This article discusses recent advances in genomic approaches used to understand chronic lymphocytic leukemia. Tools for analyzing DNA-level lesions are described, data obtained from these various platforms summarized, and the clinical relevance of these findings discussed.
Key points
- •
Genomic approaches have led to a recent explosion in knowledge of the spectrum of genetic aberrations in chronic lymphocytic leukemia (CLL).
- •
Comparative genomic hybridization and high-resolution single-nucleotide polymorphism arrays have been used to detect alterations in copy number, some of which have been associated with prognosis and/or overall survival in CLL.
- •
Efforts to identify the genetic basis of familial CLL have included genome-wide linkage studies to identify CLL susceptibility genes as well as genome-wide association studies to determine CLL risk loci.
- •
Within the last couple of years, next-generation sequencing techniques have identified common recurrent somatic mutations in SF3B1 and NOTCH1 in CLL. Ongoing work involves the investigation of lower-frequency somatic mutations and clonal evolution over time as well as the identification of germline variants.
Genomics refers to the systematic study of an organism’s entire DNA sequence (genome). Molecular information derived from genomic techniques has increased our understanding of many complex diseases, including chronic lymphocytic leukemia (CLL). CLL is the most common form of adult leukemia and is characterized by a highly variable clinical course. Little is known about the molecular correlates underlying the different CLL disease patterns that are clinically evident, and despite epidemiologic evidence showing familial aggregation of CLL, no major predisposing genes have been identified. This article discusses the historical application of genomic techniques to these various problems in CLL: first, the use of comparative genomic hybridization (CGH) and single-nucleotide polymorphism (SNP) arrays to identify somatically acquired genetic alterations in CLL, then the use of genome-wide linkage analysis and genome-wide association studies (GWAS) in attempts to determine the cause of heritable predisposition to CLL. Finally, next-generation sequencing technology, which allows detection of both somatic and germline lesions in CLL at unprecedented speeds, is discussed.
Tumor analysis by comparative genomic hybridization
The first genome-wide copy-number analyses of CLL were made possible by the development of CGH in the 1990s. CGH allows the detection of chromosomal imbalances using differentially labeled tumor and normal DNA that are cohybridized to normal metaphase chromosomes. Intensities of the fluorescent labels are then used to determine overrepresentation (gain) or underrepresentation (loss) of genomic content in specific chromosomal regions.
In 1995, Bentz and colleagues first applied the CGH method to 28 CLL patients. Copy-number alterations (CNAs) were observed in 68% of the patients, with the most frequent gains found in chromosome 8q and 12 and the most frequent losses in chromosomes 6q, 11q, 13q, and 17p ( Table 1 ). A similar study, conducted by Karhu and colleagues using 25 CLL patients, observed chromosomal imbalances in 48% of the cases, with frequent gains in chromosome 12 and frequent losses in chromosome 11q and 13q (see Table 1 ).
