Keywords
Salmonella , genetic engineering, gene delivery, gene therapy, attenuation, toxicity, imaging
Introduction
Gene therapy is considered to be one of the most promising therapeutic approaches for treating cancer, but the optimized delivery of genes into target cells is a significant issue that hinders successful gene therapy . Several different types of delivery vectors (viral, nonviral, and bacterial vectors) have been investigated in an attempt to resolve this issue .
Many therapeutic approaches to cancer have used bacteria because they have distinctive characteristics as anticancer agents and as delivery vectors during gene therapy . Certain strains of bacteria, such as Escherichia coli , Salmonella , Clostridium , Bifidobacterium , and Listeria , can selectively colonize and grow in tumors. A number of recent reports have demonstrated that bacteria are capable of targeting primary tumors and metastases , and this feature is being exploited by tumor-selective drug delivery .
Salmonella typhimurium has several possible advantages compared to the other strains: It can grow in aerobic or anaerobic conditions that are present in solid tumors; it expresses specialized systems that mediate the invasion of epithelial cells and macrophages; and there is a vast body of knowledge and a comprehensive understanding of its genetics, which facilitates the genetic engineering of this bacterium for cancer therapies . The genetic engineering of Salmonella has become very important for lowering its toxicity and maximizing its therapeutic efficacy, particularly since the 2000s .
However, there are still many hurdles to overcome before bacterial-mediated cancer therapy is translated from preclinical studies to clinical practice. This chapter introduces the genetic engineering of Salmonella according to the specific requirements of effective cancer therapy, and it summarizes possible directions for future clinical applications.
Genetic Engineering Map for Cancer Therapy
Salmonella species have been investigated in genetic studies to overcome or compensate for the problems of conventional therapies, including chemotherapy, radiation therapy, and existing gene therapy methods, particularly in four main areas ( Figure 30.1 ): (1) lowering intrinsic toxicity, (2) promoting target cell selectivity, (3) delivering therapeutic drugs (cargo drug), and (4) producing imaging signals .

Many different therapeutic approaches based on Salmonella species have been applied in animal models, and most have combined different forms of engineering techniques in these four areas. Thus, Salmonella has been transformed into a combination of genetically engineered elements (known as a genetic engineering map) for specific purposes.
Salmonella as an Effective Delivery Vector
An ideal vector has to be intrinsically safe in living subjects, including humans, and it must accumulate selectively in the target tissue or cell, which facilitates the delivery of cargo molecules to specific tissues, cells, or the cellular nucleus. Therefore, the genetic engineering of Salmonella is very important for generating nonvirulent strains, enhancing its therapeutic potency, and regulating the host immune response. The genetic material should be transcribed without degradation due to environmental factors, such as enzymes and the immune system .
Attenuated Salmonella typhimurium
The attenuation of virulence has been achieved by auxotrophic mutations that affect the biosynthesis of purines or aromatic amino acids , by mutations in the biosynthesis of lipid A , or by deletions of the relA and spoT genes, which generate strains defective in guanosine 5′-diphosphate 3′-diphosphate (ppGpp) synthesis .
Salmonella typhimurium VNP20009 was genetically engineered by the partial deletion of the msbB and purI genes . The msbB gene is responsible for the addition of a terminal myristyl group to lipid A. The lipopolysaccharide (LPS) produced by these lipid A mutants has a much lower capacity for inducing tumor necrosis factor-α (TNF-α) . The purI gene can induce a growth requirement for external sources of purines , and a purI gene mutation results in purine deficiency and a limited replication rate .
