Fig. 1
Motor and sensory direct cortical stimulation data. Rendering of left and right hemisphere positive stimulation sites eliciting movement (green) and dysesthesias (yellow) within the precentral and postcentral gyri, respectively. Adapted from Tate et al. [41]
In addition to the contribution of M1 to the CST, premotor and supplementary motor cortices anterior to M1 in the frontal lobe also contribute to the CST. The exact function of these so-called “higher-order” motor areas are less well understood, but recent studies have given some insight into their functional contributions. The premotor cortex (PMC), corresponding to Brodmann Area 6, is composed of a dorsal part (dPMC) that includes the posterior part of the superior and middle frontal gyri just anterior to the precentral sulcus, and a ventral part (vPMC) that is defined by the precentral gyrus from the Sylvian fissure to the level of the superior frontal sulcus. PMC lesions result in proximal muscle weakness and difficulty with sequencing of movements of the contralateral limb [12]. DCS of the vPMC in patients undergoing awake craniotomy for tumor resection reliably causes disorders of articulation [13], while DCS in the left hemisphere points to a role in language. The supplementary motor area (SMA), also a part of Brodmann area 6, is located in the medial/posterior portion of the superior frontal gyrus just anterior to leg motor cortex. The SMA is thought to be involved in motor planning, as SMA stimulation causes complex contralateral movements and resection of the SMA produces a stereotypical “SMA-syndrome” characterized by impairment of volitional movements, hemineglect, and dyspraxia of the contralateral limbs with preservation of muscle tone. If the SMA of the dominant (language) hemisphere is involved, difficulty initiating speech may also be observed. Deficits caused by SMA syndrome typically resolve within a few months postoperatively [14, 15]. As with M1, the SMA also demonstrates somatotopy: leg representation is most posterior, face is most anterior, and the arm region is located in between [14]. The frontal eye field (FEF) of the frontal lobe is located within the middle frontal gyrus just anterior to dPMC and is involved in saccadic eye movement. Lesions of the FEF impair voluntarily contralateral gaze, and DCS may elicit conjugate eye movements toward the contralateral side [16, 17].
In addition to motor regions located in the frontal lobe, the parietal lobe is involved in tuning of movement (preparation, control, adjustment). For example, the primary somatosensory area (S1), discussed further in the sensory section below, can produce complex motor movements when stimulated. Other proposed parietal lobe functions include visuomotor transformation and coding of motor acts such as grasping [18].
At the subcortical level, the major descending pathway is the corticospinal tract (CST) which originates as axons of pyramidal neurons within the layer V of M1 that travel through the centrum semiovale, corona radiata, posterior limb of the internal capsule, cerebral peduncle, pyramidal decussation in the medulla, and then descends as the lateral corticospinal spinal tract in the spinal cord (Fig. 2). The other major descending motor system is the corticobulbar tract, which originates in the cortical motor areas described above and descends alongside the corticospinal tracts before eventually projecting to brainstem motor cranial nerve nuclei bilaterally. The exception to this rule is innervation to the lower face, which is unilateral.
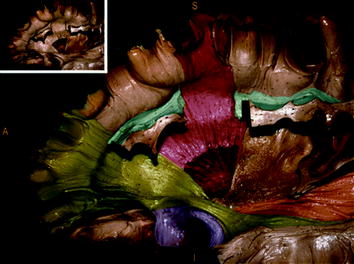
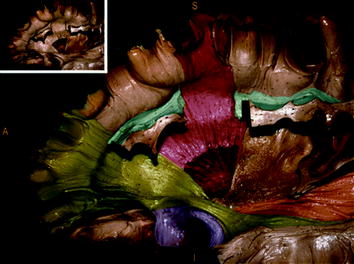
Fig. 2
Major white matter pathways. Dissected left hemisphere demonstrating main white matter pathways. Inferior frontal occipital fasciculus (IFOF, green); uncinated fasciculus (UF, violet); superior longitudinal fasciculus (SLF, blue); corticospinal tract (CST, orange). Inset shows colors prior to colorization. Adapted from De Benedictis et al. [42]
2.2 Sensory
The primary sensory pathway of relevance to glioma surgery is the posterior column–medial lemniscus pathway. Vibration, proprioceptive, and light touch sensory information from the periphery is detected by sensory neurons that have their cell body in the dorsal root ganglion and axon that ascends within the posterior column of the spinal cord and synapse onto ipsilateral gracile and cuneatus nuclei that are devoted to lower body and upper body sensation, respectively, within the caudal medulla. Second-order axons then cross the midline and ascend within the medial lemniscus within the contralateral brainstem and midbrain before eventually synapsing onto neurons of the ventral posterior lateral (VPL) nucleus of the thalamus. Neurons of the VPL then project to the layers III and IV of S1 [19]. S1 is within the postcentral gyrus of the parietal lobe, and electrical stimulation of S1 causes sensory perception, typically described as tingling (Fig. 1), in a localized region of the contralateral body, as initially described by Cushing in 1909 [20].
