Introduction
Osteosarcopenia (OSP) is a geriatric syndrome characterized by systemic loss of bone (osteopenia/osteoporosis), muscle strength, and muscle mass (sarcopenia) . The age-related loss of bone and muscle tissues is known to be interconnected and usually occurs comorbidly. Low parameters of both lean mass and grip strength affect the bone stability and may cause the propensity to fall with a high fracture risk . Taking into account these two comorbid events, the diagnostics of osteosarcopenia is dichotomous and comprises the assessment of bone and muscle parameters, such as strength and quality . Bone mineral density (BMD) is one of the main markers of bone strength and can be easily measured by dual X-ray absorptiometry (DXA). By World Health Organization (WHO) definition, the “low bone mass” is considered as osteopenia when the T-score of BMD is between − 1 and − 2.5 SD from a young adult reference population, therefore for more severe condition—osteoporosis—BMD lower than − 2.5 SD is a diagnostic criterion . The assessment of muscle health is more complex and requires multistep-based diagnostic strategies . According to the European Working Group of Sarcopenia in Older People (EWGSOP), the sarcopenia (low muscle mass) diagnostic procedure has to be built on muscle strength assessment and characterization of muscle quality. Later on, in 2019 EWGSOP2 working group provided an algorithm to diagnose and quantify the severity of sarcopenia . Muscle quantity can be measured by DXA, bioelectric impedance analysis (BIA), computed tomography, and magnetic resonance imaging (MRI); muscle strength by grip strength and chair stand test. If the sarcopenia is confirmed, its severity can be estimated by physical performance tests, such as gait, speed, short physical performance battery (SPPB), etc. Taken together if the patient is diagnosed with both osteoporosis and sarcopenia, it is considered an osteosarcopenic condition. Currently, therapeutic options for preventing osteosarcopenia are limited and mostly based on nonpharmacological therapies with lifestyle modifications including regular exercising and nutritional strategies. A further search of pharmacological agents preserving both the strength and the quality of bone and muscles is required .
Heritability of musculoskeletal traits
In investigating the genetic associations with osteosarcopenia the understanding of the musculoskeletal phenotypic heritability ( h 2 ) is necessary. Heritability is a proportion of measured variance in the trait of interest determined by genetic factors; it ranges in value from 0 to 1. Heritability can be defined, as “broad-sense” or “narrow-sense”: broad-sense heritability comprises all genetic influences on variation, such as epistasis, dominance, gene-environmental interaction; the “narrow-sense” heritability includes just additive genetic variance. Traditionally the heritability of traits can be estimated by genetic similarity within the family and twin data; however, it is also possible to be extracted from genome-wide SNP data of unrelated individuals .
Since in 1973 Smith et al. demonstrated the significant contribution of genetic factors on bone mass of forearm, numerous heritability studies revealed the genetic contribution for different quantitative bone parameters, such as BMD of spine and hip, bone size, which confirmed them as high heritable traits ( h 2 = 0.5 to 0.8) . Similar to bone traits, it has been shown that muscle traits such as hand-grip strength are also highly heritable ( h 2 = 30%–52%). Twin studies showed the additive genetic correlation ( ρ g ) between lean mass and BMD within the range from 30% to 45% and in pediatric cohorts, a shared genetic component was shown around 43% . Altogether, this shared significant correlation between bone and muscle traits triggered further studies of pleiotropy .
Genome-wide association studies
The pursuit of the genetic determinants of common phenotypes is essentially a quest for better prevention and treatment of heritable diseases. Genome-wide association studies (GWAS) are a relatively new way but have been instrumental in identifying genomic regions that harbor genetic determinants of human diseases or traits including bone and muscle. This approach equals a hypothesis-free evaluation to scan DNA sequence variation, specifically the single nucleotide polymorphisms (SNPs), across the human genome, evaluating their association with the traits of interest. For over a decade, GWAS have reported an impressive number of reproducible genomic regions associated with various common traits. To date, the NHGRI-EBI GWAS Catalog has documented more than 71,673 variant-trait associations. The clinical utility from this success in GWAS may lie in many aspects, such as improving the disease prediction, better understanding response to treatment, and developing more personalized approaches to disease management.
Univariate GWAS
Over a decade since the first GWAS emerged, GWAS have provided numerous compelling genetic associations for human complex traits including musculoskeletal disorders. Specifically, by 2018 GWAS have identified more than 100 loci which are associated with DXA-derived BMD at the femoral neck, lumbar spine, or total body BMD . In addition, more than 300 novel loci which are associated with estimated BMD based on heel ultrasound were added in 2019, by leveraging the large collection of the sample from UK Biobank . On the other hand, relatively fewer GWAS efforts have been devoted to muscle-related traits, such as total body lean mass, appendicular lean mass, and handgrip strength. Specifically, more than 200 loci have been associated with these muscle-related traits .
