In Pennsylvania, the incidence of moderate-to-severe TBI in those aged 65–90 increased by 87 % between 1992 and 2009 [28]. On a global scale, it has been posited that TBI will be the third leading cause of death and disability by 2020 [29, 30]. Given the increasing trend of TBI in the growing geriatric population, adequate knowledge of the mechanism of injury and associated outcomes becomes increasingly important for physicians.
Although the hospital course varies with severity of injury and associated comorbidities, the in-hospital mortality rate or discharge to a residential home correlates positively with age [4]. Indeed the literature almost universally shows an increasing likelihood of poor outcome with increasing age. Jennett showed that less than 5 % of patients greater than 65 years of age who remain in coma for greater than 6 h had a good outcome or recovered to moderate disability [31].
Some studies have found a higher incidence of TBI in older females which opposes the trend seen in younger populations in which males more frequently present with TBI [9, 32]. Mechanism of injury is an additional difference found in older patient populations; falls are the most common reason for TBI in geriatric patients [4, 8–10, 28, 33, 34]. After falling, the most common brain injury is intracranial hemorrhage, especially chronic subdural hematoma (CSDH). Alcohol abuse is increasingly seen in this population and obviously predisposes to falls [35]. Other disorders predisposing to falls include increased frailty in bone and musculature [36–40] and comorbid conditions such as diabetes [41].
Pathophysiology of the Aging Brain
Following a traumatic brain injury, damage occurs in two phases, primary and secondary. Primary injury refers to the initial insult to the brain resulting in immediate cellular perturbation or death. This results in neurological deficits dependent upon the type of injury and specific location within the brain as well as vascular damage which results in immediate hemorrhage. Secondary injury is a consequence of primary injury and includes changes in the microenvironment, altered cellular metabolism, diminished vascular response, hypoxia, edema, and inflammation. In the context of a geriatric patient, these mechanisms may be enhanced due to the normal physiologic changes that occur with increased age [42].
Primary Injury
Neuronal change and glial cell loss are expected occurrences in the aging brain. Beginning in the fifth decade of life, normal brains begin to undergo some degree of degeneration [43, 44]. Nerve cells mainly experience death secondary to Wallerian degeneration, apoptosis, or decreased efficiency due to diminished synaptic potential [45]. Consequently, aging reduces plasticity and repair mechanisms [9, 43, 46, 47]. Although the natural reduction in cell number and plasticity has been studied in the context of diminished memory [48, 49], plasticity plays an important role following traumatic brain injury. For instance, Yager et al. showed decreased plasticity measured by functional outcome following a cerebrovascular accident in immature versus mature mice [47].
Evidence from animal studies suggests marked differences in the morphology, number, and/or organization in the supportive cells of the central nervous system. Astrocytes appear to undergo hypertrophy with advanced age without increasing in number [50–56]. Studies indicating increased staining with glial fibrillary acidic protein (GFAP) support this hypothesis [57, 58]. Additionally, the gap junctions by which astrocytes communicate and maintain the blood–brain barrier appear to undergo reorganization. The changes in microglial cells during the normal aging process are not fully understood. Some studies report an increased phagocytic activity of these protective cells. There is conflicting evidence as to whether these cells proliferate, particularly in female versus male. Further studies suggest that microglia undergo “dystrophy,” in which morphological changes occur due to the increased age of the cells. The third type of neuroglial cell, oligodendrocytes appear to undergo both proliferation and reorganization of cellular alignment [53, 59–61]. Evidence of remyelination of nerve fibers in the CNS supports the need for increased numbers of oligodendrocytes with increasing age [62]. Although little is known about the fourth type of neuroglial cell, which stains to NG2 antibodies, these cells have been suggested to be precursors to oligodendrocytes and their proliferation during the aging process [63, 64]. Demyelination in the aging brain is thought to occur due to the degenerative processes that have been studied in animals and a consequential nerve fiber loss quantified in humans. Studies have shown that changes in myelin include dense cytoplasmic accumulations, areas of myelin ballooning, and changes in the lipid composition [65–69]. As a result of myelin degeneration, studies have shown that white matter loss also occurs during aging. Additional imaging studies support the loss of white matter in the normal aging brain [45].