2.3 Language
2.3.1 Hemispheric Dominance
The concept of left hemispheric dominance for language was first proposed by Paul Broca in 1865 [21]. Current data are that for 85 % of the population the left hemisphere is dominant, while 9 % of patients have bilateral representation, and 6 % have right-sided dominance. For right-handed patients, 98 % have left-sided dominance. Thus, clinical investigation of dominance, the gold standard being the Wada test, is typically reserved for left-handed or ambidextrous patients. In addition to language, the left hemisphere is typically involved in logical problem solving and calculation. Conversely, the right hemisphere is specifically devoted to facial recognition, tasks involving visuospatial manipulation, and musicality [22].
2.3.2 Comprehension
Language comprehension was described by Wernicke as residing in the posterior superior temporal gyrus [23]. DCS studies have further expanded the region of language comprehension to include the posterior portion of both the superior and middle temporal gyri, as well as the inferior parietal lobule superior to the Sylvian fissure. Damage to any of these areas can result in a receptive aphasia, in which the patient can still produce written or oral language with normal grammar/syntax/prosody, but the word content is incorrect, often with neologisms or word salad. In addition, the ability to repeat words and name pictures is compromised, although naming and language comprehension may be mediated by distinct regions of the posterior superior temporal lobe [24]. In addition, the ability to sing and to recite memorized passages is maintained. If similar areas are damaged in the nondominant hemisphere, dysprosody may occur, which is the inability to detect the pitch, rhythm, or emotional content of speech. More recent studies have aimed to further dissect various aspects of language within the parietal-temporal-occipital junction. From these studies, we have learned that the posterior temporal lobe is involved in reading and word retrieval but not particularly involved in word repetition.
2.3.3 Language Output
Classically, the final common pathway of language output is known as Broca’s area, which encompasses the pars triangularis and pars opercularis within the posterior third of the inferior frontal gyrus. However, recent studies point to the ventral dPMC as the final common speech output region, while Broca’s area may be more involved in higher order speech processing. Thus, stimulation of vPMC typically causes overt speech arrest, while stimulation at Broca’s area causes dysnomias. Interestingly, the inferior frontal gyrus of the nondominant hemisphere appears to be involved in the speech prosody (rhythm/stress/intonation of speech), with lesions to this area causing flat, unemotional speech [25]. In addition, as mentioned earlier in the chapter, the SMA of the dominant hemisphere is involved in speech initiation, and stimulation can cause temporary speech arrest or vocalization. Finally, the dominant insular cortex may plan an important role in speech planning [26].
Recent insights from cortical stimulation studies have refined our understanding of the relationship of writing. While circuits involved in writing do correspond to the same hemisphere as oral language, at least some of the writing sites are partially distinct, as evidenced by the presence of writing deficits despite negative mapping at traditional language sites. Areas shown to be important for writing function include the dominant hemisphere superior parietal lobe, supramarginal gyrus, insula, middle and inferior frontal gyri, and SMA [27].
