Abstract
Many molecular genetic abnormalities have been recognized in the setting of anterior pituitary adenomas. However, pituitary adenomas with heritable genetic causes are rare and have been described most often in the setting of an endocrine tumor syndrome, such as multiple endocrine neoplasia type 1 (MEN1) and Carney complex (CNC). MEN1 is an autosomal dominant condition that is associated with the occurrence of parathyroid, enteropancreatic, and anterior pituitary tumors. Endocrine-inactive tumors, such as lipomas and angiofibromas, are also frequently seen in MEN1 patients. The MEN1 gene on chromosome 11q13 encodes the nuclear protein, menin. MEN1 appears to act as a tumor suppressor gene, and recent data suggest that menin can potentially interact with thousands of genes, 3′ sites and in chromatin. To date, over 500 different individual mutations in the MEN1 gene have been described, most of which predict a truncated menin protein. However, in 20–30% of cases suggestive of MEN1 clinically, the MEN1 sequence is normal. About 40% of patients with MEN1 have pituitary adenomas, and 17% of cases present with a pituitary tumor. Patients with pituitary tumors present earlier than patients presenting with other MEN1 tumors. In families with MEN1, pituitary tumors are more frequent than in sporadic MEN1. From a clinical perspective, MEN1-associated pituitary adenomas are more aggressive and less responsive to treatment than sporadic non-MEN1 tumors.
Keywords
MEN1, pituitary adenomas, menin, Carney complex (CNC), aryl hydrocarbon receptor interacting protein gene ( AIP ), somatotropes, familial isolated pituitary adenomas (FIPA), MEN4, X-LAG syndrome, GPR101, pituitary gigantism
Introduction
Many molecular genetic abnormalities have been recognized in the setting of anterior pituitary adenomas. However, pituitary adenomas with heritable genetic causes are rare and have been described most often in the setting of an endocrine tumor syndrome, such as multiple endocrine neoplasia type 1 (MEN1) (see Chapter 23 ) and Carney complex (CNC). MEN1 is an autosomal dominant condition that is associated with the occurrence of parathyroid, enteropancreatic, and anterior pituitary tumors. Endocrine-inactive tumors, such as lipomas and angiofibromas, are also frequently seen in MEN1 patients. The MEN1 gene on chromosome 11q13 encodes the nuclear protein, menin. MEN1 appears to act as a tumor suppressor gene, and recent data suggest that menin can potentially interact with thousands of genes, 3′ sites and in chromatin. Up to 2010, over 500 different individual mutations in the MEN1 gene have been described, most of which predict a truncated menin protein. However, in 20–30% of cases suggestive of MEN1 clinically, the MEN1 sequence is normal. About 40% of patients with MEN1 have pituitary adenomas, and 17% of cases present with a pituitary tumor. Patients with pituitary tumors may present earlier than patients presenting with other MEN1 tumors. In families with MEN1, pituitary tumors are more frequent than in sporadic MEN1. From a clinical perspective, MEN1-associated pituitary adenomas are more aggressive and less responsive to treatment than sporadic non-MEN1 tumors.
CNC is rare, with less than 1000 cases reported to date. CNC, usually familial, consists of patients with a complex of skin pigmentation, cardiac myxomas, endocrine hypersecretion, and schwannomas. The primary genetic cause of CNC is mutation of the protein kinase A regulatory subunit 1 A gene ( PRKAR1A ) on chromosome 17q22-24. Acromegaly occurs in the setting of CNC, although infrequently. Most patients have evidence of abnormal growth hormone (GH), insulin-like growth factor-1 (IGF-1), or prolactin levels.
A MEN1-like syndrome (MEN4) has been reported recently in rats and in humans, and relates to mutations in the CDKN1B gene that encodes p27 kip1 . To date less than 10 patients with MEN4 that have pituitary adenomas have been described in the literature and it is a rare cause of pituitary adenomas.
