Edwin Smith Papyrus (3000 BC)
Described a breast cancer-like disease
Eberus Papyrus (1500 BC)
Described soft tissue tumors and used cautery, knives, salts, and arsenic paste to treat this
Hippocrates (Greek physician, 460–375 BC)
Used the terms carcinos and carcinoma to describe non-ulcer-forming and ulcer-forming tumors
Aulus Celsus (Roman physician, 28 BC–50 AD)
Translated the Greek term into cancer, the Latin word for crab
Gaius Plinius Secundus (Roman, 23–79 AD)
Recommended herbal remedies for advanced cancer
Aretaeus of Cappadocia (Egyptian physician, 81–138 AD)
Described symptoms, signs, and treatment of uterine cancer; classified cancer into ulcerated and non-ulcerated
Claudius Galen (Greek physician, 130–200 AD)
Used the word oncos (Greek for swelling) to describe tumors
Oribasius (Greek physician, 325–403 AD)
Identified necrosis in cancer
Lanfranc of Milan (1252–1315 AD)
Differentiated benign tumors of the breast from cancer
Guy de Chauliac (French surgeon, 1300–1368 AD)
Distinguished hot and cold (cancer) lesions
Antonio Benivieni (Florentine physician, 1507 AD)
Published first case report of gastric cancer
Paracelsus (Swiss-German physician, 1493–1541)
Therapeutic use of chemicals, described industrial lung cancer
Ambroise Pare (French Army Surgeon, 1575)
Treated breast cancer with mercury-covered sheet of lead
Felix Platter (Basel physician, 1526–1614)
First described brain tumor
Thomas Venner (London, 1620)
Warned about tobacco dangers in his Via Recta
William Harvey (1628)
Described the systemic blood circulation through the heart and body
Zacutus Lusitani and Nicolaes Tulp (1649)
Created the “Contagion Theory”
George Stahl and Friedrich Hoffmann (1660–1742)
Tumors grow from lymph constantly thrown out by the blood
Bernardino Ramazzini (Italian doctor, 1713)
Absence of cervical cancer in nuns linked to high breast cancer
Giovanni Battista Morgagni (Padua, 1761)
Did autopsies to connect pathologic findings with cancer
John Hill (London surgeon, 1761)
Recognized tobacco as a carcinogen
Percival Pott (London, 1775)
Linked soot in the skin folds of the scrotum to cancer
John Hunter (Scottish surgeon, 1728–1793)
Suggested that some cancers might be cured by surgery
Jean Louis Petit (French surgeon, eighteenth century)
Linked lymphatic glands with breast tumor
Joseph Lister (British surgeon, 1827–1912)
Was the first to perform an adequate axillary dissection
Julius Vogel (Germany, 1845)
Introduced an Atlas of cancer pathology
Henry Bence Jones (English physician, 1848)
Discovered Bence Jones protein
John Birkett (English surgeon, 1850)
Introduced the term lobular carcinoma
James Paget (English surgeon, 1853)
Described the Paget disease of the breast
Alfred Velpeau (French surgeon, 1854)
Described and illustrated bilateral breast cancer
Karl Thiersch (German surgeon, 1860)
Cancers metastasize through the spread of malignant cells
Moritz Kaposi (Hungarian dermatologist, 1872)
Reported Kaposi sarcoma
Richard Von Volkmann (German surgeon, 1873)
Advocated the routine complete removal of the breast
Rudolph Virchow (1821–1902)
All cells, including cancer cells, are derived from other cells
Gaillard Thomas (New York, 1876)
Recommended the use of intraoperative frozen section
Thomas Beatson (Edinburgh, 1878)
Hormonal communication between breasts and ovary in rabbits
William Stewart Halsted (American surgeon, 1889)
Developed radical surgery of breast cancer
Charles Huggins (University of Chicago, 1901–1997)
Regression of metastatic prostate cancer
William Conrad Roentgen (German, 1896)
Discovered “X-Ray,” used to treat cancer
George T. Beatson (Glasgow surgeon, 1896)
Introduced oophorectomy for inoperable breast carcinomas
Pierre Curie and Marie Curie (1898)
Discovered radium which was later used for cancer treatment
David P. von Hansemann (German pathologist, 1902)
Introduced histologic grading of carcinoma
Hugh H. Young (American surgeon, 1904)
Carried out a radical operation for prostate cancer
Paul Ehrlich (German biochemist, 1909)
Introduced the word “chemotherapy”
Peyton Rous (Pathologist, 1910)
Transmitted sarcoma in hens by cell-free filtrate
Peyton Rous (New York, 1911)
Induced cancer in chickens by Rous sarcoma virus
Alexis Carrel and Montrose T. Burrows (1914)
First to grow human cancer tissue in culture
Katsusaburo Yamagiwa and Koichi Ichikawa (1915)
