- 14.1 Introduction
- 14.1.1 Normal immune mechanisms
- 14.1.2 Spectrum of the intestinal immune response
- 14.1.1 Normal immune mechanisms
- 14.2 Infection and the gut
- 14.2.1 Infection and the gut
- 14.3 Gastritis
- 14.3.1 Atrophic gastritis and pernicious anaemia
- 14.4 Food-induced gastrointestinal disease
- 14.4.1 Food allergy
- 14.4.2 Coeliac disease
- 14.4.1 Food allergy
- 14.5 Autoimmune enteropathy
- 14.6 Inflammatory bowel disease
- 14.6.1 Crohn’s disease and ulcerative colitis
- 14.7 Viral hepatitis
- 14.7.1 Hepatitis A
- 14.7.2 Hepatitis B
- 14.7.3 Hepatitis C
- 14.7.4 Other hepatitis viruses
- 14.7.1 Hepatitis A
- 14.8 Autoimmune liver diseases
- 14.8.1 Chronic active hepatitis
- 14.8.2 Primary biliary cirrhosis
- 14.8.3 Associated syndromes
- 14.8.4 Alcohol-induced liver disease
- 14.8.5 Drug-induced liver disease
- 14.8.1 Chronic active hepatitis
Visit the companion website at www.immunologyclinic.com to download cases with additional figures on these topics.
14.1 Introduction
14.1.1 Normal immune mechanisms
The gastrointestinal tract is the largest immunological organ in the body. Over 90% of the exposure of the human body to microorganisms occurs at the mucosal surface of the gastrointestinal tract and over 400 bacterial species inhabit the average human gut. The gut is protected by several non-specific mechanisms (Fig. 14.1). Epithelial cells form an important physical barrier via their intercellular tight junctions and turn over rapidly (every 24–96 h). Any injury to the epithelial barrier results in rapid migration of adjacent viable epithelial cells to cover the denuded area, a process called ‘restitution’, while lymphocytes and macrophages migrate out through pores in the basement membrane to provide temporary host protection. The acid pH of the stomach is a formidable chemical barrier to many organisms and bacterial overgrowth is a consequent complication in patients with achlorhydria due to atrophic gastritis. Any change in the normal microflora of the intestine also allows pathogenic bacteria to flourish: an example is pseudomembranous colitis caused by the toxin-producing bacterium Clostridium difficile in patients given certain antibiotics.
Mucosal immune responses involve the gut-associated lymphoid tissue (GALT). Lymphocytes are found at three sites within the mucosa (Fig. 14.2 and Box 14.1).
- Organized lymphoid aggregates (Peyer’s patches) beneath the epithelium of the terminal small intestine
- Lymphocytes within the epithelial cell layer (IELs)
- Lymphocytes scattered, with other immunocompetent cells, within the lamina propria
GALT is divided into two functional compartments: an afferent arm – Peyer’s patches – where interaction occurs between luminal antigen and the immune system; and an effector arm – the diffusely distributed intraepithelial and lamina propria lymphocytes.
Peyer’s patches are covered by specialized epithelium (follicle-associated epithelium). Some of these epithelial cells have surfaces that seem folded under the scanning electron microscope (Fig. 14.3). These microfold, or M, cells sample and actively transport particulate antigens from the lumen into the ‘dome’ area, where priming of both T and B lymphocytes occurs. Within Peyer’s patches are specialized T cells that induce antigen-activated IgM-bearing B lymphocytes to switch isotype to IgA as well as immature IgA+ cells that are probably independent of T cells.
Fig. 14.3 The stages involved in the transport of antigen by the M cell from the intestinal lumen into the extracellular space where it is taken up by dendritic cells and T lymphocytes (L).