Isolated pituitary adenomas also occur in an inherited setting in the absence of MEN1 and CNC. Familial isolated pituitary adenomas (FIPA; >/=2 pituitary adenoma patients occurring in related members of the same kindred in the absence of MEN1, MEN4, or CNC) have been described in more than 200 since 1999. Within FIPA, patients may have pituitary adenomas of any functional or nonfunctional type, and different tumor types may occur within different members of the same family. Previous studies on familial cases of acromegaly pointed to a region of chromosome 11q13.1-q13.3 as being involved. Subsequently, mutations in the aryl hydrocarbon receptor interacting protein gene ( AIP ) were noted to occur in association with familial acromegaly/prolactinoma kindreds. AIP mutations account for about 15% of FIPA families and 50% of familial acromegaly kindreds, indicating that other genetic factors remain to be described. AIP mutations are also associated with larger/more aggressive pituitary tumors that occur at an earlier age than non- AIP mutated cases; a relatively high frequency of AIP mutations occur in sporadic (nonfamilial) cohorts with acromegaly or other pituitary adenomas with a young age at onset and an aggressive clinical history.
Recently a novel X-linked genomic disorder consisting of early childhood-onset gigantism with excessive secretion of GH due to pituitary hyperplasia or adenoma was described. This gigantism cohort was characterized by heritable duplications in chromosome Xq26.3 containing four duplicated genes (CD40LG, ARHGEF6, RBMX, and GPR101). GPR101 is the gene most likely responsible for GH oversecretion and gigantism in these patients.
Genetic pathophysiology of pituitary adenomas
Most pituitary adenomas arise as a clonal expansion from a single mutated anterior pituitary cell, which can be accompanied by a wide variety of genetic and molecular alterations in adenomatous pituitary tissue. At the tissue level, however, the picture can be somewhat complicated, as a single pituitary can contain multiple tumors or hyperplastic areas, each with its own clonal origin and specific pattern of growth, apoptosis, and pathological features. The development of a pituitary adenoma is dependent on a variety of tumor suppressor genes and oncogenes ( Table 4.1 ). The most important oncogene involved in sporadic pituitary tumorigenesis is gsp , which encodes the Gsα subunit, a stimulatory guanine binding protein that regulates hypothalamic GH-releasing hormone effects in somatotropes. Biallelic expression of mutated gsp can lead to endogenous activation of adenylate cyclase and elevated levels of cyclic adenosine monophosphate (cAMP). Mutations in gsp have been most closely associated with somatotropinomas, and they are found to occur in up to 40% of these tumors. The oncogene ras has also been implicated in pituitary tumorigenesis, although in a very small number of cases. Mutations in ras appear to be associated with high levels of tumor aggression and have been noted to occur among rare pituitary carcinomas. Pituitary tumor transforming gene (PTTG) is a gene that is usually poorly expressed in normal pituitary, but is upregulated in most pituitary tumor types.
Genes | Defects |
---|---|
Cyclin D1 | Overexpression in nonsecreting adenomas and somatotropinomas |
Gsp | Somatic activating mutations in up to 40% of somatotropinomas |
Mosaicism in McCune–Albright syndrome (somatotropinoma, somatomammotropinoma, and Cushing’s syndrome in association with precocious puberty, hyperthyroidism, and dermal and bony lesions) | |
PRKAR1A | Truncation mutations in Carney’s complex leading to somatolactotrope hyperplasia and adenomas |
Pdt-FGFR4 | Alternative transcription initiation in pituitary adenomas |
PTTG | Increased expression in more aggressive pituitary tumors |
BMP-4 | Diminished expression in prolactinoma |
GADD45G | Promoter methylation in nonsecreting adenomas, prolactinomas, and somatotropinomas |
MEG3a | Promoter methylation in nonsecreting adenomas and gonadotropinomas |
MEN1 | Inactivating mutations in all pituitary adenoma types |
PKC | Point mutations in invasive pituitary adenomas |
p16 | Promoter methylation in pituitary adenomas |
CDKN1B (p27Kip1) | Germline heterozygous nonsense mutation in MEN4, a novel, rare MEN1-like syndrome |
Retinoblastoma | Promoter methylation in pituitary adenomas |
ZAC | Promoter methylation in nonfunctioning adenomas |
AIP | Germline mutations and loss of heterozygosity in 15% of FIPA cases. Seen in familial/sporadic somatotropinomas, somatolactotrope adenomas, prolactinomas, nonsecreting adenomas, and Cushing’s disease (sporadic only) |
GPR101 | Duplication in early onset gigantism patients with somatotropinoma or pituitary hyperplasia; a pathogenic cause in certain AIP negative FIPA kindred with acrogigantism. Mutation p.E308D in some sporadic acromegaly patients, mostly in tumor DNA. |
USP8 | Somatic recurrent mutations seen in Cushing disease |
Mutations in tumor suppressor genes have been identified in the setting of pituitary adenomas and tumorigenesis. The best known among these is the gene MEN1 that is responsible for MEN1, which is discussed in detail in the subsequent section, as it may lead to inherited disease and is relevant for screening. Heterozygotic retinoblastoma gene ( Rb ) mutation status is associated with the development of pituitary adenomas in mice. However, in humans the role of Rb is less certain. Somatic Rb loss may occur in occasional pituitary adenomas, while Rb promoter hypermethylation has also been reported.