Traumatic brain injury in the elderly contributes to the cellular loss of the natural aging process and may further enhance any pathological diseases in these patients. Immediate cell death in the brain following traumatic brain injury is dependent upon the type of injury. For example, a penetrating injury or localized impact initially causes focal damage, whereas a rotational head injury or greater impact may cause diffuse brain injury. When mechanical damage occurs, it has been shown that axonal damage can be primary from direct injury or secondary from death following initial swelling [70]. In either case, axonal injury leads to cell death and eventually to coma and increased mortality [71].
Another main mechanism of primary injury in TBI results from direct damage to blood vessels in the context of naturally changing and weaker vasculature. Geriatric patients are more susceptible to immediate vascular damage. Due to the decreased brain volume associated with age and decreased neuronal density, the brains of elderly patients allow for more space in which the subdural veins are located [34, 72, 73]. As such, they are less protected and more prone to rupture causing a subdural hematoma. Additionally, the inherent strength of the cellular composition of blood vessels with age is diminished increasing the incidence of leakage and rupture following less intense mechanical disruptions that might cause hemorrhage in younger patients [73].
Another important aspect of vascular changes with increased age is a natural decrease in cerebral blood flow (CBF) and altered vascular reactivity [74–77]. Studies suggest that decreased intracranial blood flow with age is associated with arteriolar rarefaction with conflicting evidence regarding capillary density [76, 78]. A combination of factors is most likely responsible from hormonal to structural changes of the vessels; in addition, changes in blood flow decrease metabolism and affect signaling, neuronal plasticity, and neurogenesis [77].
Decreased CBF with age and following injury leads to hypoxia and further damage. A study in rats following fluid percussion injury showed decreased heart rate in aged subjects while increased heart rate in younger rats. The aged rats showed significantly increased damage using histopathology and mortality endpoint [79]. Czosnyka et al. studied whether a failure of cerebral autoregulation might contribute to poor outcomes in the elderly [80]. Measures of autoregulation and pressure reactivity worsened with age. Older patients were found to have worse outcomes after brain trauma than younger patients despite having higher initial GCS scores. Interestingly, the intracranial pressure was lower and the cerebral perfusion pressure higher in the elderly patients, which typically are associated with improved outcomes [80, 81].
Secondary Injury
Mechanical perturbation from direct injury results in the release of ions, toxins, and neurotransmitters. Damaged cells experience metabolic changes, which contribute to the toxic metabolites in the microenvironment of the injured brain tissue. Both mechanisms further contribute to secondary cellular loss and subsequent injury mechanisms such as edema and inflammation in the aging brain.
One of the first ions to be released following increased membrane permeability or cell death is intracellular calcium [82]. The significant calcium release sets in motion a prolonged apoptotic and immediate necrotic pathways via caspase and calpain proteases, respectively [83–85]. In aged animals, there is significantly altered calcium regulation resulting in prolonged after hyperpolarization of cells, both of which may exacerbate the apoptotic process.
Glutamate is the main excitatory neurotransmitter in the central nervous system as well as the main neurotransmitter responsible for increasing damage following TBI in geriatric patients [83, 86, 87]. Hamm et al. suggests this effect is particularly harmful in an aged brain due to the increased density of receptors with age due to a natural loss of neurons [79]. Lactate is also released into the microenvironment and increasingly taken up by the brain following traumatic injury [88–100]. The increased concentrations of lactate can lead to edema via breakdown of the blood–brain barrier or ischemia [88, 101–104]. As previously noted, the blood–brain barrier is naturally compromised in the elderly brain, further exacerbating this issue.
Numerous studies have concluded that the formation of free radicals contributes to secondary cell damage and death following TBI [83, 105, 106]. The pertinent free radicals in this context include superoxide anion (O2), nitric oxide (NO), and peroxynitrite (ONOO). These substances are formed immediately following the rise of extracellular calcium ions [106] as well as in response to altered neuronal metabolism, vascular changes, and the other mechanisms of secondary injury following TBI [105, 107]. Free radicals increase the damage following TBI by interfering with vasodilatation [108], subcellular components, and particularly mitochondria [109, 110] in addition to creating oxidative stress [111].