Induced cancer by tar in animals
Edward B. Krumbhaar (US Army pathologist, 1919)
Noticed that mustard gas causes leukopenia
James Ewing (Hematopathologist, 1921)
Reported a deadly bone cancer known as Ewing’s sarcoma
George Papanicolaou (1923)
Developed Pap smear test for cervical cancer diagnosis
Antoine M. Lacassagne (French physician, 1932)
Breast cancer can be induced by estrogen injections in mice
Harvey W. Cushing (American neurosurgeon, 1932)
Reported Cushing’s disease
Yellapragada Subbarao (1947)
Discovered methotrexate as anticancer agent
Sidney Farber (1948)
Showed remission of childhood leukemia with aminopterin
Sauberlich and Baumann (1948)
Discovered leucovorin from Leuconostoc citrovorum
James Watson and Francis Crick (1953)
Discovered the chemical structure of DNA
Charles Heidelberger (1957)
Discovered 5-fluorouracil as an anticancer drug
Renato Dulbecco (1975)
Discovered viruses that can cause cancer
Howard Temin and David Baltimore (1975)
Discovered the enzyme reverse transcriptase
Robert L. Egan (1956)
Used low-energy X-rays to diagnose human breast cancer
Denis P. Burkitt (1958)
Discovered childhood cancer; named it “Burkitt’s lymphoma”
Stanley Cohen (1962)
Isolated first growth factor, epithelial growth factor
Ozegowski and Krebs (1963)
Discovered bendamustine, an alkylating agent,
Di Marco A (1965)
Discovered daunorubicin from a bacterium
Hamao Umezawa (1966)
Discovered bleomycin
Monroe Wall and Mansukh Wani (1967)
Discovered paclitaxol as an anticancer drug
von Wartburg and Hartmann F. Stähelin (1967)
Discovered podophyllotoxin that lead to etoposide
John Montgomery and Kathleen Hewson (1968)
Discovered fludarabine as an anticancer drug
G. Steve Martin (1970)
Discovered first oncogene that can cause cancer
Yoshinori Kidan (1976)
Discovered anticancer drug oxaliplatin
Larry Hertel (1986)
Discovered gemcitabine, an antiviral drug to kill leukemia cells
Sun HD (1992)
Discovered arsenic trioxide, effective in acute promyelocytic leukemia
Brian Druker and Nick Lydon (1996)
Discovered imatinib, a BCR-ABL inhibitor for chronic myeloid leukemia
2.3 Cancer Is a Disease of Old Age
The incidence of cancer increases as we get older; only 2 % of all cancers are diagnosed in children, the remaining 98 % are diagnosed in adults. In the United States, the average age at diagnosis of cancer in both males and females is 45–50 years (http://www.cancer.org/index). Interestingly, however, after age 70 years, the incidence of most cancers declines (Anand et al. 2008a), perhaps in part due to the aging of the cancer cells. Naked mole rats, however, challenge the theories that link cancer with aging (Kim et al. 2011). This animal shows negligible senescence, no age-related increase in mortality, and high fecundity until death. In addition to experiencing delayed aging, it is resistant to both spontaneous cancer and experimentally induced tumorigenesis.
2.4 Cancer Is More Common in Western/Developed Countries
According to the WHO, the global cancer burden jumped to 14.1 million new cases and accounted for 8.2 million deaths in 2012 (http://globocan.iarc.fr/Pages/fact_sheets_cancer.aspx). Epidemiological data suggest that both cancer incidence and cancer mortality are much more prevalent in developed countries than in developing countries (Table 2.2). In the United States, for instance, with a population of 320 million, two in every three people are diagnosed with cancer today. In 2014, 1,665,540 new cases of cancer will be diagnosed and 585,720 patients will die of this disease in the United States (http://www.cancer.org/research/cancerfactsstatistics/cancerfactsfigures2014/). When adjusted for age, 459 of 100,000 people will be diagnosed as having cancer. In the United States today, there are 14.5 million cancer survivors; by 2024, the number of cancer survivors is estimated to increase to almost 19 million. In comparison, in India, a developing country with a population of 1.3 billion, cancer was diagnosed in 1,000,000 people in 2013 and 700,000 people died of this disease (http://timesofindia.indiatimes.com/india/7-lakh-Indians-died-of-cancer-last-year-WHO/articleshow/27317742.cms). One in 10 Indians is at risk of developing cancer before 75 years of age, whereas 7 in 100 are at risk of dying from cancer before age 75 (Goss et al. 2014).
