© International Society of Gynecological Endocrinology 2016
Andrea R. Genazzani and Basil C. Tarlatzis (eds.)Frontiers in Gynecological EndocrinologyISGE Series10.1007/978-3-319-23865-4_33. Genomics and Ovarian Response
(1)
1st Department of Obstetrics/Gynecology, School of Medicine, Aristotle University of Thessaloniki, Thessaloniki, Greece
3.1 Introduction
Ovarian stimulation constitutes an integral part of fertility treatment with the different assisted reproduction techniques (ART). As documented in other areas of medicine, patients’ response to medication depends on their genomic profile. Hence, in order to understand the variations in ovarian response to stimulation between different women, it is necessary to examine the genomics of ovarian and endometrial function. This will allow to predict more accurately low, normal, or high responders and adopt the stimulation protocol accordingly.
The aim of this chapter is to review the involvement of different genes in follicular, oocyte and endometrial development, and their relevance to ovarian stimulation.
3.2 Follicular Development and Angiogenesis
In humans and large mammals, the follicles grow after antrum formation until they become gonadotropin dependent and enter a phase of rapid terminal development [1]. Gonadotropin dependence is acquired at a given follicular diameter. Below this diameter, the small antral follicles constitute a pool of gonadotropin-responsive follicles, which is the reserve for ovulation. This is a dynamic reserve, since it is emptied by the entry of follicles in the follicular waves of terminal development mediated by the follicle-stimulating hormone (FSH) gene expression and renewed by the continuous growth of smaller follicles.
3.3 FSH, LH, and Their Receptors
In the female reproductive system, angiogenesis is a process essential for normal tissue growth and plays a crucial role in follicular growth and the selection of the ovulatory follicle [2]. In the ovary, blood vessel formation facilitates the delivery of many substances, including oxygen, nutrients, and FSH to follicles. A capillary network around each follicle is necessary for follicles to grow beyond the secondary stage, which contains multiple layers of granulosa and theca cells. As the follicle develops, endothelial cells are recruited to the theca cell layer at the adjacent ovarian stroma. Endothelial cell proliferation is maintained in healthy tertiary follicles to support the expansion of the theca vasculature. In contrast, follicular atresia is associated with inadequate development and regression of the theca vasculature. Increased vascularity is a determining factor in the establishment of follicular dominance. Consequently, angiogenic factors are of increasing interest in ovarian physiology.
FSH and luteinizing hormone (LH) gene products are pituitary glycoproteins essential for normal gonadal function [3]. They regulate gonadal growth, differentiation, endocrine function, and gametogenesis. The effects of FSH and LH are mediated through binding to specific cell surface receptors, FSHR, and LHR, respectively. The presence of FSHR and LHR mRNA in denuded oocytes and preimplantation embryos from zygotes to blastocysts has been demonstrated, indicating a possible role for gonadotropins in the resumption of meiosis and early embryonic development.
FSHR and LHR are G-protein-coupled receptors, which span the plasma membrane seven times and transduce the biological action of FSH and LH, using cyclic AMP (cAMP) as the main intracellular second messenger [3]. The FSHR gene contains a single large exon, which encodes the transmembrane and intracellular domains, and nine smaller exons, which encode the extracellular domain.
Ovarian response to FSH stimulation depends on the FSH genotype. Factors proposed to affect ovarian response to FSH are the distribution of FSH isoforms and single nucleotide polymorphisms [3]. The role of two distinct FSHR variants, Thr307/Asn680 and Ala307/Ser680, in ovarian response to FSH in women undergoing controlled ovulation induction has been investigated by Loutradis et al. [3], who examined the prevalence of Ser680Asn polymorphisms of the FSHR gene. According to the results, good responders carry more often the Asn/Ser genotype. This finding may reflect a better and more rapid ovarian response to exogenous stimulation, possibly due to a more efficient FSHR. The Ser/Ser variant might be related to higher serum FSH levels, while the Asn/Ser, with lower serum FSH levels. However, Mohiyiddeen et al. [4] demonstrated that FSHR genotype does not predict metaphase II oocyte output or fertilization rates in ICSI patients.
Signaling mediated by the LHR gene expression is important for patients’ response to exogenous gonadotropins (i.e., hCG) administered during controlled ovarian hyperstimulation (COH), and inter-individual variability in LHR activity could significantly impact the outcome [5]. O’Brien et al. [5] found that insLQ polymorphism (rs4539842) is not associated with patients’ response to COH and it is not a predictor for ovarian hyperstimulation syndrome (OHSS). However, they associated the luteinizing hormone/chorionic gonadotropin receptor (LHCGR) rs4073366 polymorphism with the OHSS during COH and found that the improved function conferred to LHR by this polymorphism in vitro possibly is not reflective of the situation in the ovaries.