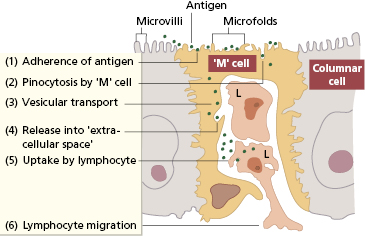
Primed B lymphoblasts, committed mainly to producing IgA class antibody, migrate from Peyer’s patches, via the lymphatics and mesenteric lymph nodes, to the thoracic duct and hence into the circulation (Fig. 14.2). These cells return preferentially to the lamina propria, a process called ‘homing’. Once back in the gut, they mature into IgA plasma cells and are responsible for local and secretory immune defences. The number of IgA-producing cells in the lamina propria far exceeds the numbers producing IgM, IgG or IgE.
The IgA coating the epithelium is specially adapted for its function. IgA plasma cells produce monomeric IgA, which is converted into a dimer by a smaller ‘joining’ peptide (J chain), also produced by the plasma cells. The polymeric immunoglobulin receptor is synthesized by epithelial cells and is essential for transport of secretory IgA into the lumen of the gut (Fig. 14.4). The receptor binds the dimeric IgA, the complex is endocytosed and transported through the cytoplasm to the luminal surface of the cell where proteolysis of the polymeric Ig receptor occurs. As a result, the IgA dimer is released into the gut attached to a 70-kDa proteolytic fragment of the receptor, called the secretory component. Secretory component stabilizes the secretory IgA molecule and protects it from proteolytic attack by enzymes in the gut. Secreted dimeric IgA neutralizes viruses, bacteria and toxins, prevents the adherence of pathogenic microorganisms to gut epithelium and so blocks the uptake of antigen into the systemic immune system – a role termed ‘immune exclusion’.
Fig. 14.4 Synthesis and transport of secretory IgA through the gut epithelial cells into the gut lumen.
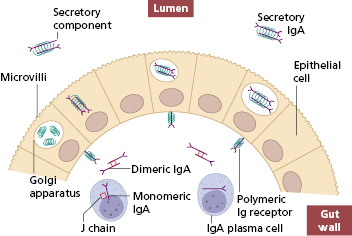
There is a similar migration pathway for T lymphocytes whereby activated T cells from mesenteric nodes ‘home’ both to the epithelium and to the lamina propria. Intraepithelial lymphocytes (IELs) express both non-rearranged innate immune receptors and rearranged adaptive immune receptors and have been conserved throughout vertebrate evolution. Their importance is underlined by the fact that they populate the gut before birth, unlike conventional T cells. Peripheral blood T cells rarely express the human mucosal lymphocyte antigen 1 (HML-1) but nearly all IEL do (Fig. 14.5). HML-1 (CD103) is an adhesion molecule of the β7-integrin family. It is important in the homing of IEL, allowing IEL to bind via HML-1 to its ligand, E-cadherin, expressed on epithelial cells.
Fig. 14.5 Typical phenotypes of gut lymphocytes. CD69, activation marker; CD25, activation marker; CD45 RO, memory cell marker.
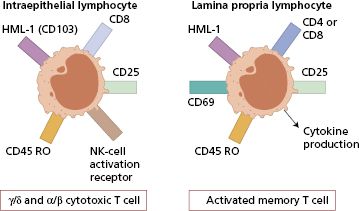
IELs are not a homogeneous population: they comprise at least three different T-cell phenotypes. The major population is CD8+ and shows increased expression of the γ/δ form of the T-cell receptor (TCR) when compared with peripheral lymphocytes. In contrast to the epidermis where TCR-γδ T cells are the sole population of lymphocytes, there are also TCR-αβ T cells in the respiratory and intestinal tracts; the third subset expresses neither CD4 nor CD8 and is not found elsewhere in lymphoid organs. IELS of the γδ T-cell type have limited TCR diversity but are able to respond immediately, in a manner similar to innate cells, avoiding a priming step before secreting cytokines.
Some IELs are cytotoxic and some have natural killer cell activity, functions important in the control of gut viruses particularly enteroviruses. IELs also seem to have a role in controlling epithelial cell barrier function, i.e. ‘restitution’.