Table 2.2
Cancer incidence and mortality in the top 50 most populated countries
Country | Population | New cases | Deaths | Incidence/100,000 | Deaths/100,000 |
---|---|---|---|---|---|
China | 1,343,239,923 | 3,065,400 | 2,205,900 | 174 | 122 |
India | 1,205,073,612 | 1,014,900 | 682,000 | 94 | 65 |
United States | 313,847,465 | 1,603,600 | 617,200 | 318 | 106 |
Indonesia | 248,645,008 | 299,700 | 194,500 | 134 | 89 |
Brazil | 193,946,886 | 437,600 | 224,700 | 206 | 104 |
Pakistan | 190,291,129 | 148,000 | 101,000 | 112 | 80 |
Nigeria | 170,123,740 | 102,100 | 71,600 | 100 | 72 |
Bangladesh | 161,083,804 | 122,700 | 91,300 | 104 | 81 |
Russia | 142,517,670 | 458,400 | 295,400 | 204 | 123 |
Japan | 127,368,088 | 703,900 | 378,000 | 217 | 94 |
Mexico | 114,975,406 | 148,000 | 78,000 | 132 | 67 |
Philippines | 103,775,002 | 98,200 | 59,000 | 140 | 91 |
Vietnam | 91,519,289 | 125,000 | 94,700 | 140 | 109 |
Ethiopia | 91,195,675 | 61,000 | 45,000 | 108 | 85 |
Egypt | 83,688,164 | 108,600 | 152,000 | 72 | 103 |
Germany | 81,305,856 | 493,800 | 217,600 | 284 | 101 |
Turkey | 79,749,461 | 148,000 | 91,800 | 205 | 129 |
Iran | 78,868,711 | 84,800 | 53,400 | 128 | 82 |
Congo | 73,599,190 | 37,400 | 30,800 | 108 | 93 |
Thailand | 67,091,089 | 108,600 | 152,000 | 72 | 103 |
France | 65,630,692 | 371,700 | 154,600 | 325 | 108 |
United Kingdom | 63,047,162 | 327,800 | 157,800 | 273 | 110 |
Italy | 61,261,254 | 354,500 | 170,000 | 279 | 102 |
Burma | 54,584,650 | 63,600 | 49,200 | 141 | 113 |
South Korea | 48,860,500 | 55,400 | 38,900 | 181 | 126 |
South Africa | 48,810,427 | 77,400 | 47,400 | 187 | 118 |
Spain | 47,042,984 | 215,500 | 102,800 | 249 | 98 |
Tanzania | 46,912,768 | 33,900 | 23,600 | 124 | 92 |
Colombia | 45,239,079 | 71,400 | 37,900 | 161 | 85 |
Ukraine | 44,854,065 | 141,000 | 87,300 | 193 | 114 |
Kenya | 43,013,341 | 41,000 | 28,500 | 182 | 135 |
Argentina | 42,192,494 | 115,200 | 66,400 | 217 | 115 |
Poland | 38,415,284 | 152,200 | 24,100 | 246 | 99 |
Algeria | 37,367,226 | 37,900 | 21,700 | 124 | 75 |
Canada | 34,300,083 | 182,200 | 74,100 | 296 | 103 |
Sudan | 34,206,710 | 8,700 | 6,600 | 133 | 106 |
Uganda | 33,640,833 | 29,400 | 21,500 | 170 | 134 |
Morocco | 32,309,239 | 35,000 | 22,800 | 118 | 78 |
Iraq | 31,129,225 30,419,928 | 25,700 | 17,500 | 135 | 97 |
Afghanistan | 20,000 | 15,400 | 115 | 98 | |
Nepal | 29,890,686 | 18,800 | 14,300 | 85 | 68 |
Peru | 29,549,517 | 42,800 | 26,200 | 155 | 92 |
Malaysia | 29,179,952 | 37,400 | 21,700 | 144 | 86 |
Uzbekistan | 28,394,180 | 22,600 | 14,900 | 100 | 70 |
Venezuela | 28,047,938 | 41,800 | 23,500 | 140 | 86 |
Saudi Arabia | 26,534,504 | 17,500 | 9,100 | 91 | 54 |
Yemen | 24,771,809 | 11,400 | 8,400 | 80 | 64 |
Ghana | 24,652,402 | 15,800 | 10,700 | 92 | 64 |
North Korea | 24,589,122 | 219,500 | 81,500 | 308 | 100 |
Mozambique | 23,515,934 | 22,000 | 17,000 | 137 | 115 |
Among the most populated countries in the world, China, with a population of 1.4 billion, had 2.6 million patients who were diagnosed as having cancer in 2010, and 1.8 million died of the disease (http://english.peopledaily.com.cn/90001/90776/90882/7122372.html). In comparison, in 2013, 3.5 million new cancer cases were identified and 2.5 million cancer deaths were reported (http://www.china.org.cn/2013-04/16/content_28556502.htm). These numbers are more comparable with those of the United States than with those of India.