Chromosome | CGH | SNP Arrays | ||
---|---|---|---|---|
Gains | Losses | Gains | Losses | |
1 | 1 (33%) | — | — | 1p31 (10%) |
2 | 2p (4%) 2p24 (6%) | 2 (25%) | 2p14 (28%) 2p16 (6%) 2p22 (28%) 2p (7%) | — |
3 | 3 (19%) 3q (7%) | 3p (4%) | 3q24 (17%) 3q26 (6%) | — |
4 | — | 4 (25%) 4q (4%) | — | — |
5 | — | 5 (17%) | — | — |
6 | Trisomy 6 (4%) | 6q (11%), (4%) 6q15 (4%) | — | 6q (7%) |
7 | — | 7q (4%) 7q31 (4%) | Trisomy 7 (10%) | 7q34 (4%) |
8 | 8q (11%) | 8p (4%) | 8q13 (10%) 8q21 (20%) 8q23 (28%) 8q24 (4%) 8q24.3 (28%) 8q24.13 (28%) 8q24.21 (5%) | 8p12 (28%) 8p21 (28%) 8p23 (10%), (28%), (5%) 8q12 (10%) |
9 | — | — | — | 9p21 (10%) 9q21 (22%) |
10 | — | 10q (4%) | — | — |
11 | 11 (38%) | 11q (14%), (24%), (17%), (13%) | — | 11q22 (13%), (10%), (6%), (27%) |
12 | Trisomy 12 (16%), (7%) 12p (4%) | 12p12 (4%) | Trisomy 12 (13%), (20%), (12%), (11%) | — |
13 | — | 13q (11%), (13%), (53%) 13q13-qter (4%) 13q14-q31 (4%) 13c-q31 (4%) | — | 13q14 (51%), (40%), (50%), (51.7%), (57%), (61%) |
14 | 14q (15%) | — | 14q32 (28%) | 14q31 (3%) 14q32 (33%) |
15 | Trisomy 15 (4%) 15q (4%) | 15q (4%) 15q11 (4%) | 15q26 (10%) | 15q13 (10%) 15q15.1 (4%) |
16 | — | 16p (4%) | — | — |
17 | 17q (7%) | 17p (29%), (4%), (17%) | 17q 21.1 (28%) 17q21.31 (33%) 17q21.32 (28%) | 17p11 (10%) 17p12 (6%) 17p13 (6%), (20%) 17p (8%) |
18 | 18q (4%) | 18p (7%) | 18q21 (10%) | 18q11 (10%) 18q22 (10%) |
19 | Trisomy 19 (4%), (5%) | — | 19p13 (20%) | 19p13 (20%) 19q13 (10%) |
20 | — | — | — | — |
21 | — | — | — | — |
22 | 22q (4%) | 22q11 (15%) | — | 22q11 (10%), (33%) |
X | X (42%) Xq22 (4%) | X (33%) | — | — |
To identify chromosomal abnormalities specifically in familial CLL, Summersgill and colleagues used CGH to analyze 24 pedigrees. The investigators detected at least 1 chromosomal imbalance in each patient, with an average of 7 abnormalities per case. The most common gain and loss were observed in the X chromosome (see Table 1 ). The chromosomal imbalances observed in the X chromosome appeared to be more common in familial CLL and were hypothesized to contribute to the differential survival of male and female CLL patients.
Classic CGH used metaphase chromosomes, which have limited resolution. Substitution of the chromosome targets with a matrix or array containing nucleic acids with defined sequences allowed the detection of much smaller gains and losses. This technique, known as matrix-CGH or array-CGH, makes use of sequence pools representative of whole chromosomes or chromosome arms, cloned in bacterial artificial chromosomes (BAC), P1-derived artificial chromosomes (PAC), or other vectors.
An automated matrix-CGH array specific for CLL was developed in 2004 and was validated against 106 CLL cases. Array profiles were compared with cytogenetic data, and showed high specificity and sensitivity. A total of 27 gains and 95 losses were detected using this approach. Novel recurrent genomic imbalances were identified, namely trisomy 19 and a small copy-number gain in the MYCN gene on chromosome 2p24 (see Table 1 ). Similarly, Gunn and colleagues analyzed 187 CLL cases using BAC array–based CGH. Copy-number changes were identified in 90% of these cases, with expected frequencies for the common genomic alterations, deletions of 13q, 11q, and 17p with 12 gain. In addition, submicroscopic deletions of chromosome 22q11 were observed in 28 cases (15%).
More recently, the authors’ group used high-resolution array-CGH to investigate differences between 37 sporadic and 38 familial cases. Sporadic cases showed significant association with 11q loss, whereas familial cases showed significant association with 14q11 gain. Alterations in 14q11 were also associated with mutated IGHV status and homozygous deletions in 13q. Homozygous deletion in 13q was associated with mutated IGHV , low expression of ZAP-70, and a significantly longer time to first treatment (TTFT).