Salmonella typhimurium A1 is auxotrophic for leucine and arginine, and it was developed to prevent continuous infections in normal tissues while maintaining virulence against tumors because it has no other attenuated mutations compared with S. typhimurium VNP20009 . To enhance its tumor virulence, S. typhimurium A1 was injected into nude mice that had been transplanted with an HT-29 human colon tumor. The bacteria isolated from the infected tumor were then cultured, and the re-isolated A1 was designated A1-R. The number of A1-R bacteria attached to HT-29 human colon cancer cells was approximately six times higher than that of the parent A1 .
ppGpp is a stringent signaling molecule that is considered to be a key molecule for the cessation of ribosome production when a bacterial culture enters the stationary phase . Recently, ppGpp was implicated in the stationary phase induction of Salmonella pathogenicity island type I (SPI-1) genes by the activator hilA, which is a master transcriptional regulator of SPI-1-encoded genes. During the course of infections, it has been suggested that Salmonella encounters various stressful environments, which are sensed and translated into the intracellular signal ppGpp, and this induces the expression of Salmonella virulence genes, including SPI-1 genes . A ppGpp-defective Salmonella mutant exhibited significant virulence attenuation, whereas the LD 50 of an S. typhimurium mutant that was defective for ppGpp synthesis (STΔppGpp) was increased by five orders of magnitude, irrespective of the administration route . This strain has been investigated in mouse models .
Selective Targeting of Tumors
From the standpoint of the interaction between bacteria and tumor cells or tissues, tumors have several unique characteristics compared with normal tissue, including hypoxic or necrotic areas, immune-privileged microenvironments, and abundant nutrients.
As a facultative anaerobe, Salmonella is thought to use several mechanisms to accumulate in tumors, such as the entrapment of Salmonella in the tumor vasculature , flooding into tumors after TNF-α-mediated inflammation , chemotaxis toward compounds produced by tumors , preferential amplification in tumor-specific microenvironments , and protection from immune system clearance .
The attenuation of Salmonella by auxotrophy reduces its virulence in mice but also amplifies its targeting capacity by two or three orders of magnitude in tumors compared with the liver . Auxotrophic mutants require exogenous nutrients, so they are generally weakened in mice, which allows them to be controlled or eradicated by the host defense mechanisms. Tumors contain actively dividing cells and necrotic areas, and they appear to be an environment in which metabolites such as purines, pyrimidines, and amino acids are plentiful, thereby providing a reservoir of nutrients for bacteria.
Salmonella chemotaxis using surface chemoreceptors also supports the targeting mechanism. A study using knockout bacteria showed that the aspartate receptor initiates chemotaxis toward viable tumor tissue where the serine receptor initiated tissue penetration and the ribose/galactose receptor directed S. typhimurium toward necrotic areas .
By contrast, attenuated Salmonella with a partial deletion of the msbB gene had a lower potential for accumulating in tumor tissues due to a decline in TNF-α, which could also lead to an influx of blood into tumors . Therefore, an adequate balance between attenuation and immunogenicity must be achieved.
Effective Delivery of Genes or Gene-Related Materials
As a delivery vector, Salmonella has been applied to gene therapy via three delivery routes : (1) the direct transfer of genes (or DNA) into the target tissue , (2) the delivery of proteins that were originally expressed by bacteria , and (3) the transfer of plasmids encoding small hairpin RNAs (shRNAs) ( Figure 30.2 ) . The direct transfer of genes (also known as bactofection) is the bacteria-mediated transfer of plasmid DNA into mammalian cells. Bacteria disrupt and release a plasmid, encoding the therapeutic gene after escaping from the blood vessel and entering the target cell. The plasmid is transferred into the cell nucleus and a therapeutic protein, with cytotoxic activity or immune response, is expressed by the host cell . Bactofection of mammalian cells can be achieved via the active invasion of nonphagocytic mammalian cells or passive uptake by phagocytic immune cells . Compared with other delivery mechanisms, such as protein delivery, this approach has benefits and drawbacks. Bactofection may produce stronger, more stable expression because it uses the mammalian expression system. However, the expression of the transferred genes may be more difficult to control . A poor transfer efficiency could also limit the therapeutic response because the transferred genes may be expressed exclusively in infected cells rather than being distributed homogeneously in the tissues. Furthermore, plasmids generally cannot replicate in host mammalian cells .