Another mechanism of secondary injury following TBI are the resulting inflammation and edema of brain tissue [112]. In addition to hemorrhage, inflammation and edema contribute to increased intracranial pressure (ICP). The decreased ability of the aged brain to compensate for the changes in volume may be another reason for the increased mortality and morbidity in this patient population. Evidence suggests inflammation results in release of pro-inflammatory cytokines and mediators [113, 114]. Onyszchuk et al. reported the edema that develops in the aged rats requires a longer time to subside and affects a larger area of the brain [115].
Lastly, studies have reported the formation of thrombin and plasmin following TBI [116, 117]. These coagulation mediators become particularly important in a patient population that is frequently prescribed anticoagulation therapy for atrial fibrillation, ischemic heart disease, and peripheral vascular disease. One study showed the injection of the thrombin into the caudate of mice correlated with increased infiltration of inflammatory cells, angiogenesis, and reactive gliosis [118].
Medical Management of Geriatric TBI
While the medical management/neurocritical care of TBI has been codified through the Guidelines for the Management of Severe TBI for greater than 10 years, their application to geriatric patients remains unclear.
After several iterations, there remain only four class I evidence-based recommendations [119]:
1.
Avoidance of hyperventilation
2.
Utilization of anticonvulsants in the first week after injury
3.
Avoidance/correction of hypoxia and hypotension
4.
Avoidance of the use of steroids for treatment of the TBI
While the general principles put forth in the guidelines would seem to hold true for any adult patient of any age, there are some areas which might require special consideration given the unique pathophysiology of the aging brain.
As previously described, there is evidence that autoregulation worsens with age. The impact of hyperventilation in the elderly might be attenuated with respect to decreasing cerebral blood flow [81]. However, there is also evidence that CBF naturally decreases with age [74, 75, 77, 84]. Thus, avoidance of hypoperfusion from hyperventilation and maintenance of adequate fluid resuscitation would appear to be an extremely important component of geriatric neurocritical care of TBI.
While not specifically studied in elderly patients, the avoidance/minimization of hypoxia or hypotension is particularly important. The blood pressure issue requires consideration of the fact that many geriatric patients have longstanding hypertension. Thus, they may require a higher blood pressure to maintain adequate cerebral blood flow and oxygenation as a result of vascular noncompliance. It thus may be reasonable to allow blood pressure to remain higher than normal or if known the same as that of the pre-injury status in elderly patients.
In general, ICP issues are not of paramount importance in elderly patients unless there is an associated significant intracranial hemorrhage, which may or may not be treated surgically. Given the overall reduced brain volume and the high incidence of low velocity injuries from falls, the likelihood of diffuse axonal injury is quite low; the occurrence of significant brain swelling is minimal, and thus the need for aggressive and escalating ICP control is rare.
While mannitol for the control of ICP has the highest level of evidence in the guidelines, there may be an advantage for hypertonic saline in elderly patients. As previously described, there is a reorganization of the astrocytic gap junctions with a resultant “opening” of the blood–brain barrier with normal aging [57, 58, 120–124]. This lack of normal “tightness” in the BBB in elderly patients may allow for greater egress of mannitol into the extracellular space potentially exacerbating cerebral edema and ICP.
While there are no randomized controlled clinical trials regarding the use of hypertonic saline in this setting, it has become a generally accepted treatment principle across all age groups. In addition, hypertonic saline may assist in overall fluid management, as fluid overload in the elderly patient is a frequent and significant issue. In this population, it is recommended that heart ejection fraction be established to guide fluid management. In general, 3 cc/kg of hypertonic saline provides the same level of volume expansion as 10 cc/kg of other resuscitative fluids.
As elderly patients for the most part have been excluded from the phase III neuroprotective clinical trials that took place in the 1990s and are now reemerging, it is unknown whether any of the previous or current agents might be more beneficial in geriatric patients than they were in the younger patients.
Recent information, however, has become available that statins may improve outcomes in geriatric TBI [125–129]. Statins are common medications used to treat hyperlipidemia in elderly patients with or without comorbid cardiovascular disease. Statin use in patients without concomitant cardiovascular disease showed a decreased risk in incidence of in-hospital mortality as well as improved 12-month functional outcome in geriatric patients with TBI [125]. It is important to note that these patients were already being treated with statins, and there is no indication that post TBI use of statins might have a similar effect [130].