3.4 Oocyte Development and Quality
Human cumulus cells express long pentraxin 3 (PTX3), which is a member of complex superfamily of multifunctional proteins characterized by a cyclic multimeric structure [6]. PTX3 is highly conserved in evolution, and its protein is present in human cumulus matrix, suggesting that this molecule is essential in female fertility by acting as a nodal point for the assembly of the cumulus oophorushyaluronan-rich extracellular matrix. Moreover, a higher relative abundance of PTX3 mRNA in cumulus cells from fertilized oocytes has been detected compared with cumulus cells from unfertilized oocytes, indicating that PTX3 is a possible marker for oocyte quality [6].
Granulosa cells (GC) are the most important somatic cells for determining the final size of preovulatory follicles [7]. Luteinized GC can proliferate, and the telomerase activity (TA) of luteinized GC may predict the clinical outcome of IVF treatment. However, telomerase activity seems to be more significant for predicting the outcome of IVF treatment than telomere length (TL) in granulosa cells. Telomeres are the physical ends of eukaryotic chromosomes. They consist of a 5- to 15-kb-long tandem repeat hexanucleotide sequence (TTAGGG)n that protects the ends of the double-stranded DNA. Hence, telomeres play an essential role in the maintenance of chromosomal stability and cell viability. There is evidence that TL is longer in the oocytes of women who become pregnant than in those of women who fail to become pregnant after IVF treatment [7]. In addition, TL can predict oocyte development. Telomeric DNA deficiency is associated with genomic instability in somatic cells and plays a role in the development of aneuploidies commonly found in female germ cells and human embryos.
In GC of preantral follicles, NFIA, a transcription factor involved in the control of cell growth in humans and model systems, and HIF1A, an interacting protein that activates the transcription of target genes involved in energy metabolism, angiogenesis, and apoptosis, are over-expressed [8].
Luteinization of granulosa cells is initiated by the LH surge or the addition of LH or human chorionic gonadotropin (hCG). Nevertheless, it has been shown that the removal of granulosa cells from the follicle causes spontaneous luteinization in the absence of LH [9]. Therefore, luteinization is a differentiation pathway programmed before antral formation, and the only way follicles can escape this procedure is by inhibitory factors. Such inhibitors may be present in follicular fluid or may come directly from the oocyte itself [9]. The LH surge is able to remove such inhibitory factors to disrupt connections between granulosa cells and the oocyte and to induce genes that facilitate luteinization.
One molecule that may have a role in the prevention of luteinization is activin A [9], a dimeric glycoprotein and member of the transforming growth factor (TGF)-β superfamily. Human chorionic gonadotropin (hCG) and activin A have opposite effects on the luteinized granulosa cells involved in luteal formation, with hCG increasing luteinization to a more luteal phenotype [9]. Activins are necessary for follicular granulosa cell proliferation, FSHR regulation, FSH-induced aromatase expression, decreased theca cell androgen production and increased oocyte maturation. As activin A appears to have a positive role in the follicle and a negative role in the corpus luteum, its activity appears to be suppressed at the follicular–luteal transition [9].
Other luteinization inhibitors are the bone morphogenetic proteins (BMPs) [2]. The BMP cytokine system plays a crucial role in folliculogenesis and angiogenesis. The BMP cytokines are growth factors belonging to the transforming growth factor β superfamily. In the ovary, BMP cytokines act as luteinization inhibitors by suppressing LHR gene expression in GC. Of the BMP cytokines, BMP-7 is most highly expressed in the theca cell layer in the ovarian follicles. According to Akiyama et al. [2], BMP-7 can induce vascular endothelial growth factor (VEGF)-A mRNA and protein expression in human GC. In many species, VEGF, which is detected in the granulosa and theca layer of secondary follicles, is recognized as an important factor in the recruitment of a vascular network to the theca layer [2]. During the process of follicular development, the vasculature of the follicle is limited to the theca layer outside the basement membrane. Therefore, it is likely that follicles create a gradient of angiogenic factors to stimulate vascularization toward the basement membrane, maximizing the supply of oxygen, nutrients, and hormones to GC. Furthermore, in endothelial cells, BMP-7 can increase the number of cells, accelerate tube formation, and increase the VEGF receptor mRNA. Thus, endothelial cells could be stimulated to form vasculature by BMP-7 via two distinct mechanisms: induction of VEGF expression in GC and increased sensitivity of endothelial cells to VEGF [2].