Tumor analysis by comparative genomic hybridization
The first genome-wide copy-number analyses of CLL were made possible by the development of CGH in the 1990s. CGH allows the detection of chromosomal imbalances using differentially labeled tumor and normal DNA that are cohybridized to normal metaphase chromosomes. Intensities of the fluorescent labels are then used to determine overrepresentation (gain) or underrepresentation (loss) of genomic content in specific chromosomal regions.
In 1995, Bentz and colleagues first applied the CGH method to 28 CLL patients. Copy-number alterations (CNAs) were observed in 68% of the patients, with the most frequent gains found in chromosome 8q and 12 and the most frequent losses in chromosomes 6q, 11q, 13q, and 17p ( Table 1 ). A similar study, conducted by Karhu and colleagues using 25 CLL patients, observed chromosomal imbalances in 48% of the cases, with frequent gains in chromosome 12 and frequent losses in chromosome 11q and 13q (see Table 1 ).
Chromosome | CGH | SNP Arrays | ||
---|---|---|---|---|
Gains | Losses | Gains | Losses | |
1 | 1 (33%) | — | — | 1p31 (10%) |
2 | 2p (4%) 2p24 (6%) | 2 (25%) | 2p14 (28%) 2p16 (6%) 2p22 (28%) 2p (7%) | — |
3 | 3 (19%) 3q (7%) | 3p (4%) | 3q24 (17%) 3q26 (6%) | — |
4 | — | 4 (25%) 4q (4%) | — | — |
5 | — | 5 (17%) | — | — |
6 | Trisomy 6 (4%) | 6q (11%), (4%) 6q15 (4%) | — | 6q (7%) |
7 | — | 7q (4%) 7q31 (4%) | Trisomy 7 (10%) | 7q34 (4%) |
8 | 8q (11%) | 8p (4%) | 8q13 (10%) 8q21 (20%) 8q23 (28%) 8q24 (4%) 8q24.3 (28%) 8q24.13 (28%) 8q24.21 (5%) | 8p12 (28%) 8p21 (28%) 8p23 (10%), (28%), (5%) 8q12 (10%) |
9 | — | — | — | 9p21 (10%) 9q21 (22%) |
10 | — | 10q (4%) | — | — |
11 | 11 (38%) | 11q (14%), (24%), (17%), (13%) | — | 11q22 (13%), (10%), (6%), (27%) |
12 | Trisomy 12 (16%), (7%) 12p (4%) | 12p12 (4%) | Trisomy 12 (13%), (20%), (12%), (11%) | — |
13 | — | 13q (11%), (13%), (53%) 13q13-qter (4%) 13q14-q31 (4%) 13c-q31 (4%) | — | 13q14 (51%), (40%), (50%), (51.7%), (57%), (61%) |
14 | 14q (15%) | — | 14q32 (28%) | 14q31 (3%) 14q32 (33%) |
15 | Trisomy 15 (4%) 15q (4%) | 15q (4%) 15q11 (4%) | 15q26 (10%) | 15q13 (10%) 15q15.1 (4%) |
16 | — | 16p (4%) | — | — |
17 | 17q (7%) | 17p (29%), (4%), (17%) | 17q 21.1 (28%) 17q21.31 (33%) 17q21.32 (28%) | 17p11 (10%) 17p12 (6%) 17p13 (6%), (20%) 17p (8%) |
18 | 18q (4%) | 18p (7%) | 18q21 (10%) | 18q11 (10%) 18q22 (10%) |
19 | Trisomy 19 (4%), (5%) | — | 19p13 (20%) | 19p13 (20%) 19q13 (10%) |
20 | — | — | — | — |
21 | — | — | — | — |
22 | 22q (4%) | 22q11 (15%) | — | 22q11 (10%), (33%) |
X | X (42%) Xq22 (4%) | X (33%) | — | — |
To identify chromosomal abnormalities specifically in familial CLL, Summersgill and colleagues used CGH to analyze 24 pedigrees. The investigators detected at least 1 chromosomal imbalance in each patient, with an average of 7 abnormalities per case. The most common gain and loss were observed in the X chromosome (see Table 1 ). The chromosomal imbalances observed in the X chromosome appeared to be more common in familial CLL and were hypothesized to contribute to the differential survival of male and female CLL patients.