Large numbers of lymphocytes, natural killer cells, mast cells, macrophages and plasma cells are seen in the lamina propria. T and B lymphocytes are both found, but T cells predominate in a ratio of about four to one. These T cells do not proliferate well after stimulation of the T-cell receptor, yet produce large amounts of the cytokines interleukin (IL)-2, IL-4, interferon (IFN)-γ and tumour necrosis factor (TNF)-α. Such lymphocytes express at least two molecules essential for homing to the gut: α4β7 integrin and the chemokine receptor CCR9. MAdCAM-1 (see section 1.2.5). The ligand for α4β7, is expressed widely in gut mucosal vessels and is the predominant adhesion molecule in the intestinal lamina propria.
Many similarities exist between the mucosal lymphoid tissues of the gut and organs such as the bronchus, breasts, salivary glands and uterine cervix. Lymphoblasts from any of these sites will repopulate all mucosa-associated lymphoid tissue in irradiated animals, with a selective preference for the organ of origin. If antigen is fed to lactating females, specific IgA antibodies appear in the milk, and gut-derived lymphoblasts home to breast tissue, lungs and parotid glands as well as back to the gut. There is evidence, therefore, of a common mucosal immune system as well as site-specific lymphocyte homing. This has at least one important implication: it may eventually prove possible to provide immune protection at one mucosal site by immunization at another.
14.1.2 Spectrum of the intestinal immune response
Ingestion of antigens can lead to a local or systemic immune response, or oral tolerance (specific immune unresponsiveness).
The gut can mount a local immune response to an antigen independent of a systemic response. For example, immunization against poliomyelitis with oral attenuated Sabin vaccine typically gives better protection than the injected killed Salk vaccine, even though both induce serum antibodies of IgG and IgA class. Local IgA antibody, produced in response to the oral vaccine, partly blocks uptake of pathogenic virus into the circulation. However the disadvantage of the oral vaccine was that the attenuated (but still living virus) could revert to wild type and cause poliomyelitis in immunocompromised recipients, so this is no longer used in view of the WHO programme to eradicate poliomyelitis worldwide.
A range of macromolecules and particles are absorbed by the intestine into the portal or systemic circulations, via either the glandular epithelium covering the villus or the specialized M cells. Up to 2% of a dietary protein load can appear antigenically intact in the circulation. Sinusoidal phagocytes (Kupffer cells) of the liver destroy much of the antigen, but enough passes through the liver to stimulate systemic antibody production, particularly in the spleen. Antibody formed in the spleen goes directly into the portal circulation to complex with incoming antigen. Circulating immune complexes of IgA with dietary antigens are regularly found in normal people after meals.
A unique feature of the mucosal immune system is its ability to downregulate immune responses to dietary antigens – oral tolerance. In animal models, oral feeding of an antigen followed by systemic antigenic challenge results in marked reduction of antibody levels and cell-mediated immunity compared with animals not fed the antigen first. This has led to attempts to treat autoimmune diseases in humans by feeding autoantigens to patients (see Chapters 5 and 7).
Normally the intestinal immune system steers a delicate course between the undesirable extremes (Table 14.1) of immune incompetence, with resulting vulnerability to ingested pathogens [e.g. infective diarrhorea due to other non-pathogenic bacteria] and hypersensitivity, with damage each time the relevant antigen is eaten (e.g. coeliac disease). Beneficial homeostasis is achieved by IELs responding to epithelial stress signals to maintain the balance of the intestinal bacterial load and IELs are directly involved in epithelial cell growth and repair. Most of the data are obtained from mouse studies and supported by observations in humans, but experimental data on human tissue are being developed and initial studies show similar results.
Table 14.1 Some examples of immunological involvement in intestinal disease
1 Infection with virulent organism | Gastroenteritis |
2 Immunodeficiency, e.g. primary or secondary | Chronic infection with enteropathogens Intestinal tumours |
3 Autoimmunity, e.g. gastric atrophy | Pernicious anaemia |
4 Hypersensitivity to dietary antigen | Coeliac disease |
Food allergy |
14.2 Infection and the gut
14.2.1 Infection and the gut
Infections of the gastrointestinal tract range from infectious diarrhoea that can be life threatening, especially in immunodeficient children and elderly people with comorbidities, to mild food poisoning in otherwise healthy individuals. In addition, systemic viruses can cause gastrointestinal lesions as well as enteropathogens.