Common genetic loci are observed to be associated with both bone and muscle, indicating the potential pleiotropy, which occurs when one locus affects multiple traits. Pleiotropy, either horizontal, vertical, or spurious (defined below), may result in genetic correlations . Traditionally, the calculation of genetic correlation ( ρ g ) requires the collection of family samples, and researchers can estimate ρ g based on resemblance among family members. Recent development in cross-trait linkage disequilibrium (LD) score regression provides an alternative way to estimate genetic correlation by leveraging GWAS association summary statistics . Although genetic correlation may mainly result from pleiotropy, vanished genetic correlation between two traits does not exclude the possibility of pleiotropy . Intuitively, the overall genetic correlation can be considered as the averaged effect of genetic factors across the genome, which may include positive pleiotropy or negative pleiotropy at different loci . Studying genetically correlated traits may offer insight into complex disease-related traits and lead to tremendous clinical implications because they may reveal genetic variation that contributes to both diseases.
GWAS uncover genetic determinants across the genome in an unbiased way, which implies that GWAS may uncover previously unsuspected, yet important loci that could be useful for further analysis. With this property, GWAS offer the opportunity for researchers to evaluate the commonly shared genetic determinants between bone and muscle. Several genomic loci identified in GWAS of bone-related traits have been also found to be associated with muscle-related traits . Cross-trait association, which searches for the significant association with another trait for the loci associated with the trait of interest, may be the simplest and most intuitive approach to investigate cross-trait effect. However, such an ad hoc cross-trait association approach can be underpowered . Besides, such screening will severely depend on the LD structure and may more likely identify spurious pleiotropy.
Bivariate GWAS and co-localization
Multivariate statistical approaches have direct applications in the analysis of multiple phenotypes. Many approaches have been proposed beyond the classic multivariate methods. For example, based on generalized estimating equations, Lange et al. proposed a multivariate extension of the family-based association test to model multiple phenotypes. Zhang et al. presented a nonparametric approach to testing the association for multiple traits together based on the generalized Kendall’s Tau. Ray et al. and Deng et al. constructed a unified score-based association test for detecting pleiotropy that combines multivariate analysis of variance (MANOVA) and the sum of squared score (SSU) test, while Hartley et al. modeled the pleiotropic association with Bayesian networks. These multivariate approaches can jointly model multiple traits under a certain statistical framework to increase the power compared to each single trait analyzed separately. However, through using the individual-level data, these approaches require the phenotypic and genotypic information from all or majority of the participants, ignoring the existing resources generated by large-scale trait-specific GWAS.
GWAS conventionally scans the DNA genome for the association with one trait at a time; however, recently, researchers have advanced the approach to leverage the single-trait derived association summary statistics and jointly examine the association with multiple traits. Besides, compared to directly modeling multiple phenotypes simultaneously, utilizing GWAS summary statistics involves less assumption about the relationship among the individual phenotypes. Therefore, such a combined summary statistic approach is instrumental to identify pleiotropic loci associated with multiple traits. For example, motivated by stratified false discovery rate (FDR) methods, a genetic pleiotropy-informed conditional FDR (cFDR) method was proposed to identify the SNPs associated with multiple traits by applying this method to GWAS summary statistics data in order to minimize the association driven by a single phenotype. Intuitively, it is more likely to observe smaller P -values for the association with one trait of interest for the subsets of SNPs determined by the nominal P -values of their associations with another trait of interest being as small or smaller than a given threshold if there is a pleiotropic effect for the traits of interest. Therefore, the authors calculated conjunction FDR for the identification of pleiotropy, which is defined as the posterior probability to obtain the P -values for all the traits simultaneously as small or smaller than the observed P -values under the assumption of null association. This approach does not take the association effect into consideration.
Yang et al. proposed the extension of O’Brien’s statistics by sample splitting or cross-validation method to gain the power in the scenario with the potentially heterogeneous effect of association with some computing expense for cross-validation method. However, there is one downside for Yang’s approach requiring individual-level data to estimate the optimal weight of association, limiting its application. Therefore, the data-driven empirical linear combining approach (eLC) was proposed to overcome this limitation by combining correlated test statistics . The eLC approach applied permutation techniques to construct the empirical null distribution of test statistics, and empirically optimize the overall association signals while accounting for the association between phenotypes. Similar to Yang et al.’s approach, the eLC also allows for mixed directions of effects. There are many other methods to identify multivariate phenotypes simultaneously by leveraging the GWAS summary statistics, such as meta-SCOPA, metaUSAT, and so on .
The aforementioned bivariate analysis intends to identify genetic variants that are associated with multiple phenotypes. However, the underlying mechanism of interrelationship among the traits of interest and identified genetic variants remains unknown. The pleiotropy relationship between two traits can be classified into different categories: (1) biological pleiotropy or horizontal pleiotropy where the SNP affects both traits through different paths; (2) mediated or vertical pleiotropy where the SNP affects one of the traits, which lies on the causal path to another trait; and (3) spurious pleiotropy where the identified SNP is in LD with two different—not colocalized—causal variants (even different genes) that affect different traits, respectively. Spurious pleiotropy is usually less of interest. However, the other two pleiotropy phenomena have attracted much attention. For example, Phenome-wide association (PheWAS) studies have been conducted to explore the biological pleiotropy, and the Mendelian randomization approach has been widely used to delineate the causal relationship of traits in the vertical pleiotropy. Along the same line, some researchers have also explored pleiotropy with polygenic risk scores (PRS ). These approaches have been proven to be a useful tool to shed the light to usher the new discoveries which require experimental validation eventually. It is obvious that with the extension of current bivariate applications to really multivariate ones (e.g., combining BMD, lean mass, and grip strength), the scenarios—increasing the number of hypotheses regarding interrelationship among identified genetic variants and our traits of interest—will become even more convoluted, possibly affecting the interpretability .