Surgical Management of Geriatric TBI
While guidelines have been developed for the surgical management of traumatic brain injury, there is no class I evidence and all “guidelines” are put forward as “recommendations” [119]. The recommendations for surgical management of acute subdural hematoma are as follows: “In acute SDH with a thickness greater than 10 mm and/or midline shift greater than 5 mm on computed tomographic (CT) scan should be surgically evacuated regardless of the patient’s Glasgow coma score (GCS)” [119].
One would expect that such a recommendation needs to be tempered in elderly patients. The surgical literature is quite contradictory in this regard. Lau et al. recently posed the following question on craniotomy for hematoma in elderly patients: “do elder patients continue to suffer higher morbidity and mortality than younger patients.” One-hundred and three patients (27 older than 80; 76 younger than 80) were studied. Age was not associated with any significant difference in return to baseline, 30-day mortality or postoperative ICU length of stay. However, there was no stratification of age versus GCS score which was 13–15 in almost 50 % of patients [131]. In stark contradistinction, Cagetti et al. in a retrospective study of 28 patients aged 80–100 demonstrated an 88 % mortality rate. All 19 patients with a GCS less than 9 died [132]. It must be acknowledged that the Cagetti paper is 20 years old and since that time neurocritical care has significantly improved. Nonetheless, the majority of the literature on this subject points to an unfortunately dismal prognosis in the setting of severe TBI associated with intracranial hemorrhage in patients older than 65 years.
However, the majority of posttraumatic intracranial hematomas in the elderly are chronic subdural hematomas (CSDH). The incidence of CSDH increases from 1.72 per 100,000 per year in those less than 70 years old to 7.35 per 100,000 per year in those greater than 70 [133]. These patients may present solely with headache and subtle mental status changes. Less than 10 % present with significant neurological symptoms.
CSDH has generally been felt to be a benign lesion. While there are a variety of treatment methodologies, the literature has uniformly shown good outcomes from both medical and surgical treatment. The overall in-hospital mortality ranges from less than 5 % to approximately 16 % [130].
Recent literature, however, indicates that CSDH is not necessarily a “benign” disease/injury. Miranda et al. recently studied the 6-month and 1-year outcomes of 209 patients treated surgically for CSDH in patients 65–96 years of age [134]. Mortality rates were 26.3 % at 6 months and 32 % at 12 months. The follow-up period extended for up to 8.3 years (median 1.45 years). Comparison of survival with anticipated actuarial survival demonstrated a twofold increase in mortality in those patients who had suffered a CSDH. Similar outcomes have been found in long-term follow-up after hip fracture [135–137]. Miranda postulates that CSDH “unmasks” underlying medical conditions and exacerbates them [134].
Obviously, the decision/recommendation to operate or not operate cannot be based on guidelines or prior nonclass I evidence. Increasingly, patients are providing more and more specific living will and potential end-of-life instructions which must be respected. Nevertheless, families will look to the neurosurgeon to guide final decisions. (See section “Palliative Care”.)
Outcomes
Although overwhelming evidence suggests poor outcome with increased age following TBI, there is discord about whether this trend is stepwise with an age threshold beyond which outcome is significantly worse versus continuously increasing poor outcomes with increasing age. A number of reports provide evidence showing increased mortality as well as a 4–6 fold higher probability for unfavorable outcome [8, 9, 80, 81, 132, 138, 139]. Thomas et al. retrospectively showed hospital and death rates after nonfatal TBI to increase with the near-geriatric age group in both men and women [140]. Diminished functioning in all areas – cognitive, motor, and memory – has also been reported [9, 141].
Many studies have been conducted to investigate risk factors for worse outcomes following TBI specific to the geriatric population. It has been shown that gender plays a role; females typically have better outcomes [142–145] although this has been contended [146]. This suggests a possible role of estrogen or progesterone in the reparation process following a TBI. The ProTECT trial found that the patients that received progesterone had a lower 30-day mortality rate that those that received placebo [147]. Moderate traumatic brain injury survivors who received progesterone were more likely to have a moderate-to-good outcome than those randomized to placebo. The specific type of injury has been shown to be another factor. Improved outcomes with progesterone have been shown in other randomized controlled trials [148, 149].