3.5 Follicular Development and Signaling Pathways
VEGF promotes early folliculogenesis. Bonnet et al. [8] identified the overexpression of members of VEGF pathway, including VEGF-A and NRP1 (a VEGF receptor), in GC and overexpression of FLT1 (another VEGF receptor) in oocytes. These gene expressions suggest a role for the VEGF pathway in GC-oocyte and GC-GC crosstalks [8]. The VEGF pathway may protect GC against atresia. Atresia is an important process in folliculogenesis and concerns the majority of the follicles. Apoptosis is found in the oocytes of primordial follicles and progressively extends to GC of growing follicles. Bonnet et al. characterized the expression of different genes of the BCL2 family either in oocytes (BCL2L1, BCL2L10, BCL2L11 [BIM], BCL2L14 [BCLG]), or in GC (BCL2 BCL2L2, BOK). For example, the BCL2L1 gene plays a crucial role in the survival of germ cells. BCL2L10 may play other roles related to cell cycle control and oocyte maturation.
Oogenesis begins with the migration of primordial germ cells into the gonadal ridges and their proliferation within ovarian nests or cysts [1]. Then primary oocytes are developed, meiotic prophase starts, and primordial follicles are formed, each one consisting of a primary oocyte arrested at the diplotene stage of prophase I of meiosis. The primordial follicles may begin to grow immediately or after a gap depending on the species, or they become quiescent.
The reserve of primordial follicles seems to determine the ovarian activity of the adult [1]. In fact, the regulation of germ and somatic cell survival, proliferation, and differentiation involves the same factors and molecular mechanisms from the formation of the primordial follicles up to the stage when the follicles become gonadotropin dependent and enter terminal development. Two main signaling pathways play major roles in all these processes. The first one is the PTEN/PI3K/PDPK1 (previously known as PDK1)/AKT1 (previously known as AKT or PKB) signaling pathway, which regulates germ cell survival, follicular growth activation, and follicle growth [1]. This pathway is activated by various hormones, growth factors, and cytokines. Among them, insulin, insulin-like growth factors (IGF), and KIT ligand are crucial for the survival and differentiation of germ and somatic ovarian cells. The second important signaling pathway involves SMAD transcription factors, which are activated by factors of the transforming growth factor-B (TGFB) super family (i.e., BMP and AMH for the SMAD1/5/8 pathway) and the TGFB and activins for the SMAD2/3 pathway [1]. It orchestrates the formation and development of follicles under the control of oocyte (bone morphogenetic protein 15 [BMP15], growth differentiation factor-9 [GDF9])- and somatic cell (BMP2, BMP4, AMH, activins)-derived factors. BMP15, like other genes, is not expressed in the oocyte until the primary follicle stage and is involved in the transition from primary to secondary follicles [8]. However, βFGF, GDF 9, and BMP 4 are involved in the transition from primordial to primary follicles [8].
Various mutations in genes encoding the ligands, receptors, or signaling effectors of the PTEN/PI3K/PDPK1/AKT1 or the SMAD signaling pathways can accelerate the exhaustion rate of the ovarian reserves and cause premature ovarian insufficiency (POI) [1]. Mutations in some factors of the TGFβ super family affect the transition of growing follicles between the two follicular reserves. In humans, various mutations in BMP15 and, to a lesser extent, in GDF9 and INHA have been found to be associated with POI, while genetic variants of AMH and its receptor AMHRII are associated with different ages at menopause, confirming the importance of these factors for the lifespan of the ovarian reserves [1]. Antimullerian hormone (AMH) gene is the best endocrine marker of the population of small antral follicles in humans because AMH expression in female mammals is strictly restricted to granulosa cells of growing follicles, while the granulosa cells of the largest preantral and the small antral healthy growing follicles express the highest amounts of AMH in the ovaries [1].
The Notch signaling pathway contributes to cell communication by influencing cell proliferation, differentiation, and apoptosis, as mentioned by Bonnet et al. [8]. Notch is involved in GC proliferation through NOTCH1/ CNTN1 binding. According to Tanriverdi et al. [10], the Notch genes encode transmembrane receptors that are highly conserved evolutionarily and modulate cell proliferation, differentiation, and survival. The Notch signaling pathway is initiated by a receptor-ligand interaction between two neighboring cells [10]. Cleavage of the receptors occurs after Notch receptors bind to their ligands. The intracellular domain of the receptor releases and translocates to the nucleus, where Notch forms transcriptional complexes with transcription factors of the CSL family (C promoter binding factor 1/suppressor of hairless/Lag-1). Notch genes are actively expressed by cumulus cells during folliculogenesis [10]. However, Notch is not released by oocytes and atretic follicles. Tanriverdi et al. [10] showed that Notch signaling proteins (Notch1, Notch2, Notch3, Notch4, Jagged 1, and Jagged 2) can be an indicator for understanding the ovarian response in ovulation induction.