Current status of OSP-related genetics in humans
During the last decade, multiple tools were developed to analyze the pleiotropic regulation of complex traits and to shed light on genetic interaction between comorbid diseases. However, only a few studies were done for musculoskeletal traits. For example, a bivariate GWAS study for bone size phenotypes (hip bone size and appendicular lean mass identified variants mapped near GLYAT gene . Bivariate GWAS metaanalysis of pediatric musculoskeletal traits revealed variants with pleiotropic effect in eight loci. Seven loci: WNT4, GALNT3, MEPE, CPED1/WNT16, TNFSF11, RIN3 , and PPP6R3/LRP5 are established BMD loci . The variants in the eighth—novel—locus ( TOM1L2/SREBF1 ) demonstrated the opposing effect on total-body lean mass and total-body BMD. In addition to that, SREBF1 was pointed out as a strong candidate driving the correlation between two traits. It was shown that SREBF1 is highly expressed during osteoblasts mineralization in human MSCs. Also, SREBP1 protein regulates myogenesis via indirect inhibition of MYOD1, MYOG, and MEF2C .
OSP genetics might reflect shared mechanisms underlying MSK-related comorbidity and the relationship between anthropometry and metabolic traits, and even neurodegenerative diseases (especially if a neuromuscular junction is involved . Identification of a candidate gene requires additional experimental validation to establish causality, for example, by validating candidate genes with gene-editing technologies in the cell cultures or model organisms. Therefore, we focus here only on several genes, which have been validated in animal models or human cells .
Candidate genes
In an early study, by combining the data from the inbred strains of mice with the human GWAS data from the GEFOS consortium, we were able to narrow a quantitative trait locus (QTL) on the mouse Chr 15—which contained 131 annotated genes—to one gene, Trps1 (then called “trichorhinophalangeal syndrome I” gene). In humans, TRPS1 SNPs were significantly associated with femoral neck BMD. Therefore, combining association studies in humans with murine models may bear fruits. At the same time, it was demonstrated that TRPS1 is a target for miR-133a/b during chondrogenesis and underlies a QTL for carcass weight and muscle area in Korean Cattle . More recently, TRPS1 was identified among potentially pleiotropic loci for bone and obesity metabolism , lean mass , and handgrip strength , again supporting its possible involvement with body weight and muscle properties.
The METTL21 protein family has been implicated in the pathogenesis of some muscle (e.g., inclusion Body Myositis) and bone (Paget’s) diseases . In a bivariate (muscle-bone) GWAS study of the Framingham Study, we identified an intronic SNP in METTL21C . Functional validation was performed in both C2C12 myoblasts and MLO-Y4 osteocyte cells, and the consequences in both were remarkable. It was further shown that ablation of the Mettl21c reduces endurance capacity and leads to age-dependent accumulation of autophagic vacuoles in skeletal muscles . METTL21C mediates the trimethylation and stabilizes the heat shock protein Hspa8/Hsp70, a chaperone required for protein import into mitochondria . A recent study identifies that Mettl21c is a type I myofiber specific protein, and functions to tri-methylate valosin-containing protein (Vcp/p97), a protein required for autophagy. VCP in turn affects both muscle and bone , as its defect is associated with Inclusion Body Myopathy and Paget Disease of Bone.
In Table 1 , we provide the existing mouse KO models from the Mouse Genome Informatics database for GWAS-derived candidate genes associated with musculoskeletal phenotypes in humans. The annotated bone and or muscle phenotypes for these mutants are highlighted. For some candidates, as GALNT3 the mutant murine model is annotated with alteration in both bone and muscle parameters. However, for the majority of candidates, the mutants display either bone or muscle phenotype. Similarly, to date, Srebf1 tm2Jdh /Srebf1 tm2Jdh mouse mutant line is annotated just with decreased levels of triglyceride and cholesterol while MSK phenotypes are not reported.