An important example is the involvement of the intestinal tract in HIV infection. About half of all patients with HIV infection have gastrointestinal involvement that causes serious morbidity. Diarrhoea, malabsorption and weight loss are the most common manifestations and, in Africa, this wasting syndrome is called ‘slim disease’. HIV infects lymphocytes and macrophages within the gut as well as the intestinal cells. Impairment of the intestinal immune response, with consequent chronic infection with enteropathogens and the development of intestinal tumours such as lymphoma or Kaposi’s sarcoma (Fig. 14.6), adds to the considerable morbidity. The principal change in the small intestine is a partial villous atrophy detectable early in the natural history of HIV infection. Breastfeeding may transmit HIV in humans, suggesting that the intestine is an important portal of entry for HIV. Enteropathogens causing intestinal infections in HIV patients are of the same types as in immunocompetent subjects (Fig. 14.7), but the clinical symptoms produced by these infections are chronic, lasting for the duration of the patient’s life. Treatment of the bacterial infections remains a major problem, since some of the organisms (such as Cryptosporidia) cannot be eradicated by available antibiotics. Furthermore viral causes (such as rotavirus) are important too.
Gut infections in otherwise healthy individuals are usually self-limiting unless the pathogen, such as Salmonella, is particularly virulent. However in children with T-cell defects (such as severe combined immunodeficiency) or particular susceptibility to given organisms (such as interferon defects) (see Chapter 3) such infections may be life-threatening. Premature neonates and elderly people are also vulnerable, especially if invasive interventions or broad-spectrum antibiotics have been administered, resulting in Group b streptococcal infection in the former or Clostridia difficile in the latter.

A 67-year-old widow presented with gradually increasing tiredness, exertional dyspnoea and ankle swelling. Two years earlier she had been found to be anaemic and had been treated with oral iron without symptomatic improvement. She had lost 6 kg in weight, but denied any history of anorexia, dyspepsia or blood loss. On examination, she was very pale and had signs of congestive cardiac failure.
Laboratory investigations showed a very low haemoglobin of 54 g/l with a reduced white cell count of 3.7 × 109/l (and a platelet count of only 31 × 109/l). A blood film showed marked macrocytosis with a mean cell volume of 112 fl. Bone marrow examination revealed marked megaloblastic erythropoiesis with abundant iron stores. Serum vitamin B12 was 40 ng/l (NR 170–900) but serum folate, serum iron and total iron-binding capacity were normal. Her serum contained strongly positive gastric parietal cell antibodies of IgG class (Fig. 14.8) and blocking antibodies to intrinsic factor. Antibodies to thyroid microsomal antigen were also found, although the patient was clinically and biochemically euthyroid.
Fig. 14.8 Gastric parietal cell antibodies detected by indirect immunofluorescence (see Chapter 19).
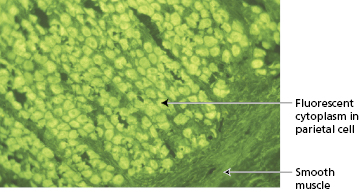
The patient had pernicious anaemia and was therefore started on intramuscular injections of hydroxycobalamin at 3-monthly intervals. Four days after the first injection, her reticulocyte count rose to 16%.
14.3 Gastritis
14.3.1 Atrophic gastritis and pernicious anaemia
The gastric mucosa contains several cell types: parietal cells producing acid and intrinsic factor, chief cells producing pepsinogen, epithelial cells, mucus neck cells and endocrine cells. Chronic inflammation of the gastric mucosa (gastritis) is relatively common, and associated with atrophy of gastric glands and loss of specialized secretory cells (Fig. 14.9). Two main types of gastritis are recognized (Table 14.2). Type A is immunologically mediated: type B gastritis is not autoimmune and usually caused by Helicobacter pylori infection. Autoimmune gastritis is associated with other organ-specific autoimmune disease, both in a given patient and in families (Table 14.3).
Fig. 14.9 Schematic representation of normal gastric mucosa and the histological changes seen in atrophic gastritis.