Classic CGH used metaphase chromosomes, which have limited resolution. Substitution of the chromosome targets with a matrix or array containing nucleic acids with defined sequences allowed the detection of much smaller gains and losses. This technique, known as matrix-CGH or array-CGH, makes use of sequence pools representative of whole chromosomes or chromosome arms, cloned in bacterial artificial chromosomes (BAC), P1-derived artificial chromosomes (PAC), or other vectors.
An automated matrix-CGH array specific for CLL was developed in 2004 and was validated against 106 CLL cases. Array profiles were compared with cytogenetic data, and showed high specificity and sensitivity. A total of 27 gains and 95 losses were detected using this approach. Novel recurrent genomic imbalances were identified, namely trisomy 19 and a small copy-number gain in the MYCN gene on chromosome 2p24 (see Table 1 ). Similarly, Gunn and colleagues analyzed 187 CLL cases using BAC array–based CGH. Copy-number changes were identified in 90% of these cases, with expected frequencies for the common genomic alterations, deletions of 13q, 11q, and 17p with 12 gain. In addition, submicroscopic deletions of chromosome 22q11 were observed in 28 cases (15%).
More recently, the authors’ group used high-resolution array-CGH to investigate differences between 37 sporadic and 38 familial cases. Sporadic cases showed significant association with 11q loss, whereas familial cases showed significant association with 14q11 gain. Alterations in 14q11 were also associated with mutated IGHV status and homozygous deletions in 13q. Homozygous deletion in 13q was associated with mutated IGHV , low expression of ZAP-70, and a significantly longer time to first treatment (TTFT).
Tumor analysis by high-resolution single-nucleotide polymorphism arrays
The necessity of overcoming the resolution limits of classic CGH (10–20 Mb) and of array-CGH (0.1 Mb) prompted the use of even higher-resolution platforms. In 2004, Bignell and colleagues demonstrated the utility of using SNP arrays, originally designed for genotyping, to detect CNAs at a genome-wide level. Using the Affymetrix p501 array as a prototype, they showed that simultaneous genotyping and copy-number analysis of cancer cell lines allowed the detection of genomic alterations that would have been missed by array-CGH or genotyping alone, including loss of heterozygosity (LOH) in copy neutral regions as in the case of uniparental disomy (UPD) or as acquired in cancer.
SNP arrays were first applied to CLL samples by Pfeifer and colleagues, using the Affymetrix 10K and 50K arrays. These investigators identified chromosomal imbalances in 65.6% and 81.5% of cases, respectively, indicating greater sensitivity of higher-density arrays. Deletion 13q14 was the most frequent aberration found, followed by trisomy 12, del11q22, and del17p13 (see Table 1 ). In addition, they identified 24 regions with LOH without altered gene dosage.
Kujawski and colleagues subsequently used the 50K Affymetrix SNP array to quantify genome-wide allelic imbalances, including LOH, in 178 CLL patients, to derive a genomic complexity score. The complexity scores correlated well with their clinical end points, TTFT, and time to subsequent therapy (TTST). Specifically, high genomic complexity was found to be an independent risk factor for disease progression and treatment failure.
Forconi and colleagues used a higher-density array (250K Affymetrix) to specifically investigate patients with deletions in 17p, as these show aggressive disease. All cases displayed multiple copy-number changes, with frequent losses in chromosome 8p and frequent gains in chromosome 8q and 2p (see Table 1 ). 8p loss and 2p gain predicted shorter TTFT and poorer overall survival in these 17p patients. The authors subsequently reported a similar association between 8p loss and 17p deletion using the SNP6.0 array.
