Keywords
Cancer cells, Immune system, Dendritic cells, Genetically modified dendritic cell vaccination, Tumor antigens, Melanoma
Introduction
The immune system has the potential to control or eliminate cancer cells. This is dramatically illustrated in the development of paraneoplastic syndromes in cancer patients, whereby antigen-specific T cell responses result in tumor control but destroy normal tissues that also express the antigen. Similarly, dramatic responses to anticancer immunotherapy (and, in some cases, dramatic autoimmune phenomena) have been observed recently with agents targeting immunoregulatory molecules such as PD-1 and CTLA-4 . Mouse models have demonstrated that the generation of antitumor immunity depends on presentation of tumor-associated antigens (TAAs) by dendritic cells (DCs) . Induction of effective immune responses results from complex coordination between the innate (antigen-nonspecific) and adaptive (antigen-specific) arms of the immune system. First described by Steinman and Cohn in 1973 , DCs serve as a vital link between the innate and adaptive immune systems.
Dendritic Cells and Antigen Presentation
DCs comprise a small population (<0.2% of white blood cells) of specialized antigen-presenting cells (APCs) with the unique ability to activate a broad range of immune effector cells, including T cells, B cells, and natural killer (NK) cells Because DCs are the most potent APCs, they are ideally suited to cancer vaccine delivery. DCs are derived from the bone marrow and distributed throughout the body, where they capture antigens in peripheral tissues and lymphoid tissue . They process and present the captured antigen on their surface in the form of peptide–major histocompatibility complex (MHC) complexes. Antigen-loaded DCs from peripheral tissues then migrate into the draining lymph nodes through afferent lymphatics. Antigens may migrate directly through lymphatics, where they are taken up by DCs residing in lymph nodes. Different T cell responses are generated depending on whether antigen is captured by DCs in peripheral tissues or in lymph nodes .
Activated (mature), antigen-loaded DCs stimulate the differentiation of antigen-specific T cells into effector T cells. Maturation is associated with decreased antigen capture capacity, increased expression of surface MHC II and costimulatory molecules, cytokine secretion, and acquisition of CCR7, which allows DCs to migrate . In addition, there is evidence that mature DCs are at least in part resistant to tumor-related suppression of the immune response . An essential step in the differentiation of immature DCs into mature DCs capable of initiating T cell responses is the ligation of the costimulatory receptor CD40 . Lymph node resident DCs acquiring antigen directly from the lymphatic system are the first to present peptides to naive CD4 + cells. This results in T cell priming and interleukin-2 (IL-2) production, which drives T cell proliferation and clonal expansion. Subsequently, DCs from peripheral tissues arrive in the lymph node and present peptides to the primed CD4 + T cells, leading to the generation of effector T cells. Upon interaction with an antigen-loaded DC, naive CD4 + T cells can differentiate into T helper 1 (TH1) cells, TH2 cells, TH17 cells, or T follicular helper cells that facilitate the differentiation of B cells. They may also differentiate into regulatory T (Treg) cells, which downregulate the immune response. Naive CD8 + T cells can become effector cytotoxic T cells (CTLs). DCs can also interact with NK cells, phagocytes, and mast cells, and they have an important role in regulating humoral immunity .
Generating Dendritic Cell Vaccines
The aim of DC vaccination is to induce tumor-specific effector T cells that can destroy existing tumor cells and induce immunological memory to control tumor relapse. In mouse models, DCs capture tumor antigens and cross-present those antigens to T cells in tumor-draining lymph nodes, ultimately generating tumor-specific CTLs and immune rejection of tumors . DCs are found in tumors in both humans and mice, where they capture antigen from dying tumor cells. DCs can also acquire antigens from live tumor cells for cross-presentation through a distinct process termed “nibbling” . Dying tumor cells interact with tumor cells through molecules that encode distinct “find me,” “eat me,” and “do not eat me” signals . Tumor cells may interfere with DC-induced immune responses through a variety of mechanisms.