Another approach is the bacterial delivery of therapeutic agents, in the form of proteins instead of genes. Bacteria can be used as protein production factories, so they can continue to produce cargo proteins while proliferating in the target tissues, either in the extracellular spaces or inside the targeted cells . The persistent bacteria can produce the requisite polypeptide in situ , thereby ensuring the localized distribution of a therapeutic agent without affecting systemic nontarget organs . This approach may facilitate the specific regulation of protein expression using inducible promoters (discussed later). Furthermore, the bacteria do not integrate the delivered genetic material into the host genome, which greatly decreases the risk of delivery-induced mutagenesis and tumorigenesis .
Finally, bacteria can be engineered to deliver plasmid-based shRNAs into tumor cells, where they produce small interfering RNA (siRNAs) (also known as gene silencing) . siRNAs induce the degradation of mRNAs transcribed by a specific gene such as Bcl2 or the signal transducer and activator of transcription 3 (STAT3) gene . The proteins expressed by both genes inhibit apoptosis, and STAT3, in particular, promotes cancer cell growth. The combination of these two advanced approaches (i.e., bacterial delivery and RNA interference) is expected to be a promising tool for cancer therapy .
Salmonella as Advanced Therapeutic Strategies
As mentioned previously, Salmonella can deliver various types of therapeutic agents into target tissues via different delivery mechanisms. In addition, clinical and preclinical studies have been conducted to validate the effectiveness of bacteria-mediated drug delivery according to their mechanisms of action in cancer. These studies have included prodrug-converting enzymes, cytotoxic agents, cytokines, and tumor-specific antigens or antibodies ( Table 30.1 ). These therapeutic strategies have been investigated using the appropriate delivery routes—that is, gene transfer or protein delivery.
Therapeutic Strategy | Engineering Approach | Related Genes or Molecules | References |
---|---|---|---|
Prodrug activation | Introduction of a gene encoding a prodrug-converting enzyme into target cells | CD, HSV-TK | |
Cytotoxic effect | Introduction of cytotoxic genes or proteins to kill cancer cells | Endostatin, thrombospondin-1, SMAC, TRAIL, cytolysin A, FAS ligand, TNF-α | |
Immune Activation | |||
Cytokines | Delivery of genes encoding cytokine(s) expressed by Salmonella to enhance the anticancer effects of immune cells | FLT3L, IL-12, GM-CSF, IL-2, IL-18, CCL21, LIGHT | |
Tumor-specific antigens (Ags) | Delivery of genes encoding tumor-specific Ags, or the Ags themselves, to sensitize immune cells via antibody formation | α-Fetoprotein, VEGFR, PSA, NY-ESO-1, CPV, RAF-1 |
Prodrug Conversion Strategies
Prodrug-converting enzymes (or suicide enzymes) aim to improve the therapeutic efficiency (benefit vs. toxic side effects) of cancer chemotherapy . A gene, encoding an enzyme that is not naturally expressed in the host, is introduced into the target cells by Salmonella and the transformed cells express the enzyme .
Salmonella typhimurium has been engineered to express suicide enzymes, such as cytosine deaminase (CD) and herpes simplex virus thymidine kinase (HSV-TK) , and administration of the appropriate prodrugs (5-fluorocytosine or ganciclovir, respectively) leads to selective localization of the active forms, leading to tumor growth suppression or regression .
Cytotoxic Approaches to Controlling Cancer Cells
This strategy involves the introduction of anti-angiogenic or cytotoxic genes and native bacterial toxic proteins, which kill cancer cells .
The direct transfer of anti-angiogenic genes such as endostatin and thrombospondin-1 can kill cancer cells by preventing new blood vessel formation and blocking the nutrient supply. Salmonella has been used as the carrier to deliver cytotoxic genes that encode the second mitochondria-derived activator of caspases (SMAC) and tumor necrosis factor-related apoptosis-inducing ligand (TRAIL), which can induce apoptosis in many types of cancer cells .