To determine which platforms allowed reliable detection of CNAs, Gunnarsson and colleagues carried out a comparative study of 4 high-resolution platforms: BAC arrays (32K), oligonucleotide arrays (185K), and 2 SNP arrays (Affymetrix 250K and Illumina 317K). All platforms were able to robustly detect large aberrations, with 29 CNAs concordantly detected, including common alterations. However, small CNAs were detected only by the high-density oligonucleotide and SNP arrays. The oligonucleotide array had lower baseline variation in comparison with the other platforms. The 250K Affymetrix array detected more CNAs than the 317K Illumina array, but the latter detected more LOH events.
To assess the performance of SNP arrays for routine clinical use, Hagenkord and colleagues compared low-density (Affymetrix 10K2.0), medium-density (Affymetrix 250K Nsp ), and high-density (Affymetrix SNP6.0) SNP arrays. The 10K2.0 array was found to be unsuitable for use in the clinic because of its relatively poor resolution. The SNP6.0 array was superior in detecting small aberrations but was equivalent to the 250K array for detecting lesions known to be clinically relevant. Furthermore, the 250K array was less costly and easier to manage. The 250K array demonstrated 98.5% concordance with the standard CLL fluorescence in situ hybridization (FISH) panel, but also detected acquired UPD and additional regions of genomic complexity.
Ouillette and colleagues also performed copy-number analysis of 255 CLL patients using Affymetrix 6.0 arrays, and observed at least 2 CNAs in 39% of all cases and 3 or more CNAs in 20% of patients. In addition, they correlated genomic complexity (defined as the total number of CNAs) with clinical outcomes, and found elevated genomic complexity to be an independent marker for aggressive CLL and short overall survival. The same group also analyzed SNP 6.0 arrays to specifically define 13q14 deletions in 255 CLL patients. Large 13q14 deletions encompassing the RB1 gene were detected in 20% of these patients and were associated with decreased survival.
Recently, the authors’ group integrated copy-number analysis using Affymetrix 6.0 SNP arrays together with gene-expression profiling in 161 CLL patients. With matched germline controls a median of only 1 somatic CNA per sample was found, suggesting that the CLL genome is relatively stable. The authors identified recurrent CNAs associated with short TTFT: 8q24 amplification, 3q26 amplification, and 8p deletions (see Table 1 ). Amplifications of 3q26 were focused on the PIK3CA gene and amplifications of 8q were focused on MYC and on the regulatory region near MYC , which has been implicated by GWAS in disease risk in CLL and many other cancers.
Similarly, Edelmann and colleagues used the Affymetrix 6.0 array to analyze 353 untreated CLL samples, and identified an average of 1.8 CNAs per case with copy-neutral LOH being found in 6% of cases, most frequently in 13q, 17p, and 11q. Chromosome 13q14 was deleted in 61% of cases, with minimally deleted regions refined to the DLEU1 and DLEU2 genes (see Table 1 ). These investigators also found novel lesions including a frequent deletion at 15q15.1 (4%), with the smallest deletion found in the MAX gene–associated ( MGA ) gene locus.
Taken together, CGH and SNP array studies of CLL tumors have identified recurrently altered loci that are likely involved in the pathogenesis of CLL and could even potentially be involved in CLL susceptibility. The extent of genomic aberrations, as well as the presence of specific CNAs in addition to those classically identified by FISH, such as 2p gain, 3q gain, 8p deletion, and 8q gain, have been suggested to have clinical relevance and should be tested in prospective clinical trials to evaluate their true usefulness as predictors for clinical outcomes.
