(SNPs), variants that occur on average every 300 base pairs (bp) throughout the genome, in DNA samples from multiple individuals from several different ethnic backgrounds. Adjacent SNPs are inherited together as blocks, referred to as haplotypes, hence the name HapMap. These blocks can be identified by selected marker SNPs, so-called tag SNPs. The availability of this information permits to characterize a limited number of SNPs in order to determine the set of haplotypes present in an individual, for example, in cases and controls. This information has a significant impact on genome-wide association studies (GWAS), which search for associations of certain SNPs and haplotypes with complex disorders.
Table 12.1. Molecular Basis of Selected Endocrine Disorders | ||||||||||||||||||||||||||||||||||||||||||||||||||||||||||||||||||||||||||||||||||||||||||||||||||||||||||||||||||||||||||||||||||||||||||||||||||||||||||||||||||||||||||||||||||||||||||||||||||||||||||||||||||||||||||||||||||||||||||||||||||||||||||||
---|---|---|---|---|---|---|---|---|---|---|---|---|---|---|---|---|---|---|---|---|---|---|---|---|---|---|---|---|---|---|---|---|---|---|---|---|---|---|---|---|---|---|---|---|---|---|---|---|---|---|---|---|---|---|---|---|---|---|---|---|---|---|---|---|---|---|---|---|---|---|---|---|---|---|---|---|---|---|---|---|---|---|---|---|---|---|---|---|---|---|---|---|---|---|---|---|---|---|---|---|---|---|---|---|---|---|---|---|---|---|---|---|---|---|---|---|---|---|---|---|---|---|---|---|---|---|---|---|---|---|---|---|---|---|---|---|---|---|---|---|---|---|---|---|---|---|---|---|---|---|---|---|---|---|---|---|---|---|---|---|---|---|---|---|---|---|---|---|---|---|---|---|---|---|---|---|---|---|---|---|---|---|---|---|---|---|---|---|---|---|---|---|---|---|---|---|---|---|---|---|---|---|---|---|---|---|---|---|---|---|---|---|---|---|---|---|---|---|---|---|---|---|---|---|---|---|---|---|---|---|---|---|---|---|---|---|---|---|---|---|---|---|---|---|---|---|---|---|---|---|---|---|
|
associated with neoplasia. Epigenetic alterations, for example, altered DNA methylation, are also frequently found in malignancies and can result in altered gene expression. With the exception of triplet nucleotide repeats [8], which can progressively expand, mutations are usually stable.
relatively rare in the population. In contrast, our knowledge about the genetic basis of diabetes mellitus type 2, although rapidly growing, remains more modest [51]. This may be explained by the difficulty in detecting alleles that are only contributing mildly to the phenotype, and the fact that these alleles are frequent in the general population [4]. Moreover, one should recognize that the clinical diagnosis of diabetes mellitus type 2 may encompass various entities of impaired insulin action and secretion and that the elucidation of the genetic components will require more homogeneous collections of patients.
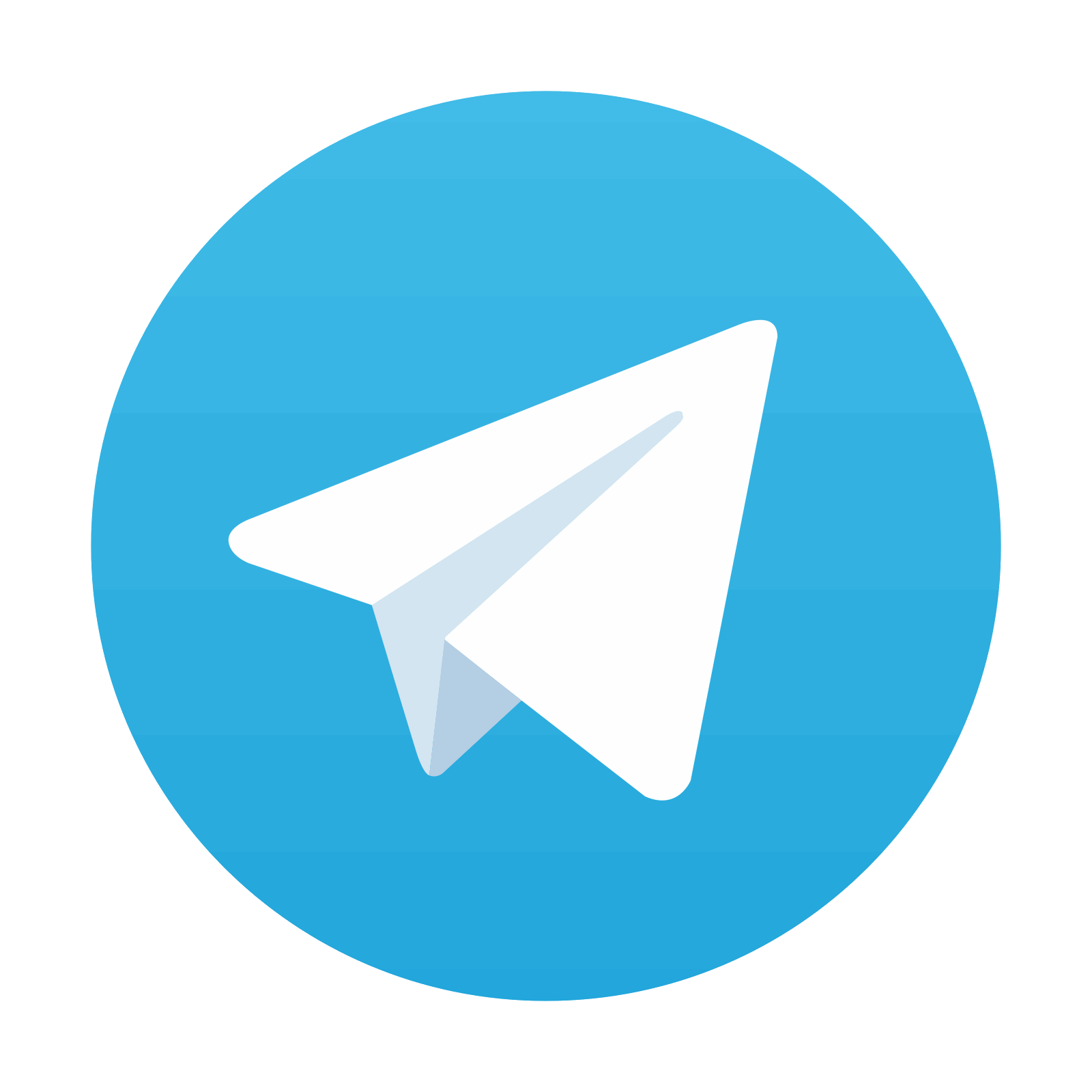
Stay updated, free articles. Join our Telegram channel

Full access? Get Clinical Tree
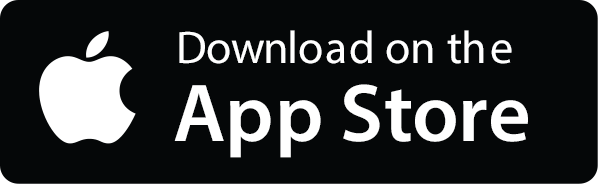
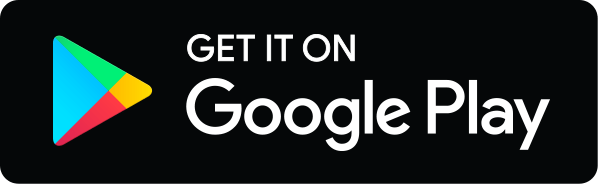