Gene | Animal model | Bone phenotype | Muscle phenotype | Mouse Genome Informatics database |
---|---|---|---|---|
WNT16 (wingless-type MMTV integration site family, member 16) | Wnt16 tm1Lex /Wnt16 tm1Lex | Decreased cortical bone volume | No data | http://www.informatics.jax.org/marker/phenotypes/MGI:2136018 |
LRP5 (low density lipoprotein receptor-related protein 5) | Lrp5 Gt(OST164883)Lex /Lrp5 Gt(OST164883)Lex | Abnormal bone morphology, decreased BMD | No data | http://www.informatics.jax.org/marker/phenotypes/MGI:1278315 |
MC4R (melanocortin 4 receptor) | Mc4r tm1.1Fbz /Mc4r tm1.1Fbz | No data | Altered lean body mass | http://www.informatics.jax.org/marker/phenotypes/MGI:99457 |
Mc4r tm1Dhu /Mc4r tm1Dhu | Decreased osteoclast cell number, increased BMD | No data | ||
TRPS1 (transcriptional repressor GATA binding 1) | Trps1 tm1.1Shiv /Trps1 tm1.1Shiv | Abnormal rib formation, thorax cage, scoliosis | No data | http://www.informatics.jax.org/marker/phenotypes/MGI:1927616 |
WNT4 (wingless-type MMTV integration site family, member 4) | Gt(ROSA)26Sor tm1(Wnt4)Bhr | Shortened limbs; abnormal axial skeleton | No data | http://www.informatics.jax.org/marker/phenotypes/MGI:98957 |
GALNT3 (polypeptide N-acetylgalactosaminyltransferase 3) | Galnt3 tcal /Galnt3 tcal | Increased BMD | Calcification of muscles | http://www.informatics.jax.org/marker/phenotypes/MGI:894695 |
MEPE (matrix extracellular phosphoglycoprotein with ASARM motif (bone)) | Mepe tm1Tbrw /Mepe tm1Tbrw | Abnormal skeleton morphology; increased BMD | No data | http://www.informatics.jax.org/marker/phenotypes/MGI:2137384 |
METTL21C (methyltransferase like 21C) | Mettl21c tm1.1Krug /Mettl21c tm1.1Krug | No data | Abnormal muscle physiology; impaired exercise endurance; muscle weakness | http://www.informatics.jax.org/marker/phenotypes/MGI:3611450 |
TNFSF11 (tumor necrosis factor (ligand) superfamily, member 11) | Tg(APCS-Tnfsf11*)#aKhs/0 C57BL/6 J-Tg(APCS-Tnfsf11*)#aKhs | Decreased BMD; osteoporotic phenotype | No data | http://www.informatics.jax.org/marker/phenotypes/MGI:1100089 |
Tnfsf11 gum /Tnfsf11 gum | Osteopetrosis; small cranium; mandible hypoplasia | Dystrophic muscle; decreased lean body mass | ||
SREBF1 (sterol regulatory element binding transcription factor 1) | Srebf1 tm2Jdh /Srebf1 tm2Jdh | No data | No data | http://www.informatics.jax.org/marker/phenotypes/MGI:107606 |
TOM1L2 (target of myb1-like 2) | Tom1l2 Gt(XG909)Byg /Tom1l2 + | Malocclusion; kyphosis | No data | http://www.informatics.jax.org/marker/phenotypes/MGI:2443306 |
Rare conditions/monogenic diseases
Rare monogenic conditions also known as Mendelian diseases provide a good source of gene candidates and pathways they are involved in Refs. . There is no conceptual dichotomy between common and rare diseases if a causal variant is known (usually common for the former and less common for the latter). Muscular dystrophies are known to affect the skeletal system as well . The most prominent is Duchenne Muscular Dystrophy (DMD) and Becker Muscular Dystrophy, where muscle impairment is accompanied by low BMD or increased fracture propensity (reviewed in Ref. ). Both are associated with the dystrophin (DMD) locus on the X chromosome.
Fibrodysplasia ossificans progressiva (FOP) is a rare autosomal dominant disease, which is caused by a mutation in ACVR1 (activin a receptor, Type I) gene. It is characterized by extraosseous ossification which does not only affect muscles, but also ligaments, tendons, and connective tissue leading to severe restriction of movements. Also, skeletal muscle myopathies, characterized by various patterns of weakness and dysfunction, sometimes also affect bones. For example, a mutation in methylthioadenosine phosphorylase (MTAP) gene causes an autosomal dominant bone dysplasia, along with a limb-girdle myopathy; there might be both muscle weakness and atrophy and pathologic fractures due to abnormal cortical growth. Congenital generalized lipodystrophy type 4 has prominent muscular dystrophy, probably due to mutated Caveolae Associated Protein 1 (CAVIN1, a.k.a. Polymerase I and Transcript Release Factor). This recessive disorder also is characterized by impaired bone formation, lordosis, or scoliosis. Also, inclusion body myopathy associated with Paget’s disease, which seems to be caused by a defect in valosin-containing protein, VCP , affects both muscle and bone.
By perusing the OMIM database with a search keyword: “sarcopenia,” one finds several additional diseases and genes, such as ERCC1 (excision repair, complementing defective); this gene is also associated with “estimated BMD” (eBMD ). Of note, this gene is located on 19q13.32 near the APOE gene, raising the likely possibility that these associations reflect the influence of variants in that well-established longevity-associated locus . Also, POLG (polymerase, DNA, gamma) was associated with eBMD . The PolgA (D257A/D257A) mice were described above. A gene responsible for spinal muscular atrophy with congenital bone fractures 1, TRIP4 (thyroid hormone receptor interactor 4), was similarly associated with heel BMD .
These disease-related genes provide new insights into biological pathways that can become the target of intervention for more complex musculoskeletal conditions like osteosarcopenia . Some of the persons with the rare musculoskeletal conditions if their symptoms are mild might be misclassified as “normal” (so-called “phenocopies”) and therefore to be included in the population- based GWAS. However, it is difficult to imagine anyone who survives for a long time without a correct diagnosis to make it to the older-age GWAS effort.