Therapeutic DC vaccines have been tested in cancer patients for more than a decade. With the approval of sipuleucel-T by the U.S. Food and Drug Administration in 2010, immunotherapy using autologous products has entered a new era . However, the approach used to generate sipuleucel-T, based on a low frequency of DCs within the vaccine and modest therapeutic efficacy, leaves room for improvement in the burgeoning field of autologous immunotherapy. A variety of different DC vaccine strategies have been developed and tested in clinical trials, and many more are underway.
Production of Mature Dendritic Cells
The first step in ex vivo vaccine production is the generation of an enriched population of mature dendritic cells for vaccines. The discovery that DCs can be generated from monocytes or CD34 + precursors permitted harvesting of these rare cells in large numbers for the first time . When cultured in the presence of granulocyte–macrophage colony-stimulating factor (GM-CSF) plus IL-4, monocytes develop into immature DCs in 3–5 days . Cells can then be exposed to a variety of different stimuli to undergo further maturation in an additional 1- or 2-day culture period. In addition to monocyte-derived DCs, CD34 + cells can be used to generate DCs by culture in cytokine mixtures . Mature DCs are more effective than immature DCs in stimulating T cells and enhancing migration to draining lymph nodes .
Selection and Delivery of Tumor Antigens
Effective immunotherapy relies on the fact that tumors express antigens that can be recognized and targeted by the immune system . Examples of TAAs include products of tumor mutations, antigens derived from oncogenic viruses, differentiation antigens, or overexpressed self-antigens. A variety of specific examples of TAAs commonly selected for DC vaccine protocols are listed in Table 19.1 .
Disease | Reference | N | DC Modification | Immunological Endpoint | Clinical Endpoint ( n ) | Remarks |
---|---|---|---|---|---|---|
Melanoma | Tsao et al . | 12 | DCs transduced with adenovirus encoding gp100 and MART-1 | Melanoma-associated hypopigmentation in three patients | No responses | |
Melanoma | Di Nicola et al . | 6 | DCs transduced with vaccinia encoding human tyrosinase | Melanoma-associated hypopigmentation in two patients; increased frequency and maturation of tyrosinase-specific T cells | PR (1) SD (5) | |
Melanoma | Kyte et al . | 22 | Autologous whole tumor RNA transfection | Increased vaccine-specific T cell responses | SD (2) | Prolonged survival noted in 2 patients |
Melanoma | Butterfield et al . | 18 | DCs transduced with adenovirus encoding MART-1 | Increased antigen-specific T cell responses | SD (4) | |
Melanoma | Schuurhuis et al . | 11 | Electroporation with mRNA encoding gp100 or tyrosinase | DC migration to lymph nodes and CD8 vaccine responses | N/A | Stage III patients only |
Melanoma | Steele et al . | 27 | Plasmid DNA encoding melan A and gp100 | Effector responses to both antigens | PR (3) SD (4) | Novel nonviral transfection method |
Melanoma | Aarntzen et al . | 45 | Electroporation with mRNA encoding gp100 and tyrosinase | Increased antigen-specific T cell responses; improved survival in patients with TAA-specific T cell response |
| TAA-specific CD8 + T cell responses significantly higher in stage III versus stage IV patients |
Renal (clear cell) | Su et al . | 10 | Whole tumor RNA transfection | Expansion of tumor-specific T cells | Lower than expected tumor-related mortality | 80% of patients underwent secondary therapies after vaccine, not evaluable for response |
Renal | Dannull et al . | 10 | Whole tumor RNA (allogeneic) transfection | Increased tumor-specific CD4 + and CD8 + T cells | N/A | Patients also underwent depletion of Treg with denileukin diftitox (ONTAK) |
Prostate | Heiser et al . | 13 | Transfection with mRNA encoding PSA | Increased PSA-specific CD4 + and CD8 + T cells | Decreased PSA log slope (6/7) | |
Prostate | Su et al . | 20 | Transfection with mRNA encoding hTERT or hTERT-LAMP | Increased hTERT-specific CD8 + | Decreased circulating tumor cells (9/10) | Increased hTERT-specific CD4 + and CTL killing in hTERT-LAMP group only |