The types of cancer that occur in various countries vary a great deal. In the United States, the top five cancers in men are lung, colon, bladder, melanoma, and prostate; in US women, the top cancers are breast, lung, colon, and melanoma. In India, however, the top five cancers in men are lip/oral cavity, lung, stomach, colorectum, and pharynx, whereas among women they are breast, cervix, colorectum, ovary, and lip/oral cavity.
In comparing the incidence of age-standardized prostate cancer in the United States and India, the US incidence was found to be 12.8 per 100,000 but was 4.4 per 100,000 in India, suggesting that cancer is a disease of lifestyle. As further evidence, one-third of all cancers in the United States have been linked to cigarette smoking, whereas half of all cancers in Indian men have been linked to smoking and chewing tobacco. Overall, another third of all cancers have been linked to diet, 14–20 % to obesity, 18 % to infections, and 7 % to environmental pollution and radiation (Anand et al. 2008a). Heredity plays a role in causing cancer, but in only 5 % of cases. Further evidence that cancer is a disease of lifestyle has been seen in immigrants such as women from Japan, who after immigrating to the United States had an increased incidence of breast cancer, from about 10 per 100,000 to more than 80 per 100,000 over the course of three generations (Kolonel et al. 2004).
Sadly, the incidence of global cancer appears to be increasing, with new cases expected to reach 16 million in 2020 and 20 million in 2030; the numbers of cancer deaths are expected to increase as well to 10 million and 14 million, respectively.
Although the incidence of cancer is increasing throughout the world, the incidence of infectious diseases is decreasing worldwide. Although certain cancers are caused by infections, the question has been raised about whether other cancers might be prevented by infections, perhaps through activation of the immune system. Investigation of the reciprocal relationship between infection and cancer goes back through the centuries (Aggarwal 2003) and led to the discovery of Coley’s toxin, used even today (McCarthy 2006). In fact, Bacillus Calmette-Guerin (BCG) treatment used for bladder cancer is based on these discoveries (Kamat et al. 2009). Intravesical instillation of BCG was first used for bladder cancer therapy in 1974 (Morales et al. 2002); to date, it is the most successful adjuvant agent for treating noninvasive bladder cancer (Herr et al. 2011).
Chronic infections, obesity, alcohol, tobacco, irradiation, environmental pollutants, and high-calorie diet have been recognized as major risk factors for the most common types of cancer (Ahn et al. 2007). All these risk factors are linked to cancer through inflammation. Linkage between cancer and inflammation is indicated by transcription factors, nuclear factor-kappa B (NF-κB), and signal transducers and activators of transcription 3 (STAT3), two major pathways for inflammation that are activated by most cancer risk factors (Aggarwal et al. 2009b; Aggarwal 2005). NF-κB itself and proteins regulated by it have been linked to cellular transformation, proliferation, apoptosis suppression, invasion, angiogenesis, and metastasis of cancer (Aggarwal 2004, 2009). However, controlled activation of NF-κB is needed for the function of the immune system, and its constitutive activation can cause inflammation and tumorigenesis (Aggarwal and Sung 2011). Thus, it exhibits a double-edged sword and behaves like friend or foe depending on the activation (Shishodia and Aggarwal 2004). Chemotherapeutic agents and gamma irradiation activate NF-κB and lead to chemoresistance and radioresistance (Ahn et al. 2008). Thus, suppression of these proinflammatory pathways may provide opportunities for both prevention and treatment of cancer (Aggarwal 2006, 2008a; Ralhan et al. 2009).