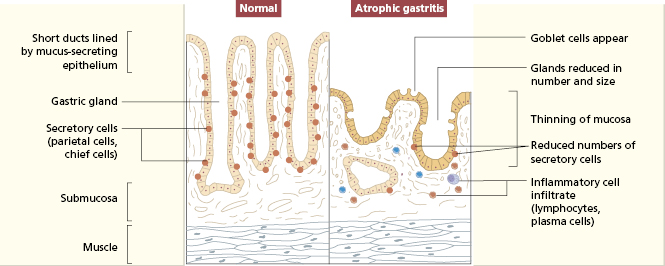
Table 14.2 Types of gastritis
Feature | Type A | Type B |
---|---|---|
Association | Pernicious anaemia | Helicobacter pylori infection |
Antral inflammation | Antral-sparing (fundus and body affected) | Antritis (fundus and body also affected) |
Antral gastrin cell counts | High | Low |
Serum gastrin level | High | Low |
Intrinsic factor production | Low | Normal |
Acid production | Low (achlorhydria) | Normal or low |
Gastric autoantibodies: | ||
Parietal cell | Present | Absent |
Intrinsic factor | Present | Absent |
Other autoimmune disease | Often present | Absent |
Risks of malignant disease | Gastric carcinoma | Gastric carcinoma |
(3× risk) | (2–6× risk) | |
B-cell gastric lymphoma |
Table 14.3 Features of pernicious anaemia (PA) consistent with an autoimmune aetiology
1 Presence of autoantibodies to:
|
2 In vitro inhibition of parietal cell function and inhibition of intrinsic factor by autoantibodies |
3 Association with other autoimmune disorders:
Thyrotoxicosis Hashimoto’s thyroiditis Myxoedema
|
4 Occurrence of PA/other autoimmune conditions in relatives:
|
5 Good experimental response to immunosuppressive drugs (not used clinically):
|
Pernicious anaemia (PA) is characterized by megaloblastic anaemia due to malabsorption of vitamin B12, itself secondary to deficiency of intrinsic factor secretion and gastric atrophy. Most patients are over 60 years old and women are affected more often than men (ratio 3 : 2) (Case 14.1). About 2% of people over 60 years old have undiagnosed PA. The most common presenting features are tiredness and weakness (90%), dyspnoea (70%), paraesthesia (30%) and a sore tongue (25%). Although neurological features due to B12 deficiency are relatively rare (about 5%), involvement of the posterior and lateral columns of the spinal cord (subacute combined degeneration) is a serious complication as the degeneration may not reverse on vitamin B12 treatment.
Over 90% of patients with PA produce an antibody to a parietal cell antigen independent of intrinsic factor. This antibody, gastric parietal cell (GPC) antibody, is commonly detected by indirect immunofluorescence (see Figure 14.8) and its molecular target is the gastric proton pump (H+,K+ATPase) contained within the membranes of the secretory canaliculi. These antibodies are unlikely to be pathogenic in vivo because gastric H+,K+ATPase is not accessible to circulating autoantibodies. GPC antibodies are found in nearly all patients with PA and provide a useful screening test, but because they can be present in other diseases and in healthy individuals, diagnosis depends on the demonstration of intrinsic factor antibodies or low serum B12 levels (Table 14.4).
Evidence from mouse models suggests that Th1 type CD4+ T cells initiate the autoimmune gastritis. Transfer of CD4+ T cell clones that recognize the β subunit of gastric H+,K+ATPase into naive mice results in gastritis and serum antibodies to the target antigen. However this may not be the initial pathogenesis since transfer of autoantibodies and CD8+ T cells has no such effects. The immunopathology responsible for gastritis of PA in humans is not known. Gastrin acts to stimulate gastric acid secretion and is a known growth factor for human gastrointestinal cancer including stomach cancer, for which pernicious anaemia is a recognized risk factor. Autoantibodies to gastrin receptors have been detected in patients with pernicious anaemia but the precise pathogenesis and the role of stimulating or blocking antibodies has yet to be worked out.