Cell cycle regulators have also been implicated in pituitary tumorigenesis and development. Cyclin D1, which regulates the transition from G 1 to S-phase is overexpressed in nearly 40% of somatotropinomas (and 70% of nonfunctional pituitary adenomas). CCND1 genotypes are related to tumor grades seen in pituitary adenomas. In pituitary adenomas, the cyclin-dependent kinase inhibitor p16 is heavily downregulated due to gene promoter hypermethylation. The cyclin dependent kinase inhibitor p27 kip1 appears to play an important role in pituitary tumorigenesis, as evidenced by data from a knockout mouse that show the development of specific patterns of pituitary adenomas and other abnormalities. The relevance of mutations in the CDKN1B gene that encodes p27 kip1 are discussed in the subsequent section as they may be rarely associated with inherited endocrine tumors, including pituitary adenomas.
The protein ZAC is normally expressed at high levels in healthy pituitary tissue. In pituitary adenomas (predominantly nonsecreting tumors), ZAC expression is strongly reduced. The somatostatin analog, octreotide, functions in somatotropinomas in part via ZAC as it increases the expression of the gene Zac1 . Fibroblast growth factor receptors (FGFR) play a role in the growth and development of many tissues. A truncated pituitary tumor-derived form of FGFR4 has been identified in humans and was reported be associated with invasive pituitary tumorigenesis in a transgenic mouse model.
MEG3 appears to play a role as a growth suppressor in pituitary tissue; a pituitary-derived variant is absent from both functional and nonsecreting pituitary adenomas, potentially due to promoter hypermethylation. Expression of the growth arrest and DNA damage-inducible gene ( GADD45G ) is decreased in somatotropinomas, prolactinomas, and nonsecreting adenomas. Bone morphogenetic protein-4, which may indirectly stimulate c-myc expression, is overexpressed in prolactinomas as compared with other tissues.
While a wide variety of genetic mutations and molecular abnormalities have been implicated in tumorigenesis in functioning pituitary adenomas, only a handful are of clinical relevance for genetic screening and testing ( Table 4.2 ). These clinically relevant genes for human screening are associated with a variety of presentations of pituitary adenomas in combination with other tumors, or occasionally as isolated pituitary adenomas. The status of these conditions from a screening perspective is also heterogeneous, as some are relatively recent discoveries over the last few years, while others have been recognized for more than a decade and have consensus guidelines.