Effect of environment on musculoskeletal quality and tissue-specific aging
Different tissues of the human body undergo DNA methylation during the lifespan and musculoskeletal tissues are not an exception . The effect of tissue-environment interactions on tissue quality and its decline with age is measurable. For example, an epigenetic clock—a mathematical algorithm—was established to predict the biological age of tissue, based on DNA methylation level . Specifically for the musculoskeletal application, the epigenetic clock may help to evaluate the effect of environmental factors, such as exercise and diet on the biological aging of muscles and bones. Multiple studies in humans and animals (even in the small fish models) have explored that exercise promotes the gain in muscle as well as bone mass, thus preventing musculoskeletal degradation . Within the human population, the regularly exercising cohort of people are usually athletes, dancers, and soldiers . Moreover, it was shown that retired athletes and dancers after cessation of high physical exercising still have higher BMD than people with a sedentary lifestyle . Therefore, people with a physically active lifestyle are more protected against impaired musculoskeletal conditions. The mechanical loading on the skeletal system induces the process of bone remodeling, by increasing the number of bone-forming cells, BMD and bone volume; however, the continuous loading can lead to impaired energy availability and increase the (stress) fracture risk . Further GWAS studies of elite athletes, who endure much higher mechanical stress than most of the people, can elucidate the genetic peculiarities underlying the musculoskeletal abilities of such an extreme population . As an example, a candidate gene ACTN3 came out from such studies and further was confirmed as a regulator of muscle and bone phenotype in the mouse model . In addition, the SNP polymorphism in ACTN3 appears to influence muscle phenotype in elite athletes , BMD, muscle impairment, and metabolic disorder risk in older people . Also, the polymorphism in VDR was shown to be associated with higher BMD in weight-bearing athletes compared to the control group . However, at the present, both these genes have not been replicated in extant GWAS for musculoskeletal traits. This might mean that either they are not as relevant for the majority of the population or that GWAS should be attempted in a case-control fashion, e.g., comparing athletes with nonexercising adults.
Besides physical activity, there are still many other factors affecting musculoskeletal homeostasis. For example, insufficient protein intake, low vitamin D level, and calcium deficiency may substantially affect the quality of bone and muscle tissues . The loss of each or both tissues may appear subsequently as a result of behavioral influences, including a balance of activity and resting (including sleeping ). It is known that physiology and behavior are rhythmically orchestrated by circadian rhythms. The disruption of circadian rhythms in humans may change the DNA methylation of clock genes in key metabolic tissues, such as skeletal muscles, thereby altering their epigenetic age . A recent study had shown that muscle-specific disruption of core molecular clock protein BMAL1 significantly affects muscle/bone metabolism in a paracrine manner . The microarray analysis of BmaL1 muscle-specific KO in mice revealed the significant expression change of myokines involved in bone formation/resorption processes, such as myostatin, TGFB1, IGFB5, irisin, and GDF11 . These findings hint at a possible direction for further discovery of new pathways controlling these relationships which may lead to intervention strategies directed to alter clock genes in muscles.
Pleiotropy for bone-and-muscle-related phenotypes in animals
Animals have been used to identify genes underlying bone-and-muscle traits; the most prominent animal models are rodent, ovine, porcine, and piscine . The International Knockout Mouse Consortium and the International Mouse Phenotyping Consortium generated a wealth of genetic information on MSK phenotypes . The advantages of these animal models are numerous, including controlled genetic background and testing of advanced phenotypes (e.g., biomechanical properties) that cannot be assessed in humans in vivo. Models of co-occurring sarcopenia and osteoporosis exist, usually in senescence-accelerated mice . Strategies for mapping of genes in mice traditionally relied largely on intercross or backcross approaches or the use of recombinant inbred lines, to facilitate the forward genetic discovery. For example, in advanced intercross lines (AIL) of mice, Gonzales et al. identified 126 significant associations for 50 traits, including bone length and muscle mass. Also, in mice, the investigators explored the QTL intervals to identify genes that potentially affect muscle mass and bone length; they revealed human-mouse genetic convergence specifically for the myogenesis loci (see also Ref. for human muscle regulation).
GWAS is widely used in farmed animals, e.g., cattle and other livestock, for MSK traits . Many putatively pleiotropic QTLs affecting traits of biomedical importance have been discovered in farmed animals , although the aims of the discovery were obviously of agricultural interest. The animal genetic terminology differs somehow, but in the essence, traits such as “bony carcass” or “body frame size” refer to bone-and-muscle-related phenotypes. For example, a recent GWAS identified an SNP within the diacylglycerol O -acyltransferase 1 ( DGAT1 ) gene that was significantly associated with the “quality” of long bones . Besides being pleiotropic for multiple bovine traits, DGAT1 was shown to function in rodent skeletal muscle cells . In humans, SNP rs7838717 in the DGAT1 locus was associated with Dupuytren’s disease .
Dogs and horses continue to serve as best helpers for humans also for genome-mapping purposes. Musculoskeletal phenotypes were studied in the domestic dog , given this species amenability for morphological genetic study . Similarly, although muscle performance is the most studied trait in the thoroughbred racing horse, it was realized that susceptibility to fracture risk is also genetically-determined . In thoroughbreds, among regions selected for inbreeding using the pan-genomic SNP-based genotypes, genes relating to musculoskeletal form and function were identified . These include the deleted lymphocytic leukemia 7 gene ( DLEU7 ) associated with growth traits and eBMD in humans . The other was the von Willebrand factor C domain containing 2 gene ( VWC2 ), a bone morphogenic protein, which promotes bone formation, regeneration, and healing .