Intrinsic factor (IF) is a 60-kDa glycoprotein produced by gastric parietal cells. It binds avidly to dietary vitamin B12 and the B12–IF complex is carried normally to the terminal ileum where it is absorbed after binding to intrinsic factor receptors on ileal cells. Malabsorption of vitamin B12 in patients with PA is due to intrinsic factor deficiency and two mechanisms are responsible. First, the progressive destruction of parietal cells leads to failure of IF production. The severity of the gastric lesion correlates with the degree of impairment of IF secretion. Second, patients with PA produce autoantibodies that impair absorption of the B12–IF complex in the ileum. Two types of IF antibody are recognized (Fig. 14.10). Blocking antibody is directed towards the combining site for vitamin B12 on IF. Binding antibody reacts with an antigenic determinant on IF distinct from the B12 combining site: this antibody can react either with free intrinsic factor or with the B12–IF complex to inhibit absorption (Fig. 14.10). Binding antibody rarely occurs without blocking antibody. Blocking antibody is readily detected in serum by radioimmunoassay or ELISA, but large amounts of free B12 in the circulation (e.g. after B12 injection) can produce a false-positive result.
Fig. 14.10 Types of intrinsic factor antibody and their effect on vitamin B12 absorption. IF, Intrinsic factor.
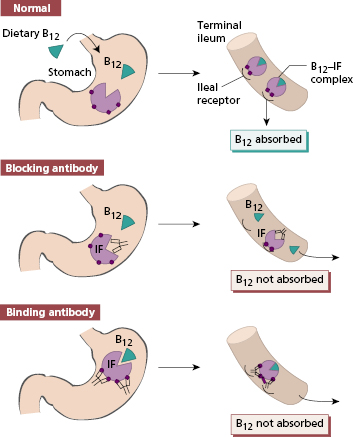
Although IF antibodies are found more frequently in the gastric juices than in the sera of patients with PA (Table 14.4), it is more convenient to test sera. Antibodies to IF are specific and rarely occur in the absence of overt or latent PA.
14.4 Food-induced gastrointestinal disease
14.4.1 Food allergy
Doctors and the public have different perceptions of food allergy. Up to 20% of ‘food-allergic’ adults believe their symptoms to be food dependent, although the objective confirmation of prevalence is nearer 1%. A limited number of foods are responsible for true food allergy; the patient’s history provides most of the diagnostic clues. Some patients have acute, life-threatening anaphylactic reactions, e.g. peanut allergy, while others have localized gastrointestinal reactions with diarrhoea, bleeding and failure to thrive, e.g. children with ‘cow’s milk protein intolerance’.
There are circumstances where patients experience distressing food-related symptoms that cannot be confirmed by objective tests or food challenge. Many of these cases have atypical or non-specific symptoms and involve foods rarely implicated in allergic reactions. It is inappropriate to label such patients as having food allergy without evidence of an immunological reaction to a food.
Food allergy and intolerance is discussed more fully in Chapter 4.
14.4.2 Coeliac disease
Coeliac disease, or gluten-sensitive enteropathy, is a relatively common small bowel enteropathy resulting from immunological hypersensitivity to ingested gluten (the storage protein of wheat and related cereals) in genetically susceptible individuals. Coeliac disease is most prevalent in Europeans and rare in Chinese or Afro-Caribbean people. Population screening surveys based on autoantibody testing have suggested a prevalence of 2% in the UK and 1% by biopsy; worldwide prevalence is probably twice these rates.

A 35-year-old school cook presented to her dentist with a 30-month history of a sore mouth and tongue; she was treated with triamcinolone oral paste without improvement. Three months later she developed loose stools and generalized but vague abdominal pain. On questioning, she had felt tired for 2 years and had lost 8 kg in weight during the preceding 6 months despite a good appetite. During her second and third pregnancies she had developed moderate folic acid-deficiency anaemia. There was no family history of gastrointestinal disease and no abnormalities were found on examination.