Prostate | Mu et al . | 19 | Transfection with allogeneic mRNA from prostate cancer cell lines | Specific immune response to vaccine | SD (11/19) Decreased PSA log slope (13/19) | Immune response correlated with clinical endpoints |
Pancreas and breast cancer | Pecher et al . | 10 | Liposomal transfection with cDNA encoding MUC1 | Increased MUC1-specific CD8 + T cells | SD (1/7 breast) | |
CEA-expressing malignancies | Morse et al . | 34 | Transfection with mRNA encoding CEA | Increased infiltrating CD4 + T cells at injection sites |
| Only patients who were NED included in phase II |
CEA-expressing colorectal and non-small cell lung cancer | Morse et al . | 14 | Infection with recombinant fowlpox encoding CEA+TriCom | Increased T cell responses against CEA | SD (5) MR (1) | |
CEA-expressing colorectal and breast cancer | Morse et al . | 15 | Infection with recombinant fowlpox encoding CEA+TriCom | Increased T cell responses against CEA Decreased circulating Treg | CR (1) SD (3) | Patients also underwent depletion of Treg with denileukin diftitox (ONTAK) |
Small cell lung cancer | Antonia et al . | 29 | Transduction with p53 via adenoviral vector | p53-specific CD8 + T cells | PR (1) SD (7) | Higher than expected response rate to post-vaccine chemotherapy |
Nasopharyngeal carcinoma | Chia et al . | 16 | Transduction with ΔLMP1-LMP2 via adenoviral vector | No increase in frequency of LMP1- and -2-specific T cells | PR (1) SD (2) |
To generate effective immune responses, the MHC molecules of a DC must be loaded with antigen. The most common strategy for achieving this is through pulsing of DCs with peptides, proteins, or irradiated tumor cells. Preparation is straightforward and involves incubation of DCs with the selected peptides, proteins, or tumor cells. Peptides are loaded directly onto MHC class I and II molecules on the surface of DCs, whereas proteins and tumor cells must undergo initial processing and presentation by DCs before stimulating CD4 + and CD8 + T cells .
Genetic modification of DCs offers several potential advantages over peptide or tumor lysate pulsing. The duration of antigen presentation should be longer in DCs expressing TAA via a transgene than peptide or lysate-pulsed DCs, where the duration of expression is determined by the half-life of the peptide–MHC complex created. In the case of viral vectors for gene transfer, the vector offers the unique advantage of combining antigen delivery with the ability to trigger DC maturation and provide a danger signal, potentially resulting in more potent antitumor immunity. A variety of gene transfer vehicles have been used to modify DCs, including viral and nonviral methods (gene gun, lipoplexes, and electroporation). Based on their high gene transfer and expression efficiency and favorable safety profile, adenoviral vectors, recombinant poxviruses, and messenger RNA (mRNA) transfection are increasingly used for genetic modification of DCs .
Of the nonviral methods, transfection of DCs with mRNA encoding TAAs by electroporation represents a viable and commonly used strategy for antigen loading without the use of bacterial or viral vectors. Similar to using proteins, the use of mRNA allows presentation of multiple epitopes and does not require determination of the patient’s haplotype or the epitopes that bind the specific haplotypes . Transfection with mRNA, in general, allows for simpler administration protocols and fewer safety concerns than viral transduction and has become a widely used method of gene transfer in dendritic cell vaccine protocols .
Examples of organisms currently under investigation in the setting of DC immunotherapy include recombinant poxviruses, Newcastle disease, Vaccinia, Sindbis, yellow fever virus, human papilloma virus, adenovirus (Ad), adeno-associated virus, and lentiviruses . A number of modifications to the viral genome must be performed prior to clinical use in the manufacture of dendritic cell vaccines for cancer therapy. In order to render them safe for clinical applications, genes required for viral replication are removed and structural/packaging sequences are separated from the viral backbone into helper plasmids . These modifications decrease the chance of homologous recombination introducing wild-type virus into the host. Removal of viral sequences also allows for the introduction of the transgene viral cassette.