Forward genetics is also feasible in a small teleost, the zebrafish ( Danio rerio ) . Screens for MSK phenotypes were performed by Harris’ group to identify genes of interest for skeletogenesis. D. rerio is also helpful to validate human-first GWAS discovery (reverse genetics) . However, a lion’s share of such studies is still done in mice. Relevant to the GWAS of MSK phenotype, Tanaka et al. recently noted that human polymorphisms in FAM210a were strongly associated with fracture risk and BMD, as well as also nominally associated with lean mass. The authors created genetically modified mice; they showed that Fam210a was expressed in muscle mitochondria and cytoplasm but not in bone. Notably, grip strength and limb lean mass were reduced in both tamoxifen-inducible Fam210a homozygous global knockout mice ( TFam210a −/− ) and skeletal muscle cell-specific knockout mice ( Fam210aMus −/− ). In parallel, decreased BMD, bone biomechanical strength and bone formation, and elevated osteoclast activity were observed in TFam210a −/− mice . Therefore, Fam210a seems to play an important role in bone quality and quantity, as well as in muscle bulk and power.
More recently, Scheuren et al. aimed to evaluate the PolgA (D257A/D257A) mouse, which due to elevated mitochondrial DNA point mutations and systemic mitochondrial dysfunction, exhibits an accelerated aging phenotype, as a model of frailty and osteosarcopenia. Longitudinal assessment of the PolgA (D257A/D257A) mice displayed progressive MSK deterioration such as reduced bone and muscle mass as well as impaired MSK function. Specifically, PolgA (D257A/D257A) had lower grip strength and concentric muscle forces as well as reduced bone turnover. In addition, PolgA (D257A/D257A) mutation altered the sensitivity to anabolic stimuli in skeletal muscle, muscle progenitors, and bone. While compared to WT, PolgA (D257A/D257A) caudal vertebrae were not responsive to a cyclic loading regime. Overall, PolgA (D257A/D257A) mutation leads to hallmarks of age-related frailty and osteosarcopenia and, thus, provides a powerful model to better understand the relationship between frailty and the OSP for human study.
Animal models of bone-muscle interaction are frequently using mechanobiology approaches, with the loading of both muscles/bones or artificial immobilization and disuse. Relevant for the MSK regeneration in older age would be a study of hibernating mammals . There is a diverse array of species that may conserve muscle mass despite prolonged inactivity. It is interesting to compare them to the artificial immobilization/prolonged disuse in nonhibernating mammals, as a source of genetic factors responsible for musculoskeletal regeneration and maintenance .
In summary, this is an exciting time for the construction of an extensive catalog of a range of model species, of a variety of genomes and conserved genetic loci, focused on the osteosarcopenia-relevant mechanisms.
Sex-specificity of disease: Is there any genetic predisposition?
Osteosarcopenia’s definition (thresholds) might seem arbitrary; however, the difference between the sexes exists in OSP occurrence. It means that sex-specific genetics has to be tested. Recently, the role of sex in the genomics of human complex traits was well described . Such body-composition-related phenotypes as BMD, lean mass, and body shape demonstrate an evident sexual dimorphism . Therefore, the Genetic Investigation of Anthropometric Traits (GIANT) Consortium performed a metaanalysis in order to study the BMI and waist-to-hip ratio (WHR), with sex-specific genetic effects (G x sex) modeled . No sex-dependent effects were identified for BMI. However, for WHR they identified 44 sex-specific loci, 11 of which showed opposite effects between sexes . This study’s findings of sexual dimorphism in body shape are important for the OSP, since it might partly explain the male-to-female differences in the OSP prevalence.
There is mounting evidence that all adult tissues exhibit sex-specific expression patterns, some of which are independent of hormonal influence . Sexually dimorphic differences in gene expression across adult tissues may be modulated by sex‐specific cis‐regulatory elements, alternative splicing, and differentially methylated sites. This calls for a transcriptome-wide study with sex interaction terms.
One might assume that for sex dimorphic traits, sex differences may be simply explained by differences in the sex chromosome. However, Traglia and colleagues did not find a consistent disproportionate role for the X-chromosome in disease risk, despite sex-heterogeneity on the X for several anthropometric traits. Here to remind that DMD and AR genes are mapped on the X chromosome, as well as FAM9B— the latter associated with testosterone concentrations and BMD . Unfortunately, X-chromosome was not systematically studied during the first GWAS decade, thus even metaanalysis of age at menopause included only autosomal SNPs.
Sex chromosomes start to program autosomal gene expression and establish sex-specific epigenetic patterns very soon after fertilization . Differences in the male and female epigenomes include distinct methylated patterns such as imprinting and result in differential gene expression . As such, the presence or absence of the Y‐linked SRY locus is noted in the early fetus. Then, the life-long sex-specific environment seems to play a role in changing genotype expression across a range of human complex traits. The role of sex steroids and sex steroid receptors (e.g., ESRs and AR) is well known both for BMD and muscle mass regulation. Testosterone is an androgen, which is produced in the fetus and controls the sexual fate of the early gonads. Of note, myostatin is an androgen target gene in skeletal muscle .
For those traits where sexual dimorphism is obvious, sex-specific statistical approaches may aid in future gene-finding efforts . However, some predictions show that for most of the traits the number of sex-specific genetic variants will be small . Furthermore, this may be a challenging endeavor due to the likely small effects of the interactions at individual loci. In an earlier study, we suggested that gene-by-sex interactions probably have weak effects on BMD, accounting for less than 0.08% of the BMD variation per implicated SNP .