Laboratory investigations showed a macrocytic anaemia but normal white cell, platelet and reticulocyte counts. The blood film showed many Howell–Jolly bodies (fragments of nuclear material within red blood cells) (Fig. 14.11), suggestive of hyposplenism. Bone marrow examination revealed active erythropoiesis with early megaloblastic features but no stainable iron. The appearances were those of a combined deficiency of iron and folate/vitamin B12; laboratory tests confirmed this (Table 14.5). In view of the blood film and the malabsorption of fat, coeliac disease was the most likely diagnosis. Her serum was positive for IgA antibodies to endomysium and to tissue transglutaminase, strongly supporting the clinical diagnosis. A jejunal biopsy was performed: this showed a convoluted pattern of stunted villi under the dissecting microscope, and subtotal villous atrophy with marked increase in intraepithelial lymphocytes and chronic inflammation in the lamina propria (Fig. 14.12).
The patient was started on a strict gluten-free diet with folic acid, iron and calcium supplements. One year later, she had put on 4.8 kg. A repeat jejunal biopsy showed improvement in villous architecture. This improvement following gluten withdrawal confirmed the diagnosis of coeliac disease and the patient will continue her gluten-free diet for life.
The characteristic histological lesion in untreated cases is the loss of normal villi with a marked increase in the numbers of IELs, particularly those expressing the γ/δ T-cell receptor (Figs 14.12 and 14.13); the infiltrate resolves following elimination of gluten from the diet, suggesting that the intestinal damage may be caused by a local cell-mediated reaction to gluten or a derivative. Within the lamina propria there is a mixed-cell infiltrate of plasma cells, stimulated CD4+ lymphocytes, macrophages, mast cells and basophils.
Fig. 14.12 Typical histological features of coeliac disease shown in (a) low and (b) high power. Intraepithelial lymphocytes are arrowed.
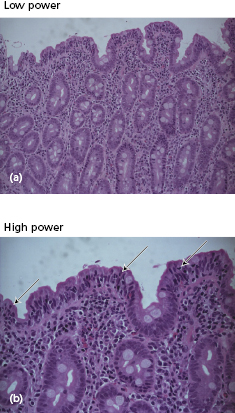
Fig. 14.13 Schematic representation of normal jejunal mucosa and histological changes in patients with coeliac disease.
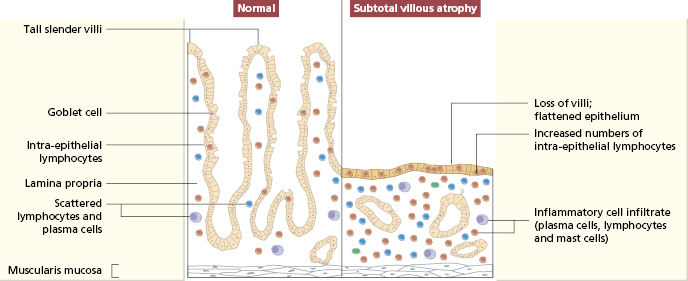
Coeliac disease runs in families: 10–20% of first-degree relatives, 40% of HLA-identical siblings and around 75% of monozygotic twins are affected. In Europeans, coeliac disease is strongly associated with inheritance of the histocompatibility antigens HLA-DQ2 and HLA-DQ8: most coeliac patients carry these risk alleles but they are also found in 20% of the general population. Together, the HLA-DQ2 and HLA-DQ8 alleles are estimated to account for 40% of the genetic risk in coeliac disease. Consequently, it is likely that at least one other, non-HLA-linked gene, maybe on the long arm of chromosome 5, is involved in disease susceptibility. The absence of complete concordance in identical twins implies that other factors are involved, presumably environmental. Whatever these factors are, patients with coeliac disease remain sensitive to gluten for the rest of their lives.