2.5 Cancer Is a Multigenic Disease
Extensive research in the last half of the twentieth century revealed that cancer is caused by dysregulation of not a single gene but multiple genes. Since cancer is a hyperproliferative disorder, most of these genes are linked to the proliferation of tumor cells. Investigation of the genomic landscape of the most common cancers has recently revealed that a limited number of genetic alterations are responsible for most cancer subtypes. Approximately 140 of the 23,000 human genes appear to drive almost all cancers (Vogelstein et al. 2013). How many genes have undergone mutations and what are those genes has also been identified for most cancers (Table 2.3). Interestingly, although 163 mutations were identified in small-cell lung cancer, as few as 8 mutations were linked with acute amyloid leukemia. Although some of these genes and their roles in cancer are well recognized, the functions of quite a few cancer-linked genes are not fully understood. For instance, NOTCH-1, p53, Wnt, EGFR, Ras, Raf, PI3K, HER2, BCR-ABL, ER, PR, PSA, and JAK2 genes have been studied extensively, and their roles in cancer are well recognized. In contrast, the roles of other genes in cancer, including ATRX, DAXX, CD20, and CD25, are still not fully understood.
Table 2.3
Identification of various somatic mutations and predictive tumor biomarkers in human cancers
Cancers | No. of mutations | Predictive tumor biomarkers |
---|---|---|
Pediatric cancers | ||
Glioblastoma | 14 | H3F3A/ATRX, DAXX, TP53 |
Neuroblastoma | 12 | |
Acute lymphocytic leukemia | 11 | NOTCH-1 |
Medulloblastoma | 8 | HH, Wnt, MLL2, MLL3 |
Rhabdoid cancer | 4 | |
Adult cancers | ||
Lung cancer (small cell) | 163 | EGFR, EML4-ALK, KRAS, MEK, SOX2 |
Lung cancer (non-small cell) | 147 | EGFR, SIK2 kinases, ARID1A, RBM10 |
Melanoma | 135 | BRAF |
Esophageal squamous cell cancer | 79 | |
Non-Hodgkin’s lymphoma | 74 | CD25 |
Colorectal cancer | 66 | BRAF, CEA, EGFR, KRAS, PI3CA, UGT1A1, MSI/MMR |
Head and neck cancer | 65 | TP53, CDKN2A, PI3K3CA, HRAS, FBXW7, NOTCH-1, TP63, IRF6, PTEN, HRAS |
Esophageal adenocarcinoma | 57 | |
Gastric cancer | 53 | CA19-9, HER2, ARID1A |
Endometrial cancer | 49 | |
Pancreatic cancer | 45 | CA 19–9, HER2 |
Ovarian cancer | 42 | CA-125, HE4, OVA1, PI3KCA, ARID1A, PPP2R1A, KRAS |
Prostate cancer | 41 | AR, PSA, ETS2, MLL2; FOXA1, MLL2, UTX, ASXL1 |
Hepatocellular cancer | 39 | ARID1A |
Glioblastoma | 35 | 1p/19q, IDH1, IDH2 |
Breast cancer | 33 | CYP2D6, ER, HER2, PI3KCA, PR |
Chronic lymphocytic leukemia | 12 | CD20 |
Chronic myeloid leukemia | BCR-ABL | |
Acute myeloid leukemia | 8 | CEBPA, FLT3, Kit, NPM1, IDH-1, IDH-2, JAK2, TET2, DNMT3A |
Diffuse large B-cell lymphoma | 30 | MLL2 |
Liver | AFP | |
Kidney | Non-LDT | |
Bladder | NMP22 |
A number of lessons have been learned from these genomic studies. For instance, it was calculated that in colorectal tumorigenesis, approximately 17 years are needed for a large benign tumor to evolve into advanced cancer, and less than 2 years are needed for cells within that cancer to acquire the ability to metastasize (Jones et al. 2008). Furthermore, it was found that few selective events are required to transform a highly invasive cancer cell into one with the capacity to metastasize. Another study showed that half or more of the somatic mutations in cancers of self-renewing tissues originate before tumor initiation (Tomasetti et al. 2013). Welch et al. found that most of the mutations in acute myeloid leukemia (AML) genomes are actually random events that occurred in hematopoietic stem/progenitor cells before they acquired the initiating mutation (Welch et al. 2012). In many cases, only one or two additional cooperating mutations are needed to generate a malignant founding clone.
Cancer is a genomic disease associated with gene mutations, resulting in a loss of control over vital cellular functions. Of all mutated genes, driver genes have been causally linked to oncogenesis, whereas passenger genes are believed to be irrelevant for tumorigenesis. Driver genes can be classified into 12 signaling pathways that regulate three core cellular processes: cell survival, cell fate, and genome maintenance (Table 2.4). Basic cancer research is needed to understand these pathways (Vogelstein et al. 2013).