In 2003, Frolkis and colleagues demonstrated that Ad could be used to generate human telomerase reverse transcriptase (hTERT)-expressing DCs from healthy donors . Since that time, Ads have become widely used viral vectors in clinical trials. Adenoviral transduction of DC results in upregulation of cell membrane MHC class II and costimulatory molecules on DC and an increased production of proinflammatory cytokines such as IL-12. Adenoviruses also provide an excellent tool for gene delivery into DCs, resulting in sustained high levels of transgenic protein production. The major disadvantage of Ad-mediated transduction of DCs is the potential immunogenicity of residual viral gene products, including preexisting immunity, which can lead to clearance of the vector by the immune system.
Clinical Trials of Genetically Modified DC Vaccines
Overall, the clinical success of DC vaccines in solid tumors has been modest. Numerous clinical trials have demonstrated the feasibility and safety of DC vaccines and have shown induction of tumor-specific T cell responses in patients. Although the majority of DC vaccine trials conducted have used nongenetic methods of loading DCs, this review focuses on genetically modified dendritic cell vaccines, primarily those produced through RNA transfection or viral transduction ( Table 19.1 ). The majority of trials completed have been phase I or II trials, and a wide variety of tumor types have been targeted. Gene-modified DC vaccines have demonstrated a favorable safety profile across multiple trials, with toxicity generally limited to local inflammatory reactions at the injection and draining lymph nodes. Systemic, flu-like symptoms have been reported less commonly. Autoimmune phenomena have been uncommon and have generally been limited to transient occurrence of autoantibodies and vitiligo-like skin changes .
Immunological endpoints have been assessed in the majority of gene-modified DC vaccine trials. Migration of gene-modified DCs to regional lymph nodes, which is an essential step in T cell activation and induction of tumor-specific immunity, has been studied in a small number of patients. The majority of clinical trials have demonstrated evidence of tumor-specific CD8 + and/or CD4 + T cell responses. Methods used to assess T cell responses included delayed-type hypersensitivity reactions, interferon-γ (IFN-γ) enzyme-linked immunospot assay, tetramer staining, and assessment of CTL killing activity.
Melanoma
The immune responsiveness of melanoma has been observed clinically, making this tumor type a natural target for immunotherapies, including dendritic cell vaccination. In 2002, Tsao et al . reported results of a phase I trial of adenovirus-mediated transduction of the melanocyte-specific antigens gp100 and MART-1 into DCs in 12 patients with metastatic melanoma . The investigators reported the observation of vitiligo-like skin changes in 3 patients. However, these skin changes were not associated with clinical responses. Di Nicola et al . conducted a phase I trial in 6 patients with advanced melanoma, administering vaccine consisting of dendritic cells transduced ex vivo with a recombinant vaccinia vector encoding the human tyrosinase gene . Only patients whose tumors expressed the tyrosinase antigen were included in the study. Two patients developed vitiligo-like skin changes during vaccination. One partial response was observed, and 4 patients experienced stable disease following vaccination. The investigators observed immunological responses in the majority of patients, with increased frequency and maturation of T cells directed to tyrosinase. Butterfield et al . performed a phase I/II trial in patients with metastatic melanoma, using autologous DCs transduced with replication-defective Ad encoding the melanoma antigen MART-1 . MART-specific CD4 + and CD8 + T cell responses were observed and 4 of 17 patients experienced stable disease, but there were no clinical responses.
Nonviral methods of creating DC vaccines have been employed in a number of clinical trials in melanoma patients. Kyte et al . conducted a phase I/II trial in 22 patients with advanced melanoma . Their vaccine was produced by transfecting DCs with autologous whole tumor mRNA via electroporation. Vaccine-specific immune responses were observed in 9 of 19 evaluable patients. Two patients had stable disease. Delayed-type hypersensitivity reactions were observed at the injection site in 8 patients, and 1 patient developed vitiligo-like skin changes.