The mainstay of understanding the pathophysiology and risk factors for worse outcomes in the geriatric population rests in potential optimization of treatment and prevention of TBI. Researchers have identified varied reasons for the worse outcomes in these patients. For instance, older patients do not receive the same intensity of care as younger patients [150]. There is a delay in getting geriatric patients to neurosurgical intervention when compared to younger patients with TBI [14, 150]. This suggests that improvements in timing and logistical care within hospitals may be the first step toward improving outcomes.
Prevention targets the patient before they arrive at the hospital. Given that the most common reason for TBI in these patients is falls, it is imperative to work toward the prevention of falls. One way in which this might be possible is to monitor medications that may contribute to falls [151]. Another way is to emphasize bone health and exercise. If patients have appropriate bone density, fracture might be prevented thus preventing falls. Additionally, if a patient does fall, an improved muscular response may prevent head impact or injury.
Special Considerations
Unfortunately, the care in this patient population is unique given their medications and comorbidities at the time of brain injury. This complicated medical picture requires more research to improve knowledge regarding how common medications and pathological processes in elderly patients may affect the treatment needed following TBI.
A particular challenge in management is anticoagulation. A significant number of patients greater than 65 years of age are on some sort of anticoagulation or antiplatelet medication given the well-known vascular processes that predispose older patients to cardiovascular disease. However, the data on the effect of this therapy in TBI is very conflicting. Some studies have shown that anticoagulation therapy has a significant effect on outcome. One study showed increased mortality in those on warfarin (trade name Coumadin) who fall from standing [152]. In a retrospective study of 384 patients 55 years or older, warfarin use before a closed head injury was associated with more serious injuries and increased mortality [153]. Yet, other studies have not been able to show a significant effect of anticoagulation therapy on outcome. Fortuna et al. found no significant effect of clopidogrel, aspirin, or warfarin on outcome in those with blunt head trauma [154]. Another study analyzed 3 age groups within the geriatric population without taking into account mechanism of head injury with nonsignificant CT findings between pre-injury warfarin use and worsened outcome [155].
Pieracci et al. further evaluated this question by retrospectively grouping the geriatric patients with TBI based on warfarin use with INR greater or less than 2 and those who did not take warfarin [156]. The group with an INR greater than 2 showed significant likelihood for GCS below 13 and increased mortality. In those with an INR less than 2 and those who were on no pre-injury anticoagulation, the difference in mortality rates was not significant [156]. This study suggests that TBI might be affected not merely by anticoagulation therapy but more so by the degree of anticoagulation at the time of injury.
Additional studies used this data to evaluate the effect of anticoagulation on current therapy in elderly patients.
Some have suggested repeating normal CT scans in patients with GCS of 14 or 15 and supratherapeutic INRs within 6 h after presentation whereas others suggest emergency CT is not necessary in those with GCS of 15 irrespective of coagulation status [157, 158].
Recently, direct thrombin inhibitors have been introduced in the United States. These anticoagulants exert their effect at the end of the coagulation cascade. Thus, there is currently no method of reversal. Serum half-life of these drugs is 12–17 h. Their level can be monitored by checking a thrombin time.
The Re-Ly trial showed that low doses of the direct thrombin inhibitor dabigatran (trade name Pradaxa) were associated with less risk of ICH when compared with warfarin while high doses had a similar risk [159]. However, this was not a head injury study.
A recent case report demonstrated fatal progressive bilateral ICH over the course of 6 h in an 83-year-old after a ground level fall and a GCS of 15 on admission [160]. These authors suggested that hemodialysis be used to clear the drug as factor VIIa and fresh frozen plasma are ineffective in reversing the effects of the dabigatran; however, only approximately 35–60 % of the drug can be removed within 23 h.
Since there is little purpose served by supratherapeutic anticoagulation, there is little argument that patients who present with TBI even with good GCS and normal CT scans should have their warfarin “reversed” to levels of normal anticoagulation for their medical condition.