The presence of sex-specific effects would require sex-stratified analysis, as well as the study of enrichment in sex-hormone-related functional categories. If successful, sex-specific approaches may aid in future gene-finding efforts. Then, in clinical practice, sex-specific diagnostic markers or targeted treatments would be warranted.
Translational perspectives
Genetic discoveries are important for drug target identification and repurposing; pharmacogenetics is also helpful in assigning a drug to a disease. This is especially true for the loci with the opposite effect in bone vs. muscle that become a target for a drug: a lack of pleiotropic effects from such analysis supports their safety and efficacy.
Clinical applications might be possible for a genetic risk score (GRS, a.k.a. polygenic risk score, PRS). Whether GRS based on pleiotropic signals have more effective prediction is still unclear. However, it was shown that PRS might serve as a genetic instrument for MR analyses. Results of a preliminary simulation study indicated that, although using a PRS provides higher statistical power, it also suffers from substantive false positive rates due to horizontal pleiotropy (defined above). A single SNP which is known to be pleiotropic with large effects on different and diverse traits had been found to distort findings from PRS analyses .
Challenges and solutions
The complex and pleiotropic genetic architecture of MSK disorders poses some challenges for work in model systems as we discussed above. The absence of a clear-cut definition or diagnosis of OSP instructs us to use multivariate and bivariate statistics. It is important to take into consideration that osteosarcopenia, as a dichotomous phenotype, is useful for clinical use; however, for the study of genetics this is not optimal, commanding the investigators to keep looking for additional (endo)phenotypes. The presence of pleiotropy implies the need for a search of a particular endophenotype that mediates the effect of risk on the clinical phenotype of OSP. There is also a need in the phenotypes of “change,” to capture a trajectory of bone and muscle loss. Ideally, a longitudinal study should be a good answer, paralleled by (multi)omics data, to reflect an individual rate of bone and muscle loss and to test whether a combination of omics data correlates with this trajectory rather than a one-time measure of OSP. This corresponds to the logistical challenge to generate gene expression data for muscle and bone of the same individual (although material left over from the surgical procedures can be a good source, for example, from the hip replacement operations).
A substrate of metabolic communications between bone and muscle can be pointed out by the cross-phenotype study. The ensuing issue would then be a cell(s) with regulatory potential—whose origin can be either in bone or in muscle; the answer for this question is important to correctly allocate the resources for a functional study following the genomic approaches . Again, Big Data/omics should help identify cell(s) of origin for these molecules. They might be neither bone nor muscle, but some other organs/tissues (e.g., liver, adipose).
Furthermore, the effects of relevant variants are often found to be environment-specific and to have different temporal patterns . This necessitates a conduct of age-dependent time-to-event analyses to investigate temporal patterns of the genetic effects, where age can be considered an environment and used to stratify the population accordingly. A related problem is the lack of GWAS specifically focused on the aged population—or trying to analyze mid- to older persons transition or to model age × gene interaction. To date, animal genetic models as well as orchid- or ovariectomy models exist; however, they are insufficient to model the organism’s aging in its entirety.
It was proposed that a pleiotropic analysis combined with annotation with various expression and regulatory database resources can serve as a substantive tool to elucidate a causality behind the association between SNPs and diseases .
Detecting pleiotropic effects is just the 1st step. After the initial discovery, replication is a must : the ability to reproduce results is a cornerstone of science, especially due to the exponential growth of the amount of scientific data . Frequently the inability to reproduce findings is seriously dampening chances of correct applications. A “triangulation” approach is a gold standard in the field—where human discovery is followed by functional analysis with various means—e.g., cells and animal models. This is where the wealth of (multi)-omics and biology/laboratory follow-up is required.
Summary and future directions
With prolonged lifespan, the importance of quality of life in older persons is growing exponentially. Even though there is still an uncertainty in the definition of OSP, it is clear that this aging-related condition comprises the impairment of bones and muscles that coincide. Genome-wide association studies substantially contributed to our understanding of the genetic architecture of osteoporosis; however, there are still gaps in genetic insight into the sarcopenia phenotypes, either muscle mass or strength. Univariate GWAS is therefore still of value. The bi- and multivariate analyses are expected to contribute to additional discoveries of metabolic communications between bone and muscle, beyond biomechanical links between these tissues.
Recent GWAS findings produced large numbers of candidates with possible functions in musculoskeletal homeostasis, demonstrating the benefit of synthesizing data on related traits to uncover potential new disease-associated loci. This search of candidate genes with possible pleiotropic effects on bones and muscles successfully reveals numerous candidates that have to be further validated. Some of these candidates have been examined in human cells and animal models, exemplified by FAM210A, SREBF1, METTL21C, and other genes. However, to evaluate the genetic landscape of OSP, further GWAS for osteoporosis and sarcopenia in the older population are necessary in order to decipher the genetic contribution of senescence-related factors. In addition, the implementation of sex-specific approaches could shed the light on the pathogenesis of OSP, which seems to have different etiology in men and women. Furthermore, retired athletes, dancers, and people with active physical backgrounds with nontraumatic experience in the past display a better quality of MSK parameters compared to people with a sedentary lifestyle. Together with experimental studies of exercising impact on MSK system in animal models, this may provide a strong statement about the necessity of physical activity in order to prevent the OSP symptoms—or to contribute the understanding of their peculiar genetics. Thus, to find out whether there is a genetic predisposition for “preserved” MSK health, the GWAS of retired athletes is required. Together with multiomics, the study of human disease’s genetics may pave the way for precision medicine in the prevention or the treatment of osteoporosis and sarcopenia. Beyond knowledge of etiology, it is destined to provide new drug target(s) for pharmacy and improve the strategies of nonpharmacological intervention.