Condition | Gene | Molecular pathology | Pituitary tumor |
---|---|---|---|
MEN1 | MEN1 (Ch11q13) | Decreased menin expression/function | All pituitary tumor types (prolactinomas, nonsecreting adenomas, and GH-secreting adenomas most frequent) |
MEN4 | CDKN1B (Chr 12p13) | Decreased p27 levels in tumor | Associated with only acromegaly and Cushing’s disease in two patients to date |
CNC | PPKR1A (Ch17q22-24) ? (Ch2p16) | Decreased protein kinase A regulatory subunit Ia expression/function | GH and GH/prolactin secreting adenomas |
FIPA | AIP (Ch11q13.32) in 15% of cases (50% of familial acromegaly) X-LAG syndrome (chromosome Xq26.3 microduplications; GPR101) | Decreased mRNA and protein in some mutated tissues. ?Altered regulation of AhR or phosphodiesterase function Increased expression of GPR101 and growth hormone secretion | All pituitary adenoma subtypes involved; AIP mutation-associated cases include somatotropinomas, prolactinomas, mixed GH/prolactin tumors, and nonsecreting adenomas Early onset acrogigantism, somatotropinomas/somatotroph hyperplasia with prolactin cosecretion |
Genetic screening in functioning pituitary adenomas
Currently, genetic screening for specific mutations in patients with functioning pituitary adenomas is limited to a minority of cases in which suggestive pathological features are present. Pituitary adenomas that occur in a familial setting account for no more than 4–5% of all pituitary adenomas. Scheithauer et al. estimated that 2.7% of pituitary adenomas were due to multiple endocrine neoplasia type I (MEN1). Our data suggest that a further 2% of pituitary tumor cases have family links. Widespread genetic screening is, therefore, not warranted in the vast majority of patients with sporadic (i.e., nonfamilial) pituitary adenomas that do not have associated endocrine/nonendocrine tumors in the same patient or in their family. This is particularly relevant from a healthcare resource utilization perspective, as clinically active pituitary adenomas are not rare, and occur with a prevalence of approximately one in every 1000 of the population in developed countries.
MEN1
The history of pituitary tumors in the setting of multiple endocrine neoplasia dates back to as early as 1903, with the description by Erdheim of a patient with adenomas in the parathyroid and pituitary. Later in the 1950s, Wermer described a family with four sisters affected with pituitary adenomas (one had acromegaly), hypercalcemia, and adenomatosis of the pancreas and gut. Further investigation revealed that the father had evidence of multiple pancreatic and gut tumors on autopsy. After analyzing this and many isolated cases, Wermer correctly posited an autosomal dominant mode of inheritance for this condition, which would later be termed MEN1. For further information on MEN1, see Chapter 23 .
MEN1-related pituitary tumors
About 40% of patients with MEN1 have pituitary adenomas, and this was the presenting tumor in about 17% of cases in one large series. Such patients that presented with a pituitary adenoma did so 7 years before patients presenting with enteropancreatic lesions. Among familial MEN1 cases, pituitary disease was significantly more frequent than in nonfamilial MEN1 cases (59% vs. 34%, respectively). Females with MEN1 have a somewhat increased chance of having a pituitary adenoma. The characteristics and features of MEN1 and non-MEN1-related pituitary tumors are outlined in Table 4.3 .
Characteristics | Pituitary adenoma | ||
---|---|---|---|
MEN1 | Non-MEN1 | P | |
Age (year) | 38.0 ± 15.3 | 36.2 ± 14.6 | NS |
Mean follow-up (year) | 11.1 ± 8.7 | 10.0 ± 6.3 | NS |
Adenoma type | |||
Prolactinoma | 85 | 68 | NS |
GH-secreting | 12 | 15 | NS |
ACTH-secreting | 6 | 7 | NS |
Cosecreting | 13 | 2 | NS |
Nonsecreting | 20 | 18 | NS |
Tumor size | |||
Micro ( n , %) | 19 (14%) | 64 (58%) | 0.001 |
Macro ( n , %) | 116 (85%) | 46 (42%) | |
Clinical signs due to tumor size ( n , %) | 39 (29%) | 15 (14%) | 0.01 |
Normalization of pituitary hypersecretion ( n , %) | 49 (42%) | 83 (90%) | 0.001 |
Prolactinomas predominate among both MEN1-associated and non-MEN1 pituitary adenomas, and the proportions of prolactinomas, GH-secreting, ACTH-secreting, nonsecreting, and cosecreting adenomas are similar between the MEN1 and non-MEN1 patients. MEN1-related prolactinomas are predominantly macroadenomas (84%) and higher rates of invasion are seen than in non-MEN1 prolactinomas. The response of MEN1-related prolactinomas to dopamine agonists is poor, with only 44% of patients being normalized.