In addition to evaluating immunologic responses, Schuurhuis et al . investigated the in vivo migratory behavior of mRNA-electroporated DCs in a phase I/II trial in patients with stage III melanoma . Eleven patients with stage III melanoma expressing the TAAs gp100 and tyrosinase who were scheduled to undergo lymph node dissection were included. DCs were electroporated with synthetic mRNA for either gp100 or tyrosinase and labeled with indium-111 and superparamagnetic iron oxide prior to injection. Subjects received injections into a single lymph node of the region scheduled for dissection 1 or 2 days prior to surgery and three additional intranodal injections after surgery. Scintigraphic imaging was then used to study migration of mRNA-electroporated DCs and revealed that electroporation did not affect the ability of DCs to migrate to multiple lymph nodes after injection. The investigators also demonstrated expression of TAA protein inside the lymph node and activation of CD8 + T cell responses in the majority of patients.
Aarntzen et al . recently conducted a trial that included two cohorts of patients, 26 with stage III melanoma and 19 with stage IV disease . Monocyte-derived DCs were electroporated with mRNA encoding the TAAs gp100 and tyrosinase, and the resulting vaccine was administered intranodally. TAA-specific T cell responses were assessed in blood and skin-test infiltrating lymphocyte (SKIL) cultures. TAA-specific CD8 + T cells recognizing multiple epitopes and producing elevated levels of IFN-γ were observed significantly more often in patients with stage III versus stage IV melanoma, supporting the authors’ hypothesis that immune responses would be more robust in patients with a lower tumor burden. Among stage IV patients, 5 patients experienced stable disease and 1 patient had a partial response initially and subsequently complete regression of disease. The authors observed a trend toward improved progression-free and overall survival among stage IV patients who demonstrated TAA-specific T cell responses in blood or SKIL cultures.
Another recent melanoma trial investigated a novel nonviral transfection method, whereby dendritic cells were loaded with the TAAs melan A and gp100 using plasmid DNA condensed with a cationic peptide . This phase I/II study enrolled 27 patients with metastatic melanoma. Partial responses were observed for 3 patients, and an additional 4 patients experienced stable disease. The majority of patients demonstrated an expansion of effector responses to both melan A and gp100, to the HLA A2-resricted modified epitope melan A ELAGIGILTV, and to a panel of MHC class I- and II-restricted epitopes.
Genitourinary
A number of trials of genetically modified DCs have been conducted in patients with prostate cancer. Heiser et al . conducted a phase I clinical trial in patients with advanced prostate cancer and serum prostate-specific antigen (PSA) values greater than 4 ng/dl . Patients received escalating doses of autologous DCs transfected with PSA mRNA. Induction of PSA-specific T cell responses was consistently detected across all patients. Of the 7 patients evaluable for serum PSA response, only 1 demonstrated a decrease in serum PSA, although an additional 5 patients manifested a decrease in the post-treatment PSA log slope (velocity). Mu et al . administered DCs transfected with allogeneic mRNA from prostate cancer cell lines to 19 patients with metastatic, androgen-resistant prostate cancer and observed cellular immune responses specific for antigens encoded by the mRNA derived from prostate cancer cell lines in 12 of 19 patients . In addition, 13 of 19 patients experienced a decrease in PSA velocity. Clinical outcome was strongly correlated with immune responses.
Su et al . conducted a clinical trial in 20 patients with metastatic prostate cancer . Patients were randomized to receive either DC transfected with hTERT or a chimeric lysosome-associated membrane protein-1(LAMP) and hTERT mRNA. The addition of LAMP in the second cohort was based on earlier work demonstrating that LAMP can direct hTERT antigen processing into the class II pathway and enhance CD4 + T cell responses . They observed expansion of hTERT-specific CD8 + T cells in 19 of 20 subjects. However, patients in the LAMP–hTERT cohort were significantly more likely to develop hTERT-specific CD4 + T cells than those who received hTERT mRNA-modified DCs alone. The authors also observed enhanced CTL-mediated killing of hTERT targets in the LAMP–hTERT group. Circulating tumor cells (CTCs) were evaluable in 10 subjects at baseline, and transient reduction or clearance of CTCs was observed in 9 of these patients. Vaccination was also associated with a reduction in PSA velocity in some patients.