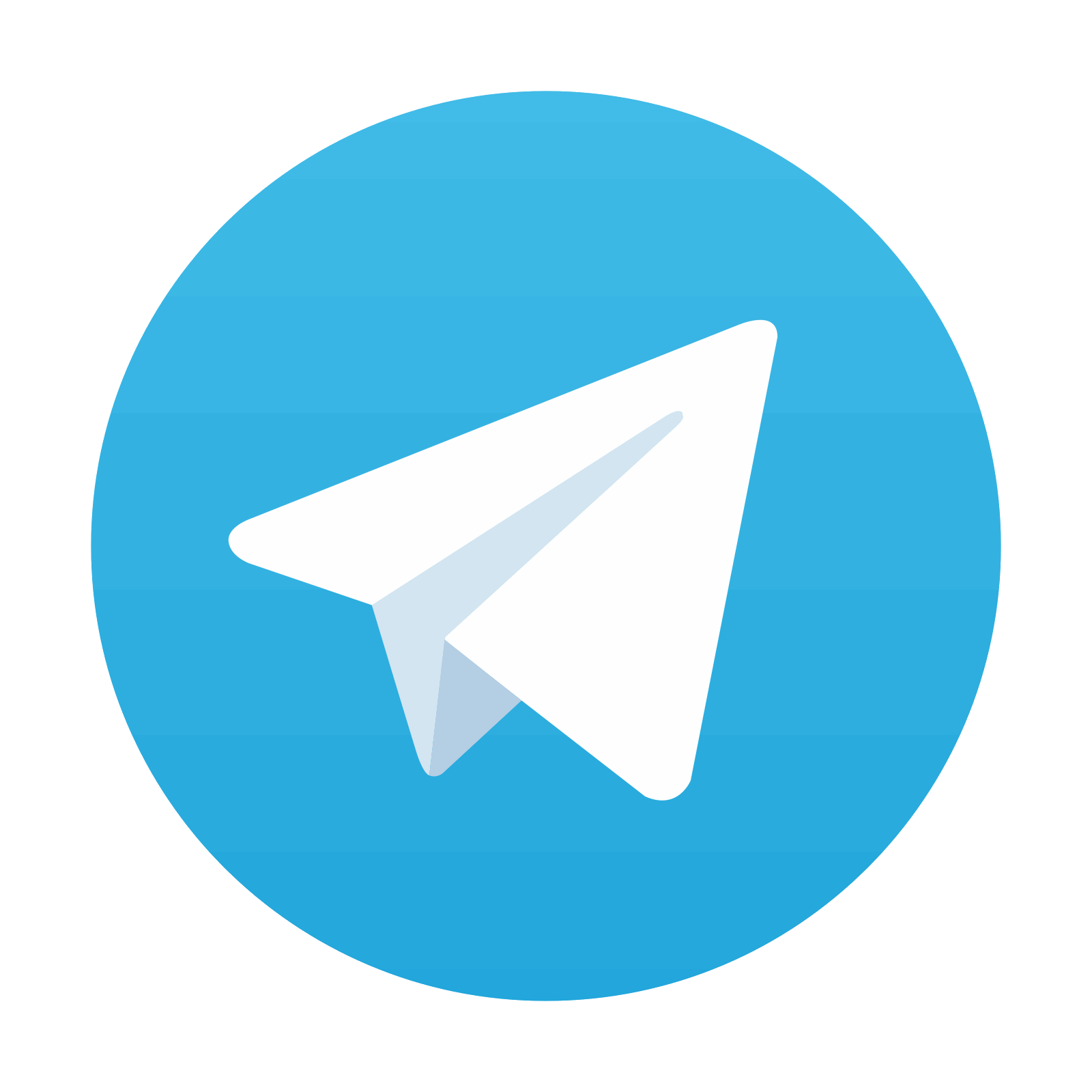
Stay updated, free articles. Join our Telegram channel

Full access? Get Clinical Tree
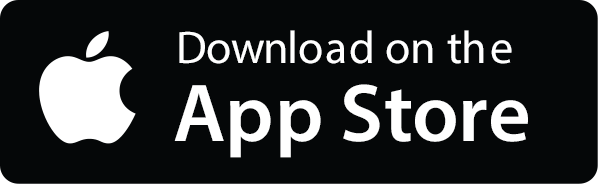
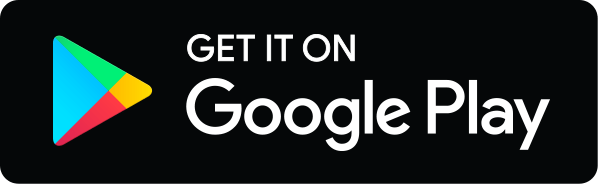