Furthermore, bacterial toxic proteins, such as cytolysin A (also known as HlyE) , and other cytotoxic proteins, such as FAS ligand , TNF-α, or TRAIL , can be delivered to cancer cells using engineered Salmonella , where they induce apoptosis. In particular, the extracellular domain of TRAIL is a potent apoptotic agent in tumor cells, whereas it has minimal toxicity against normal cells. Attenuated Salmonella has been used to express TRAIL under the control of a prokaryotic promoter, resulting in tumor growth reduction .
Activation of the Immune System Using Cytokines
Unlike normal cells, cancer cells are generally tolerant of their host’s immune system, and they prevent immune cells from recognizing them as cancer cells. Conventional therapies such as chemotherapy or radiation therapy are limited when increasing the dose to eliminate all tumor cells because they can cause severe side effects in normal tissues. Immune-based therapy (immunotherapy) using cytokines is considered a powerful therapeutic tool, but it has several problems, including a short in vivo duration and severe side effects after the systemic administration of cytokines . Therefore, immunotherapy using genetically engineered bacteria may be a promising tool for overcoming the limitations of existing therapeutic methods.
Immunotherapy using Salmonella includes the transfer of specific genes encoding cytokines and the delivery of cytokines expressed by bacteria, which can enhance the anticancer effects of various lymphocytes, such as B cells, T cells, and natural killer cells .
Several different types of cytokines and growth factors have been investigated via the direct transfer of genes. These molecules, including FLT3L , interleukin-12 (IL-12) , and granulocyte–macrophage colony-stimulating factor (GM-CSF) , can activate several types of lymphocytes.
Salmonella has also been studied with respect to the delivery of cytokines produced intrinsically by the bacteria. These include IL-2 , IL-18 , CCL21 , and LIGHT . These cytokines induce T cells, natural killer cell proliferation (IL-2 or IL-18), dendritic cell growth (LIGHT), and leukocyte and neutrophil infiltration (LIGHT or CCL21), and they control the migration of immune cells (CCL21) .
Tumor-Specific Antigens
The engineering of Salmonellae to produce a cancer vaccine is another type of immunotherapy used to break the preexisting tolerance of the immune system with regard to tumor-specific antigens. Several members of the Salmonella genus have been used in vaccination strategies in which they serve as DNA or antigen carriers .
DNA vaccine delivery by genetically engineered Salmonella can inhibit the growth of several types of tumors that express specific antigens, such as α-fetoprotein and vascular endothelial growth factor (VEGF) receptor 2 . Antibodies against these antigens can prevent tumor formation, tumor growth, and angiogenesis .
In addition to DNA delivery, vaccine therapy based on antigen delivery has also been investigated using Salmonella . Immune cells may be sensitized and the formation of tumors that express specific antigens can be prevented by the delivery of these antigens, such as prostate-specific antigen (PSA) , NY-ESO-1 , canine parvovirus (CPV) , and RAF-1 .