In a phase I trial, Su et al . administered autologous dendritic cells transfected with total renal tumor RNA to 10 patients with advanced renal cell carcinoma who had undergone nephrectomy . Patients received three escalating doses of renal tumor RNA-transfected DCs with no evidence of dose-limiting toxicity or vaccine-related adverse effects. In 6 of 7 evaluable patients, expansion of tumor-specific T cells was detected after vaccination. T cell reactivity was observed against an array of renal TAAs, including TERT, G250, and oncofetal antigen, but not against self-antigens expressed in normal tissues. The majority of patients underwent additional therapy within a short time period after vaccination; therefore, the investigators were unable to reliably determine clinical responses to vaccine. Subsequent therapy included IL-2 in the majority of patients. Although clinical responses were not reported, the investigators did report an unexpectedly low mortality rate for this patient population, with 70% of patients alive at a mean follow-up of 19.8 months.
Dannull et al. also used allogeneic whole tumor RNA-transfected DCs in a clinical trial in 10 patients with metastatic renal cell carcinoma and 1 patient with disseminated ovarian carcinoma . Seven subjects received denileukin diftitox for depletion of Tregs prior to vaccination, whereas the remaining 4 patients received vaccine alone. Receipt of denileukin diftitox was associated with a reduction in peripheral blood Tregs 4 days after the infusion. Significantly higher frequencies of tumor-specific CD8 + T cells were observed in patients treated with the combination of DC vaccine and denileukin diftitox compared to vaccine alone. Clinical outcomes were not reported.
Lung
We have reported results of our study of a vaccine consisting of DCs transduced with the p53 gene delivered via an adenoviral vector in patients with extensive stage small cell lung cancer (SCLC). In our pilot study, we enrolled 29 patients with extensive stage SCLC who had failed conventional chemotherapy with platinum and etoposide or platinum and irinotecan. DCs were generated from peripheral blood mononuclear cells obtained by leukapheresis and then infected with an adenoviral construct containing wild-type p53. Vaccines were administered via intradermal injection at four separate sites draining to the bilateral axillary and inguinal lymph nodes. This was repeated on three separate occasions at 2-week intervals. If patients showed stable disease or better, they were given three more doses of the vaccine, once per month. T cell immune responses to p53 were evaluated, and statistically significant p53-specific T cell responses were noted in 57% of patients. One patient had a partial response to therapy and 7 patients exhibited stable disease, whereas the remainder had progressive disease. Twenty-three patients went on to receive additional chemotherapy after progression; thirteen of these patients were platinum-resistant (progressed within 90 days of receiving a platinum-containing regimen). Whereas previously published objective response rates to chemotherapy in platinum-resistant SCLC are 2–5%, we observed a response rate to subsequent salvage chemotherapy of 61.5% in our platinum-resistant patients who had received the DC vaccine. A randomized phase II study is underway to confirm prospectively the finding of increased responsiveness to salvage chemotherapy in patients receiving the vaccine.
Gastrointestinal
Morse et al . performed a phase I–II clinical trial of dendritic cells transfected with mRNA encoding carcinoembryonic antigen (CEA) in patients with CEA-expressing malignancies . The phase I dose escalation included 29 patients with advanced disease (9 lung, 9 breast, and 11 colorectal cancers). Based on the observation of better immune responses in patients with a lower tumor burden in the phase I portion, the phase II portion of the study included 13 patients with no evidence of disease after a curative resection of a focus of CEA-expressing malignancy (1 breast, 1 unknown primary, and 11 colorectal cancers). In the phase I portion, one complete response and two minor responses were observed. Three patients experienced prolonged stable disease, with 1 patient having stable disease for more than 2 years. In the phase II portion of the study, 3 patients remained without evidence of disease for more than 500 days of follow-up. For those patients who experienced disease recurrence, the median time to recurrence was 122 days.