When it is otherwise felt best to reverse the patient’s anticoagulation with warfarin, the first step is the use of intravenous or intramuscular vitamin K. However, this is rarely sufficient to accomplish a normalization of INR, and fresh frozen plasma has been the mainstay of therapeutic reversal. While fresh frozen plasma is quite effective in this regard, in the elderly population, one has to be particularly vigilant for volume overload. The issue of volume overload may be addressed by the use of Prothrombin Complex Concentrates (PCC). While there is no literature to support its use, in desperate situations, recombinant factor VIIa may be brought into play.
The primary reversal agent for the platelet inhibitors is the administration of platelets. However, because of the half-life of these drugs, it is frequently necessary to administer platelets over a prolonged period of time. DDAVP has shown some promise in effecting more rapid normalization of platelet function.
As noted previously, at this time, there is no means or mechanism of reversing the effects of the direct thrombin inhibitors (Table 23.1).
Table 23.1
Commonly used anticoagulants, their brand names, mechanism of action, and reversal agents
Brand name | Mechanism of action | Reversal agents | |
---|---|---|---|
Warfarin | Coumadin | Vitamin K antagonist (inhibits factors II, VII, IX, and X) | Fresh frozen plasma (FFP), vitamin K, prothrombin complex concentrate (PCC) |
Aspirin | Antiplatelet: thromboxane inhibitor | DDAVP, platelets may give temporary reversal | |
Clopidogrel | Plavix | Antiplatelet: ADP receptor/P2Y12 inhibitors | |
Prasugrel | Effient | ||
Ticlopidine | Ticlid | ||
Abciximab | ReoPro | Antiplatelet: glycoprotein IIb/IIIa inhibitors | |
Eptifibatide | Integrilin | ||
Tirofiban | Aggrastat | ||
Fondaparinux | Arixtra | Heparin group/glycosaminoglycans/(binds antithrombin) | Protamine sulfate (not as effective as with heparin) |
Enoxaparin | Lovenox | ||
Dabigatran | Pradaxa | Direct thrombin (II) inhibitor | None known |
Aside from anticoagulation, the most common comorbid conditions include diabetes, hypertension, cardiac arrhythmias, chronic pulmonary disease, congestive heart failure, and electrolyte disorders.
Palliative Care
The issue of palliative care in the neurotrauma patient is of increasing importance – especially with the ever-increasing geriatric population.
Obviously, the neurosurgeon’s first priority is to “do no harm.” However, this has been liberally interpreted as not needlessly prolonging suffering – even in a comatose patient – when moral responsibility dictates otherwise.
Thus, it is incumbent upon the neurosurgeon and trauma surgeon to keep abreast of the ever-increasing literature on geriatric neurotrauma to be in the optimal position to answer the very frequent question of “what would you do if this were your family member.”
While neurosurgical technical advances in neurocritical care have in many cases been lifesaving, at the same time it has brought forth conundrums in initiating and withholding care. Heifitz focuses on the concept of beneficence and justice – “the equal treatment of all and privacy or the freedom to make a choice of what is not wanted.”
Decisions are typically made on ranking values and principles. A physician must guide patients and families through these difficult decisions while at the same time taking care not to make decisions for the patient or family based on the physician’s moral and ethical beliefs.
Decisions on not initiating or withholding care should be considered in the context of prognosis, poor quality of life before the TBI or poor quality of life anticipated after TBI.
As previously noted, today about 15 % of the population are over 65, while by 2030 this would double. Unfortunately many live with a limited quality. Two million are in nursing homes. While at some point in their lives over 65 years, seven million will spend some time in a nursing home with 80 % having mental impairment.
These societal issues cannot be overlooked in end-of-life TBI decisions.
References
1.
Gorman M. Development and the rights of older people. In: Randel J et al., editors. The ageing and development report: poverty, independence and the world’s older people. London: Earthscan Publications, Ltd; 1999. p. 3–21.