Pituitary tumors in MEN1 appear to be larger and more aggressive than in patients without MEN1, with macroadenomas being present in 85% of the former, compared with only 42% of the sporadic cases. MEN1-associated pituitary tumors are significantly more likely to cause signs due to tumor size and have a significantly lower rate of hormonal normalization than non-MEN1 pituitary tumors.
Mutations of the MEN1 gene are not an important factor in the tumorigenesis of non-MEN1 sporadic pituitary adenomas. Theodoropoulou et al. found that menin was detectable in 67 of 68 sporadic non-MEN1 pituitary tumors. There is no recognized relationship between the site or type of genetic mutation in the MEN1 gene and the expressed MEN1 disease phenotype, although disease clustering and variations in severity have been recognized. Such clusters include the “prolactinoma variant” seen in kindreds from the Burin peninsula in Canada (MEN1 BURIN ).
Recommendations for Testing in MEN1
As MEN1 has been recognized clinically and genetically for some time, consensus guidelines for its investigation and management have been developed. These guidelines were updated in 2012 and advances in the availability of and access to DNA testing may allow for some practical simplification. Assessment of which patients to test for MEN1 germline mutations depends on their meeting the criteria for the disease (practically, two of the three constituent major affected tissues: parathyroid, enteropancreatic, or pituitary tumors). Assessment of family history is also useful to detect previously unrecognized contributory information; however, it is often practically difficult to exclude a familial case of MEN1 in small kindreds with few living relatives. For index cases in which MEN1 is suspected as a cause for their pituitary tumor, germline DNA analysis of the MEN1 gene would be recommended. In cases that are negative for mutations on first screening, the potential for further testing for large gene deletions in an investigational laboratory could be considered. In the case where a MEN1 mutation is found, then full family screening for clinical features and biochemical abnormalities (particularly hypercalcemia) is a good first step. However, as MEN1 is an autosomal dominant disease, it may be more cost effective to construct a genealogy and undertake genetic screening for the particular mutation discovered. This has the advantage of definitively identifying carriers quickly, and focusing resources and time on assessing their potential tumor expression. Also, mutation negative individuals and their descendents can be excluded from unnecessary further work-up. Carriers should be followed closely with regular biochemical, endocrine, and appropriate radiological screening for nascent tumors.
In familial MEN1, screening of potential carriers of a known mutation should not be age limited, as MEN1 can occasionally present in the pediatric setting or occult disease can be present for some time in some individuals. Again, DNA sequencing for the specific familial mutation, while potentially more expensive, is more definitive than biochemical or other clinically based methods and allows noncarriers to be excluded from further follow-up. Completion of MEN1 genealogies may benefit from sourcing and testing of relevant stored surgical pathological material in deceased members.
Carney complex (CNC)
J. Aidan Carney described a complex of myxomas, spotty pigmentation, and endocrine overactivity that included pituitary adenomas causing acromegaly in four of a total of 40 cases in his original series. This condition, termed Carney complex (CNC), is rare and has been described in about 500 people in the largest database. CNC is familial in 70% of cases, occurs in all racial groups, and has a slight female preponderance. Two gene loci have been identified, one on chromosome 17q22-24; the other is on chromosome 2p16. The former is associated with the gene encoding the protein kinase A regulatory subunit Iα ( PRKAR1A ); mutations in PRKAR1A have been identified in up to 60% of CNC patients. Most PRKAR1A mutations lead to mRNA instability, decreased or absent protein expression and PRKAR1A haploinsufficiency in CNC tumors. Loss of heterozygosity at 17q22-24 and allelic loss have been shown in CNC tumors, while the loss of PRKAR1A function enhances intracellular response to cAMP in CNC tumors. In knockout mouse models, the Prkar1a −/− state is lethal in embryonic life. In heterozygous Prkar1a +/− mice, no typical CNC features are encountered. However, a transgenic mouse with an antisense PRKAR1A exon 2 construct develops multiple endocrine abnormalities similar to CNC.