Morse et al . conducted a phase I study of autologous dendritic cells infected with recombinant fowlpox encoding CEA and a triad of costimulatory molecules (B7-1, LFA-3, and ICAM-1) . A total of 14 patients with advanced CEA-expressing malignancies were included in the trial—11 with colorectal cancer and 3 with non-small cell lung cancer. All patients had advanced disease and had failed at least two prior regimens. Patients in the first cohort received one cycle of vaccine, consisting of administration of one dose of fresh dendritic cell vaccine administered intradermally and subcutaneously followed by three weekly doses of previously cryopreserved vaccine. In the second cohort, patients received two cycles of dendritic cell vaccines. No dose-limiting toxicities or adverse events related to vaccine were observed. Five patients experienced stable disease, and 1 patient experienced a minor response and a decrease in serum CEA level. Increased frequency of CEA-specific T cells was observed in 10 patients, with a trend for greater peak frequency of CEA-specific T cells among those with a minor response or stable disease.
The same group later conducted a phase I trial utilizing the same recombinant fowlpox CEA-encoding vaccine and costimulatory molecules but with the addition of denileukin diftitox (ONTAK; Eisai, Woodcliff Lake, NJ) prior to dendritic cell vaccine administration . Denileukin diftitox, a fusion between the active domain of diphtheria toxin and IL-2, has been shown to deplete Treg cells , which limit antigen-specific immune responses and facilitate resistance to anticancer immunotherapy . Fifteen patients with advanced CEA-expressing malignancies (14 with colorectal cancer and 1 with breast cancer) were included in the trial. The investigators demonstrated that circulating Treg cells were reduced in 4 of 4 patients who received doses of denileukin diftitox prior to each vaccine but not in those who received only a single dose of denileukin diftitox prior to the first vaccine or in a control group who received only vaccine. By imaging, 1 patient experienced a minor response and 2 patients had stable disease. However, a patient deemed as having progressive disease later underwent surgical resection of an enlarged retroperitoneal lymph node with only necrotic tissue found. An additional patient experienced progression on initial assessment but went on to have stable disease for 6 months. Thirteen of 14 evaluable patients demonstrated a CEA-specific T cell response.
Nasopharyngeal Carcinoma
Chia et al . administered DCs transduced with the nasopharyngeal carcinoma-associated Epstein–Barr virus (EBV) antigens ΔLMP1–LMP2 via a recombinant adenoviral vector to 16 patients with metastatic nasopharyngeal carcinoma . Delayed-type hypersensitivity reactions were observed in 9 of 12 evaluable patients. No increase in the frequency of EBV LMP1/2-specific T cells was observed. One patient achieved a partial response, and 2 patients had stable disease lasting more than 6 months. However, the median progression-free survival was only 1.9 months.
Brain
Whereas studies utilizing peptide-pulsed DCs have been conducted in brain tumor patients, genetically modified DC trials are lacking in adult brain tumor patients. Caruso et al . conducted a phase I study in nine pediatric patients with recurrent brain tumors using DCs transfected with whole tumor mRNA . Seven patients completed the vaccine protocol: One partial response and two patients with stable disease were seen. None of the evaluable patients exhibited cellular immune responses to the vaccine, although it was noted that cellular immune responses at baseline were generally compromised in this patient cohort.