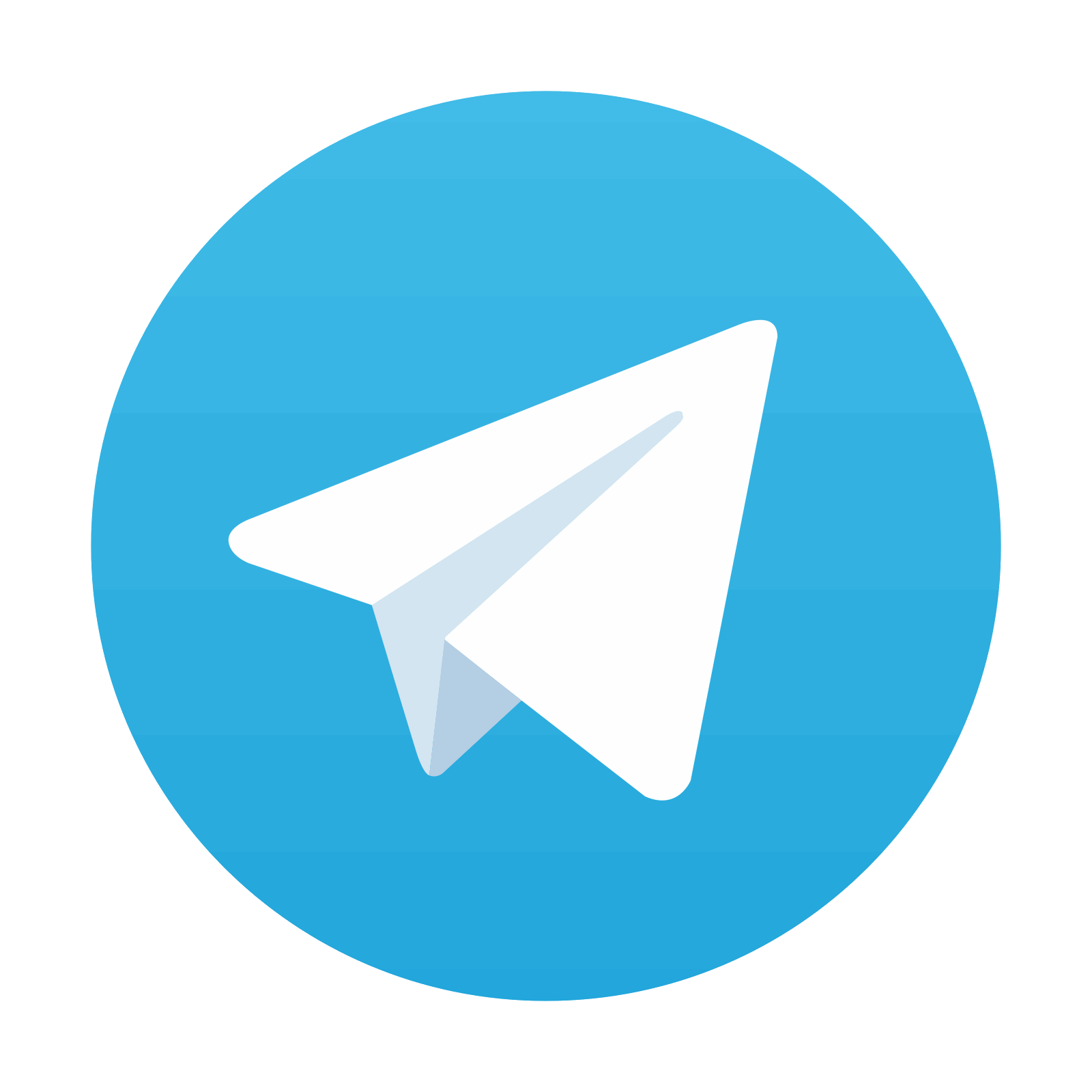
Stay updated, free articles. Join our Telegram channel

Full access? Get Clinical Tree
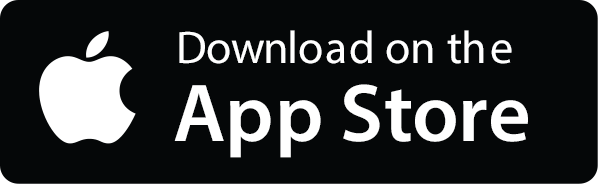
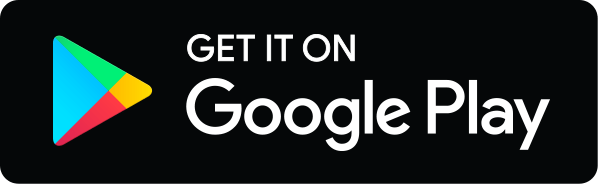