CNC-Related Pituitary Tumors
The main endocrine abnormalities seen in CNC are primary pigmented nodular adrenocortical disease (PPNAD), thyroid tumors and nodules, testicular tumors (large cell calcifying Sertoli cell tumor (LCCSCT), Leydig cell tumors) and acromegaly due to a pituitary adenoma. Acromegaly itself is seen in a minority of patients with CNC (10% of cases), but about 75% of patients exhibit asymptomatic elevations in GH, IGF-1 or prolactin levels, or abnormal responses to dynamic pituitary testing. A histologic analysis of pituitary tumors in CNC patients with acromegaly reported that all tumors were prolactin and GH positive, while a minority also stained for thyroid-stimulating hormone, luteinizing hormone, or alpha-subunit. A distinguishing feature of CNC-related acromegaly is multifocal hyperplasia of somatomammotropic cells that included nonadenomatous pituitary tissue within the tumors of CNC patients. The zones of hyperplasia were not well demarcated and exhibited increased cellularity and altered reticulin staining that merged with normal pituitary tissue. No consistent genetic abnormalities were seen on comparative genome hybridization. Electron microscopy showed that tumors from acromegalic patients with CNC demonstrate heterogeneous intracellular structure. Acromegaly in CNC develops insidiously and may begin in apparently normal somatomammotrope tissue that undergoes multifocal hyperplasia to form GH/prolactin-secreting adenomas. As in MEN1, sporadic pituitary tumors do not exhibit mutations of the PRKAR1A gene.
Recommendations for Testing in CNC
The diagnosis of CNC is clinical in the first instance, and patients displaying two or more of the following are generally considered to have CNC: PPNAD, cardiac myxoma, cutaneous myxoma, lentigines, blue nevi, LCCSCT, thyroid nodules/tumor, ovarian cysts, acromegaly, melanotic Schwann cell tumor, and osteochondromyxoma. In patients that meet these criteria for CNC, germline DNA testing for mutations in the PRKAR1A gene should be undertaken. In familial cases, patients with a diagnosed close relative with CNC and even one of the above manifestations can be considered as having CNC. Based on current experience, it is expected that one-half to two-thirds of patients with clinical CNC will have a germline PRKAR1A mutation in the heterozygous state. Up to 80% of those with familial CNC will have a PRKAR1A mutation. On diagnosing a new incident case of CNC, kindred clinical assessment should be undertaken, but as with the case in MEN1 described earlier, it may be more cost effective to undertake DNA screening. A detailed genealogical tree may allow for targeted testing for a known PRKAR1A mutation beginning with first-degree relatives. This permits resource allocation to those identified as carriers and allows those with a wild-type genotype to avoid costly and repetitive endocrine and cardiac interventions. Genetic and clinical studies should begin as early as possible and in infancy in at-risk individuals as the disease can manifest at a young age. Accurate and early diagnosis is important mainly due to the threat of sudden death caused by cardiac myxoma (potentially multiple, and occurring in any cardiac chamber), rather than by endocrine manifestations. Carriers of a PRKAR1A mutation should be screened clinically, hormonally, and/or with imaging studies at least yearly for all manifestations of CNC. With respect to the pituitary, patients should have GH, IGF-I, and prolactin secretion assessed on a yearly basis as a minimum. A baseline pituitary magnetic resonance image (MRI) should be undertaken, but the timing of repeat imaging should be based on clinical progression, or the appearance of new hormonal alterations. Diagnosis and management of acromegaly in the setting of CNC is the same as for sporadic non-CNC-related disease, with transsphenoidal neurosurgery and medical therapy (somatostatin analogs, dopamine agonists) representing the mainstream forms of treatment.