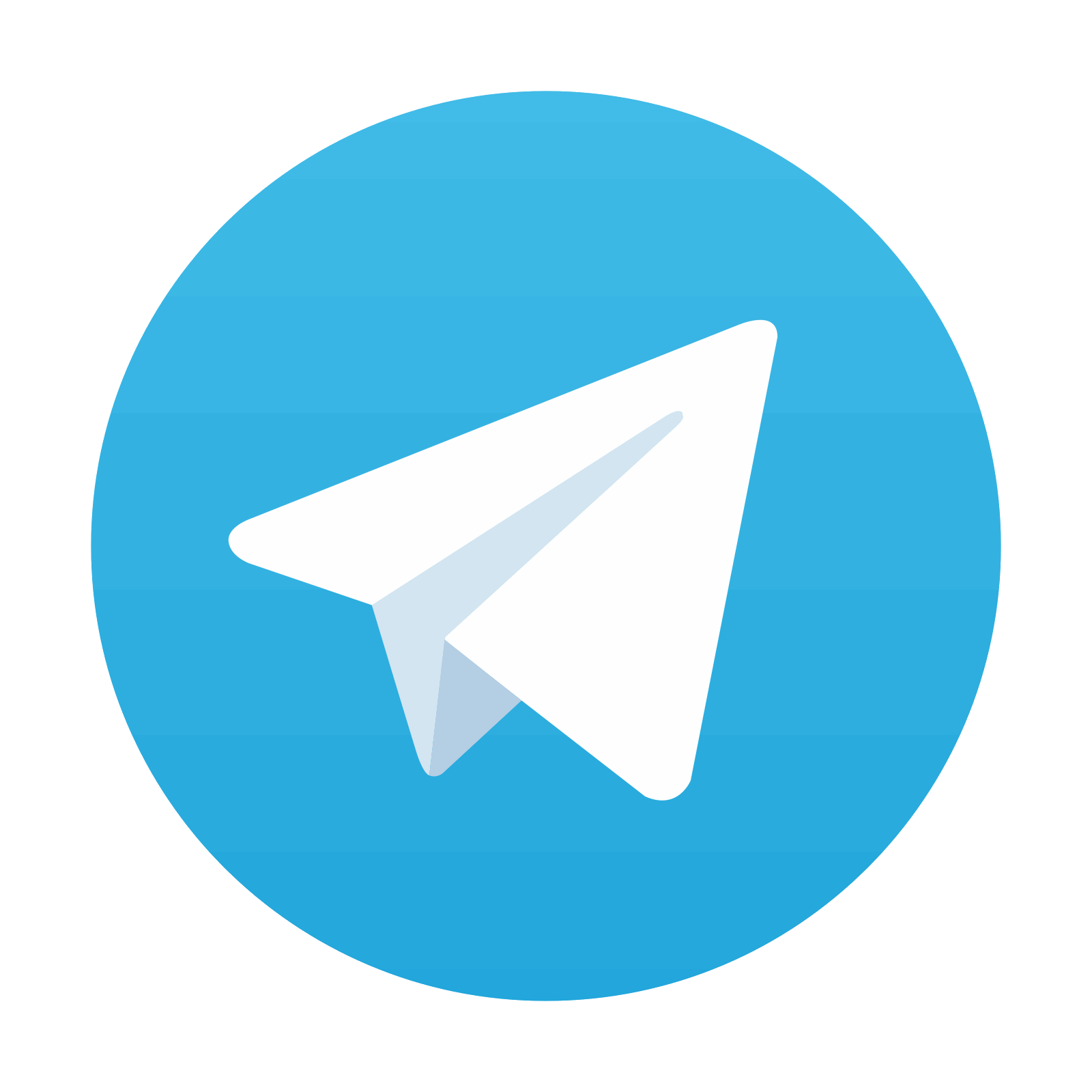
Stay updated, free articles. Join our Telegram channel

Full access? Get Clinical Tree
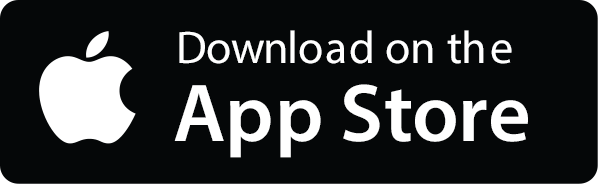
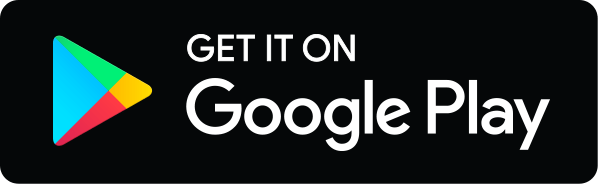