References
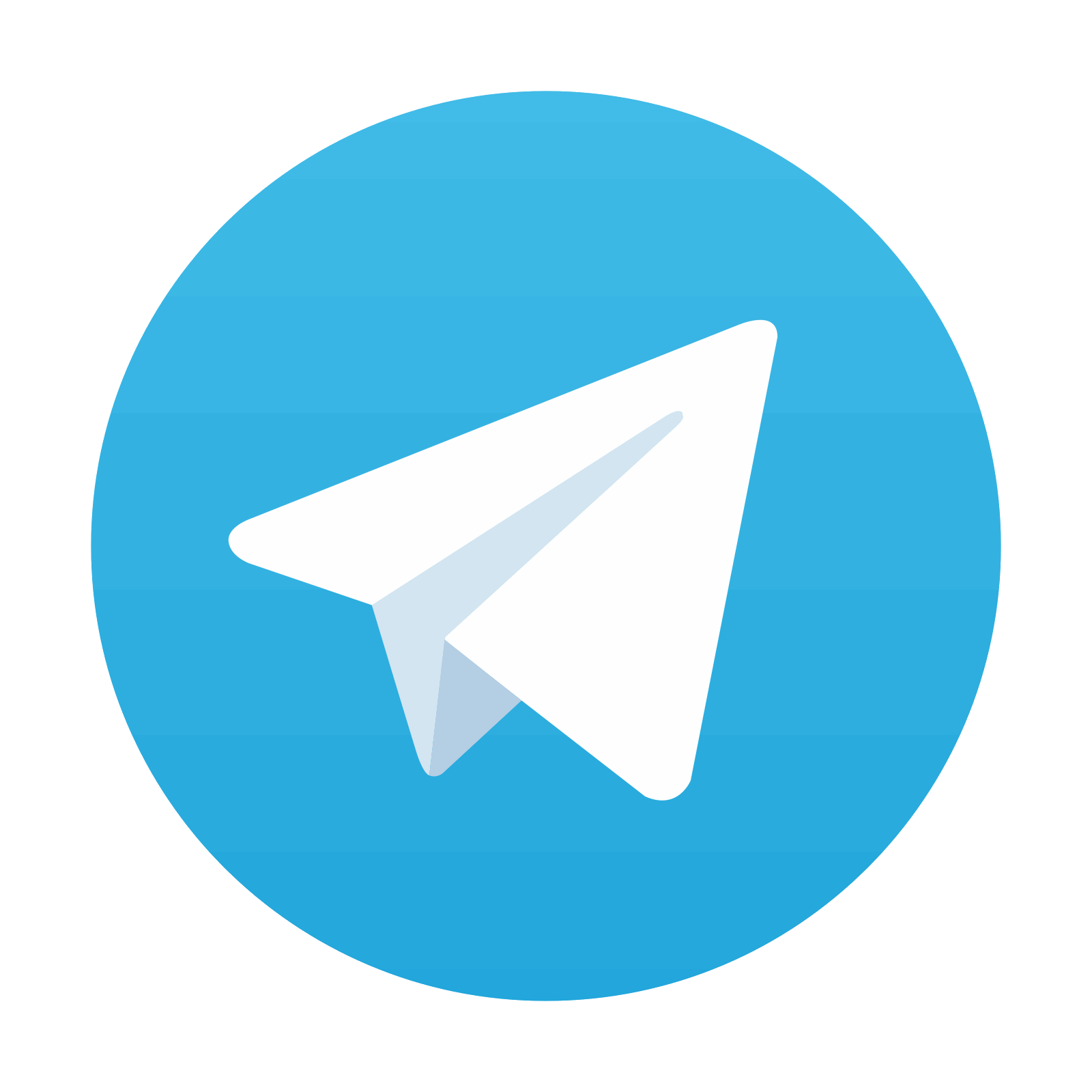
Stay updated, free articles. Join our Telegram channel

Full access? Get Clinical Tree
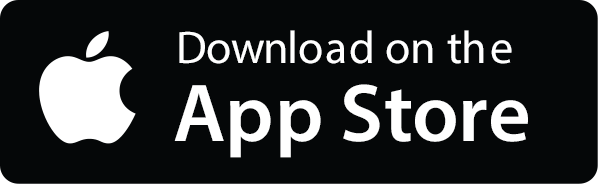
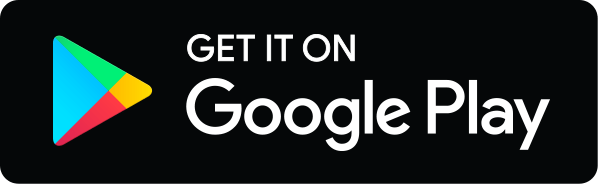