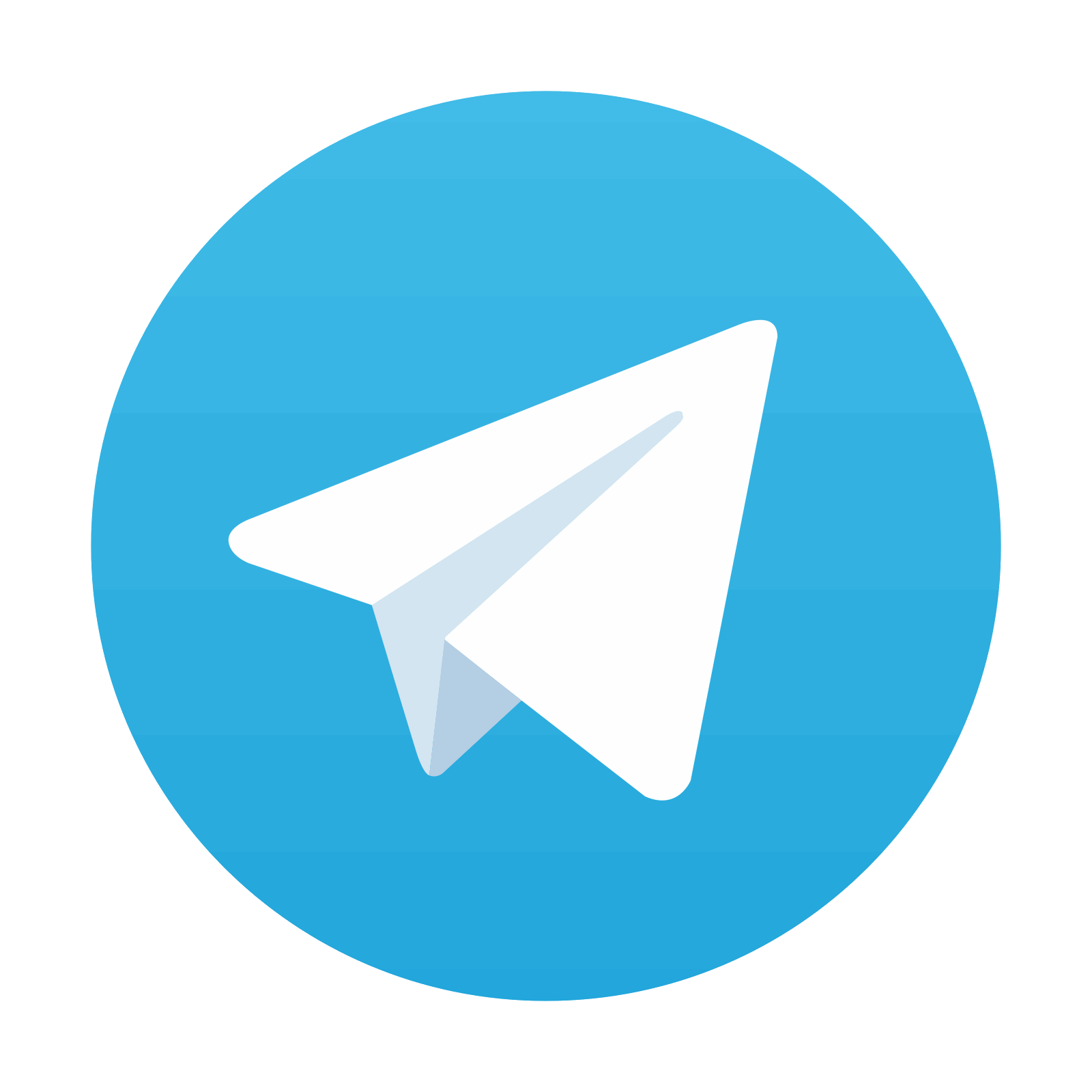
Stay updated, free articles. Join our Telegram channel

Full access? Get Clinical Tree
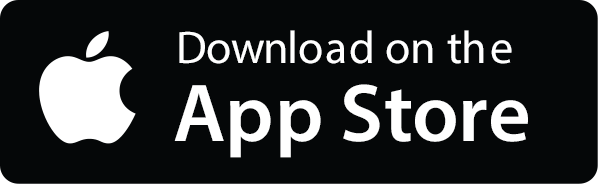
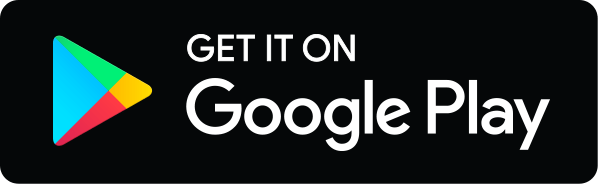