Table 2.4
List of driver genes linked to cell survival, cell fate, and genome maintenance
Cell survival | MAP3K1 | ARID1B | NOTCH-2 |
ABL1 (PTK) | MED12 | ARID2 | PAX5 |
ACVR1B (ALK4) | MET | ASXL1 | PBRM1 |
AKT1 | MPL | ATRX | PHF6 |
ALK | MYD88 | AXIN1 | PRDM1 |
B2M | NF1 | BCOR | PTCH1 |
BCL2 | NFE2L2 | CDH1 | RNF43 |
BRAF | NPM1 | CREBBP | RUNX1 |
CARD11 | NRAS | CTNNB1 | SETD2 |
CASP8 | PDGFRA | DAXX | SETBP1 |
CBL | PIK3CA | DNMT1 | SF3B1 |
CDC73 | PIK3R1 | DNMT3A | SMARCA4 |
CDKN2A | PPP2R1A | EZH2 | SMARCB1 |
CEBPA | PTEN | FAM123B | SMO |
CIC | PTPN11 | FBXW7 | SPOP |
CRLF2 | RB1 | FOXL2 | SRSF2 |
CSF1R | RET | GATA1 | TET2 |
CYLD | SMAD2 | GATA2 | U2AF1 |
EGFR | SMAD4 | GATA3 | WT1 |
ERBB2 | SOCS1 | H3F3A | |
FGFR2 | SOX9 | HIST1H3B | Genome maintenance |
FLT3 | STK11 | HNF1A | ATM |
FUBP1 | TNFAIP3 (A20) | IDH1 | BAP1 |
GNA11 | TRAF7 | IDH2 | BRCA1 |
GNAQ | TP53 | KDM5C | BRCA2 |
GNAS | TSC1 | KDM6A | MLH1 |
HRAS | TSHR | KLF4 | MSH2 |
JAK1 | VHL | MEN1 | MSH6 |
JAK2 | MLL2 | STAG2 | |
JAK3 | Cell fate | MLL3 | |
Kit | APC | NCOR1 | |
KRAS | AR | NF2 | |
MAP2K1 | ARID1A | NOTCH-1 |
It is widely accepted that cancer is attributed to the accumulation of genetic aberrations in cells. Hence it is very important to understand the molecular mechanism of cancer progression to identify the genes whose alterations accumulate during cancer progression, as well as the genes that are responsible for the metastatic potential of cancer cells. Various stages of tumor progression have been analyzed, and these studies have revealed that alterations in oncogenes and in tumor suppressor genes accumulate and are responsible for the aggressiveness of cancer (Vogelstein et al. 2013).
Several oncogenes were identified in the early 1980s, which opened the way for the search for genetic alterations in human cancer cells (Wood et al. 2007; Parsons et al. 2008). This search was further aided by the isolation of tumor suppressor genes in the early 1980s and 1990s. In 1986, the first tumor suppressor gene, RB, was isolated from the human genome (Palles et al. 2013). After that, more than 20 tumor suppressor genes have been identified (Nowell 1976). Molecular analyses of these genes have confirmed that more than one tumor suppressor gene is involved in the progression of the tumor cell (Wood et al. 2007; Kinzler and Vogelstein 1997).
These molecular analyses indicated that genetic alterations occur in a stepwise manner during complete tumor progression. In the prospect, several extensive studies were conducted. Tumors from various types of cancers were analyzed to compare the genetic alterations appearing in the early and later stages of tumor development. In most cases, more mutations were found in later-stage tumors than in early stage tumors. These findings have been verified in several genetic models, including colorectal carcinoma models (Kerbel 1990; Bozic et al. 2010; Laurenti and Dick 2012).
In various kinds of human cancers, oncogene alterations have been investigated to determine their association with prognosis in cancer patients. For example, overexpression of the c-erbB-2 oncogene is a marker for aggressiveness in ovarian and breast cancers (Yan et al. 2009; Zhao et al. 2009).
Isolation of high and low metastatic subclones from a primary tumor has made it possible to elucidate and compare the properties of high and low metastatic cells. High and low metastatic cells were found to be different in many aspects. For example, various genes are differentially expressed in these two types of cells; specifically, several genes could induce metastasis whereas others could suppress it. Thus, by analyzing genotype-phenotype correlation with respect to metastatic potential, it should be possible to identify specific genetic alterations responsible for metastasis (Yokota 2000).
In solid tumors such as those derived from the colon, breast, brain, or pancreas, an average of 33–66 genes display subtle somatic mutations that would be expected to alter their protein products. About 95 % of these mutations are single-base substitutions (such as C > G), whereas the remaining are deletions or insertions of one or a few bases (such as CTT > CT). Of the base substitutions, 90.7 % result in missense changes, 7.6 % result in nonsense changes, and 1.7 % result in alterations of splice sites or untranslated regions immediately adjacent to the start and stop codons (Vogelstein et al. 2013).