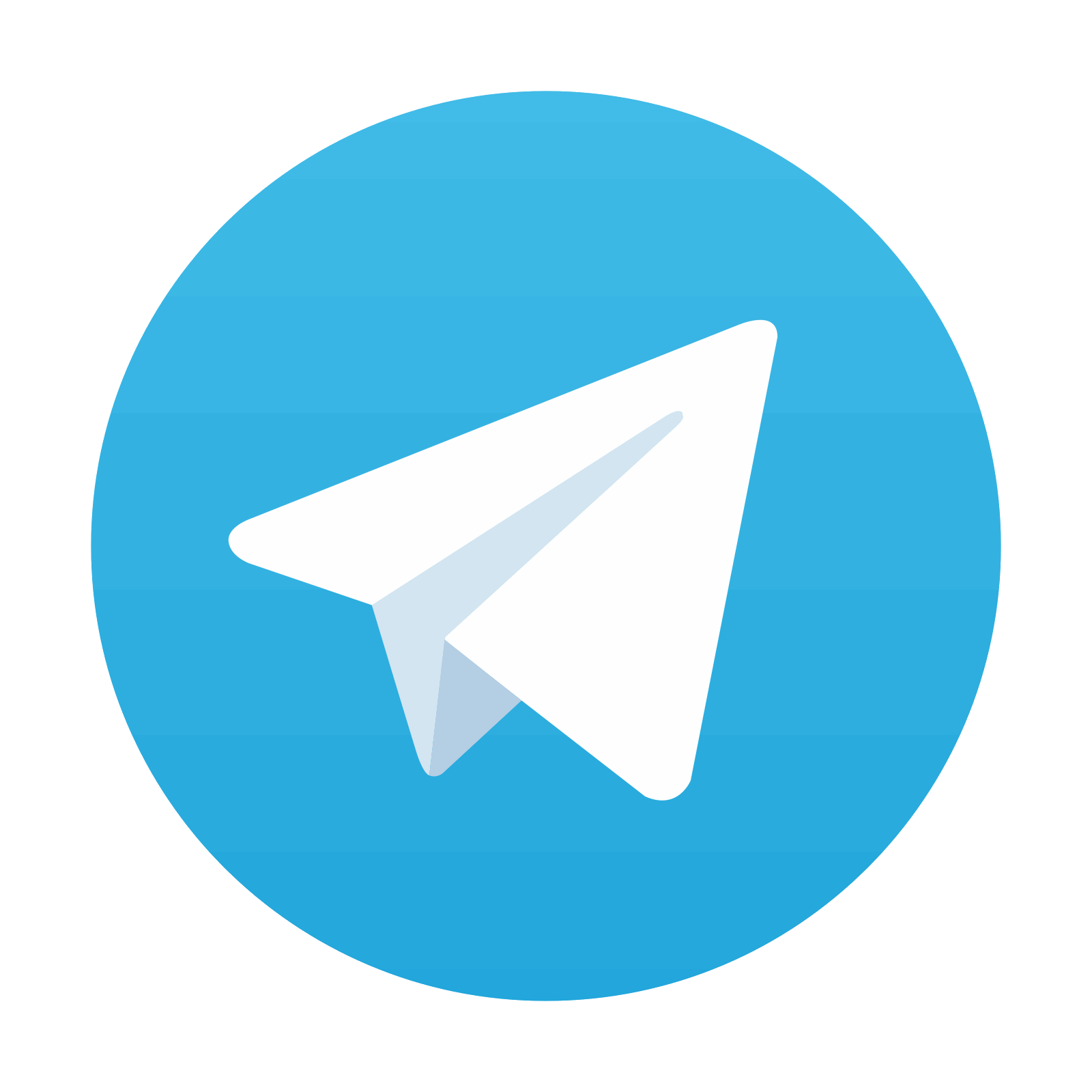
Stay updated, free articles. Join our Telegram channel

Full access? Get Clinical Tree
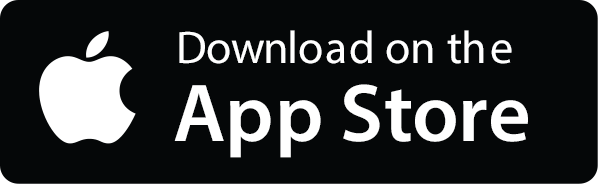
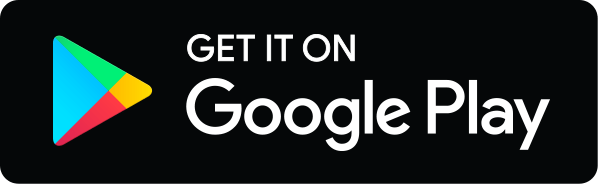