegram channel

Full access? Get Clinical Tree
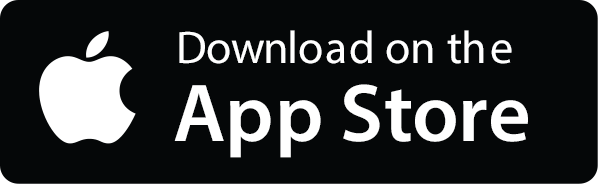
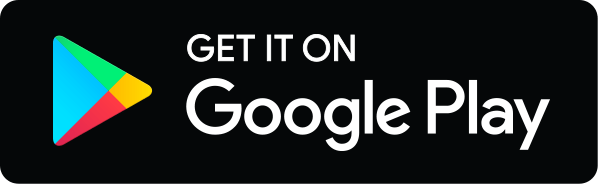