Certain types of tumors display many more or fewer mutations than the average. Notable among these outliers are melanomas and lung tumors, which contain ~200 nonsynonymous mutations per tumor. These larger numbers reflect the involvement of potent mutagens (ultraviolet light in melanomas and cigarette smoke in lung tumors) in the pathogenesis of these tumor types. Accordingly, lung cancer specimens obtained from smokers have ten times as many somatic mutations as do those from nonsmokers (Govindan et al. 2012). Tumors with defects in DNA repair form another group of outliers (Gryfe and Gallinger 2001). For example, tumors with mismatch repair defects can harbor thousands of mutations, even more than lung tumors or melanomas. Recent studies have shown that high numbers of mutations are also found in tumors with genetic alterations of the proofreading domain of DNA polymerases POLE or POLD1 (Cancer Genome Atlas Network 2012; http://ecancer.org/news/3237-cancer-genome-atlas-network-maps-colorectal-cancer.php). At the other end of the spectrum, pediatric tumors and leukemia harbor far fewer point mutations: on average, 9.6 per tumor (Vogelstein et al. 2013).
Wide genomic aberration is a hallmark of genomes of all cancer types. Deep sequencing technology recently characterized the geographic and functional spectrum of cancer genomic aberrations and revealed insights into mutational mechanisms (Koboldt et al. 2012; Kidd et al. 2010; Beroukhim et al. 2010). These somatic mutations in cancer genomes may encompass several distinct classes of DNA sequence variations, including point mutations, copy number aberrations (CNA), and genomic rearrangements (Stratton et al. 2009). CNAs are deletions or additions of large segments of a genome and usually include one to tens of genes. Although these somatically acquired changes have been observed in cancer cell genomes, this does not necessarily mean that all abnormal genes are also involved in the development of cancers. Indeed, some genes are likely to make no contribution at all to cancer progression. To distinguish between them, these mutated genes have been coined driver and passenger genes (Stratton et al. 2009; Akavia et al. 2010). A driver gene is causally implicated in the process of oncogenesis, whereas a passenger gene makes no contribution to cancer development itself but is simply a by-product of the genomic instability observed in cancer genomes. Distinguishing driver genes from passenger genes has thus been considered an important goal of cancer genome analysis, especially in the field of personalized medicine and therapy (Santarius et al. 2010; Futreal et al. 2004; Chen et al. 2013).
It was discussed earlier that tumors in all types of cancers evolve through the process of tumor progression by acquiring a series of mutations over time (Nowell 1976; Fearon and Vogelstein 1990). The gatekeeper mutation provides normal epithelial cell, giving a selective growth advantage to outgrow the cells that surround it (Kinzler and Vogelstein 1997). Hence the mutations that confer a selective growth advantage to the tumor cell are called driver mutations (Bozic et al. 2010). The number of mutations in tumors of self-renewing tissues increases with age. It was found that more than half of the somatic mutations identified in such tumors occur during the preneoplastic phase, i.e., during the growth of normal cells. All of these preneoplastic mutations, termed passenger mutations, do not have any effect on the neoplastic process (Tomasetti et al. 2013). These findings explain why advanced-stage brain tumors and pancreatic cancers have fewer mutations than do colorectal tumors: the glial cells of the brain and epithelial cells of the pancreas do not replicate. Genomic sequencing of cancer cells from leukemia patients have shown that mutations occur as random events in normal precursor cells before the cells acquire an initiating mutation (Nowell 1976; Bozic et al. 2010).
So when do the remaining somatic mutations occur during tumorigenesis? A number of mutations in the progressive stages of colorectal and pancreatic cancers have been measured (Jones et al. 2008; Bernards and Weinberg 2002), and researchers concluded that it takes decades for a metastatic cancer to develop into a full-blown tumor. Furthermore, the mutations in metastatic lesions were already present in the cells of the primary tumors. It is possible that just as there are mutations that convert a normal cell to a benign cell or a benign tumor to a malignant tumor, there may be mutations responsible for converting a primary cancer to a metastatic one (Vogelstein et al. 2013). Advanced tumors release millions of cells each day into the circulatory system, but only few of them establish metastatic lesions. These circulating cells lodge in the capillary bed that provides the favorable environment for the growth of the metastatic cell (Yu et al. 2011).