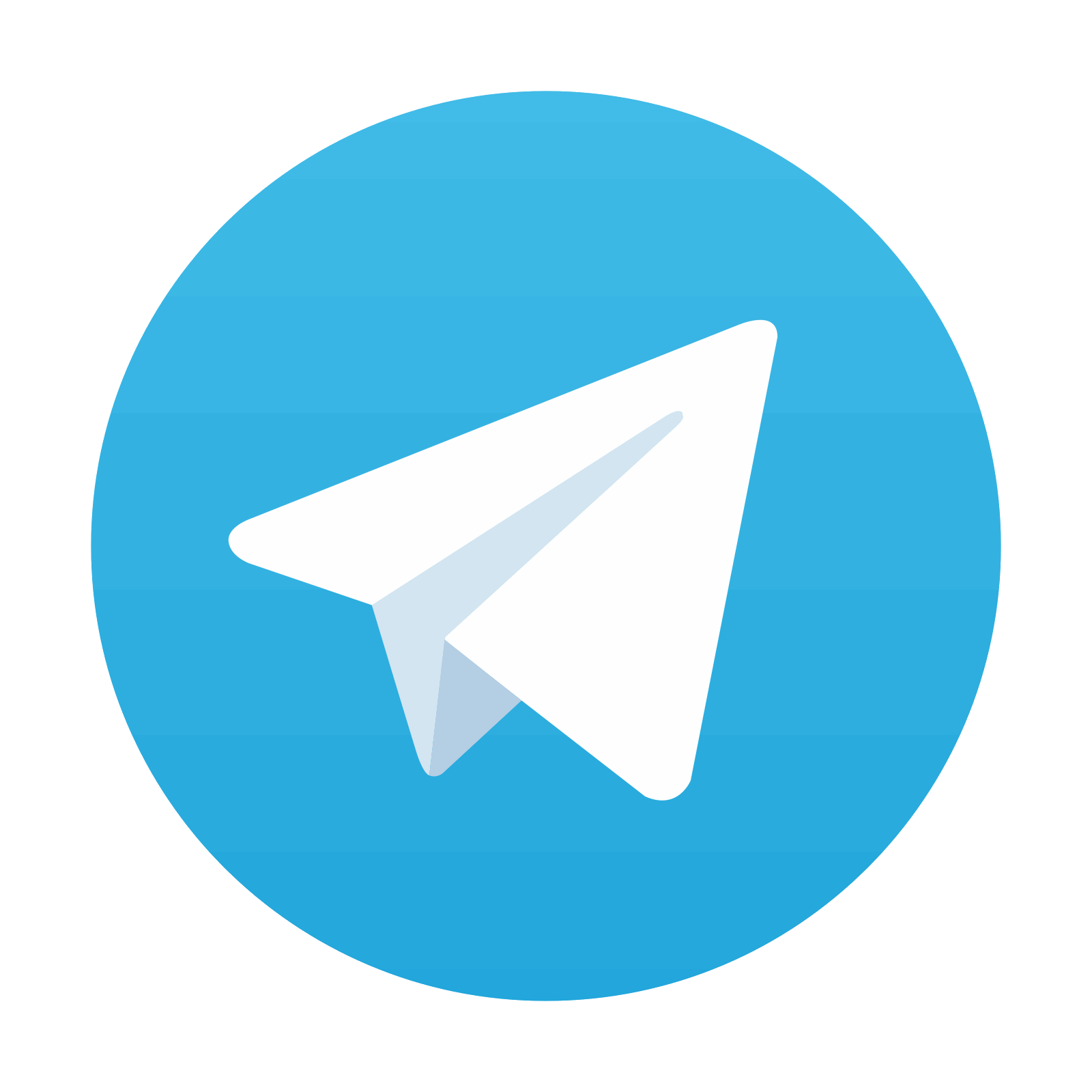
Stay updated, free articles. Join our Telegram channel

Full access? Get Clinical Tree
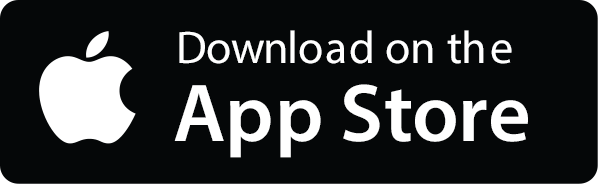
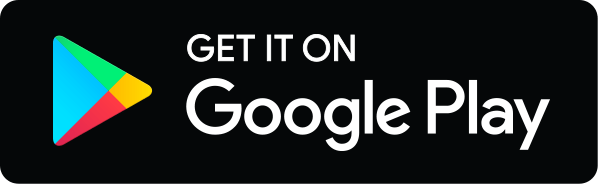