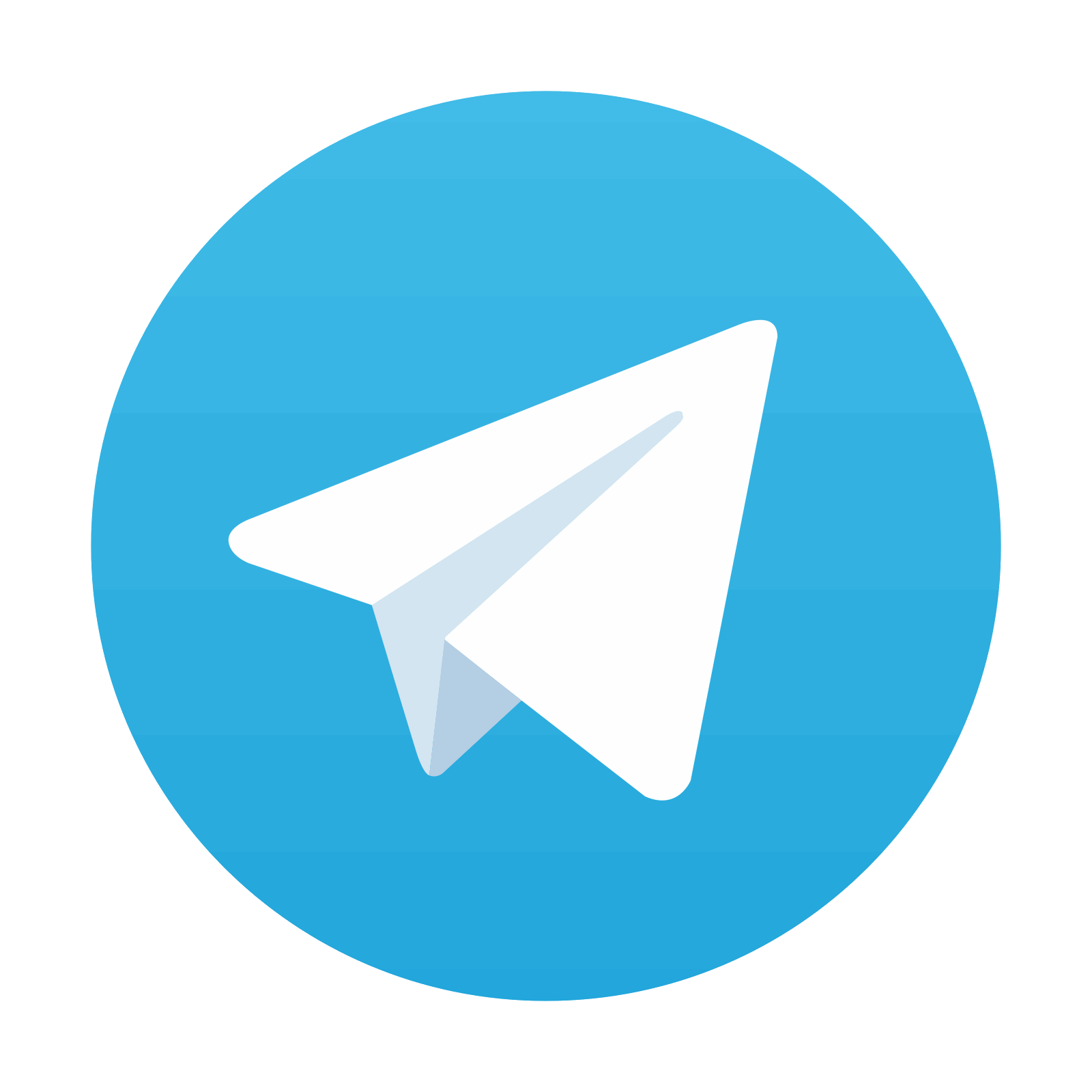
Stay updated, free articles. Join our Telegram channel

Full access? Get Clinical Tree
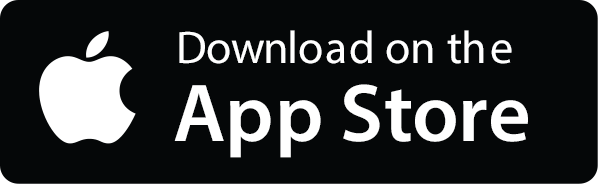
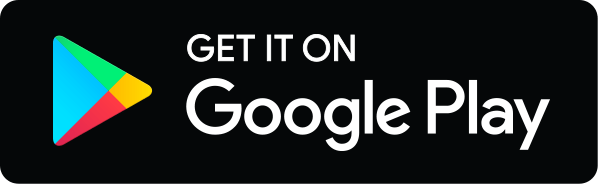