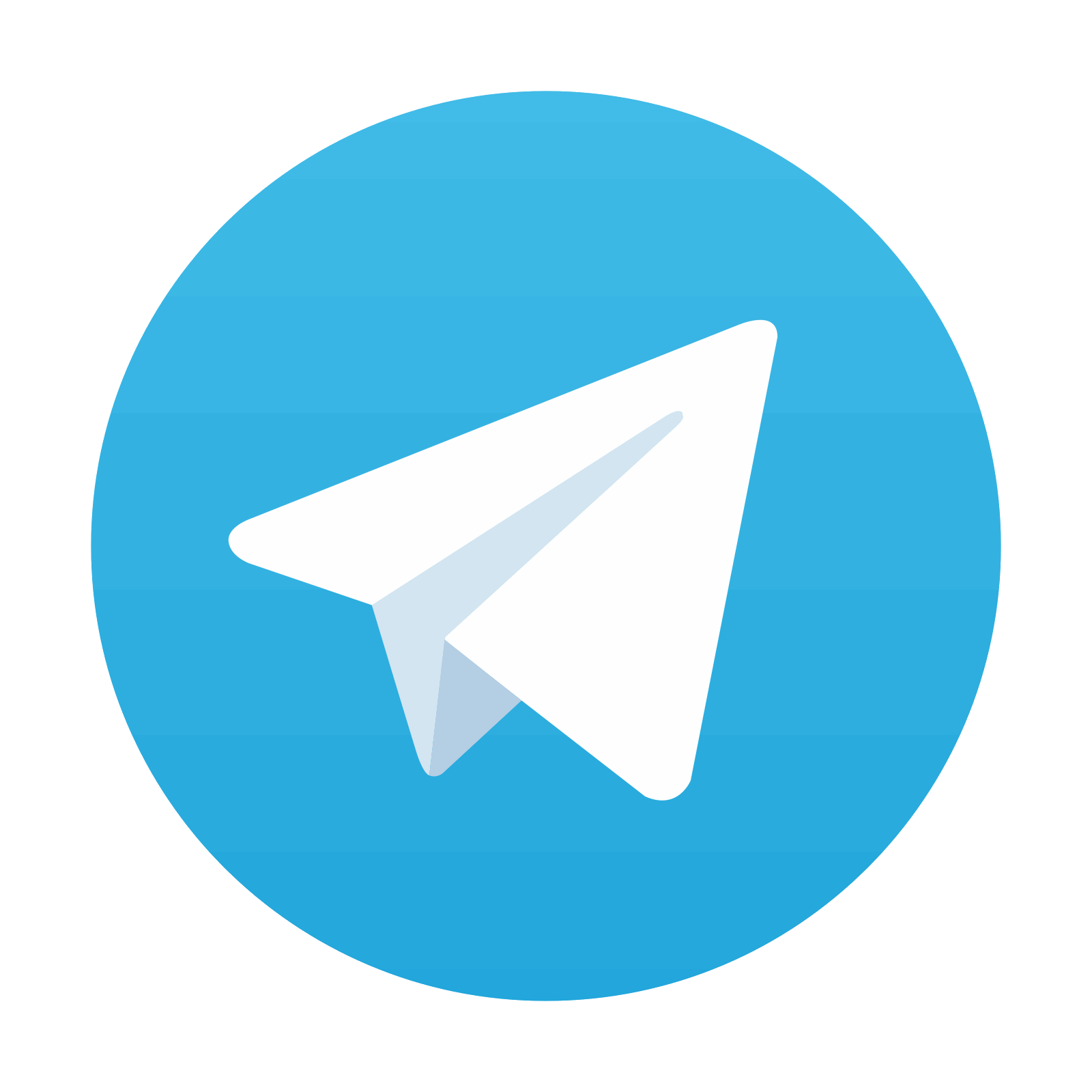
Stay updated, free articles. Join our Telegram channel

Full access? Get Clinical Tree
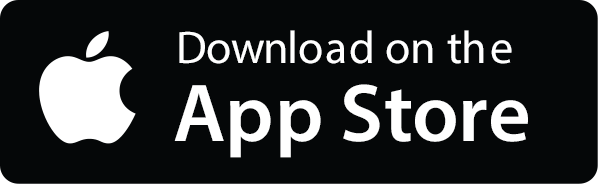
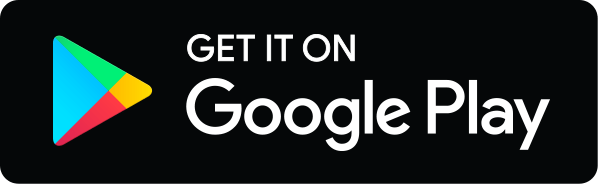