2.3.4 Subcortical Language Representation
The major subcortical pathways subserving oral language function as identified by direct intraoperative stimulation are the superior longitudinal fasciculus (SLF) and inferior occipito-frontal fasciculus (Fig. 2). The SLF connects the parietal/temporal region with the frontal lobe and is composed of two functionally distinct white matter pathways—the arcuate fasciculus (AF) and an indirect pathway parallel and lateral to the AF termed the lateral SLF (latSLF). The AF (also termed the dorsal phonologic stream) connects the posterior middle and inferior frontal gyri with the posterior portion of the middle and inferior temporal gyri, with interruption at any site along the pathway resulting in phonological disturbances. The latSLF, which connects the posterior superior temporal gyrus, supramarginal gyrus, and primarily vPMC, is involved in speech perception and articulation. The inferior frontal occipital fasciculus, also termed the ventral semantic stream, is involved with semantic aspects of speech and stimulation of the pathway intraoperatively produces semantic paraphasias. Finally, the subcallosal fasciculus, connecting the mesial frontal lobe structures (SMA, cingulate) to the caudate nucleus may mediate the control of language, with lesions resulting in transcortical motor aphasias characterized by nonfluent aphasia with intact repetition.
A number of studies have investigated the representation of languages in multilingual patients. A consistent finding is that both shared and language-specific sites are present in bilingual patients. While primary language and shared language sites are found throughout the temporal, parietal, and frontal lobes, distinct secondary language sites are located in the posterior temporal and parietal regions [28]. Interestingly, a recent study using DCS in bilingual patients implicate the dominant posterior temporal area and SLF in mediating language switching [29].
2.4 Vision
Visual information enters the retina and is transmitted via axons of retinal ganglion cells in the optic nerves, which cross at the optic chiasm and continue as the optic tracts which synapse in the lateral geniculate nucleus (LGN) of the thalamus. Cells of the LGN then project to the primary visual cortex of the occipital lobe via a fan-like projection of fibers (optic radiations) that pass lateral and superior to the atria and temporal horn of the lateral ventricle. The inferior optic radiations (Meyer’s loop) project through the temporal lobe, carrying visual information from the contralateral superior visual field, with lesions causing a “pie in the sky” contralateral homonymous superior quadrantanopsia, though the ipsilateral eye is typically affected to a greater extent because of the lateral position of ipsilateral relative to contralateral eye fibers in the optic radiations [19]. Superior optic radiations project through the inferior parietal lobe and carry visual information from the contralateral inferior quadrants. The primary visual cortex resides in the occipital lobe along both borders of the calcarine sulcus and is retinotopically organized.
2.5 Spatial Cognition
The nondominant parietal lobe has been implicated in visuospatial perception, with damage to this region in humans producing a clinical syndrome of unilateral neglect, where patients essentially ignore the left half of their visual field. Recent studies using a line bisection task in awake human patients undergoing DCS has further defined the regions responsible for spatial cognition as both the supramarginal gyrus and posterior superior temporal lobe of the nondominant hemisphere [30]. Subcortically, both the optic radiations and nondominant SLF are involved in visuospatial information transmission.
3 Tumor Location and Extraoperative Assessment of Functional Regions
3.1 Tumor Imaging
Preoperative image of patients harboring gliomas begin with standard MRI sequences, most importantly T1-weighted images with gadolinium and T2/FLAIR. In addition to providing anatomic localization of the tumor, the presence of enhancing tumor presents a target for surgical resection, as tumor recurrence risk is greatest within 2 cm of the enhancing rim [31]. Also, enhancement generally indicates the presence of a high-grade tumor, although there are exceptions. FLAIR sequences demonstrate the extent of edema, although one cannot determine the relative contributions of tumor versus vasogenic edema. With that caveat, for the case of low-grade gliomas (LGG), FLAIR signal is generally considered the target of resection. MR spectroscopy may be a useful adjunct in the setting of previously treated patients for which the diagnosis of recurrence versus treatment effect is equivocal.
3.2 Identifying Functional Brain Regions
3.2.1 Anatomic Localization of Functional Regions with MRI and DTI
In order to determine the relationship of the tumor to the Rolandic (sensorimotor) cortices, it is imperative that the surgeon be able to localize the central sulcus from preoperative MRI. Multiple methods exist for identifying the central sulcus (CS). On high-vertex axial images, mirror-image transverse sulci that are nearly perpendicular to the midline represent the CS (Fig. 3). The “hand knob”, an omega-shaped region of the precentral gyrus, can usually be seen anterior to the CS. Another method is to analyze a sagittal MRI image at the midline. The cingulate sulcus is identified and followed posteriorly and then superiorly where it terminates as the marginal sulcus. Immediately anterior to the marginal sulcus is the paracentral lobule, which is a medial extension of both the precentral (motor) and postcentral gyri of the lateral hemisphere. A third method utilizes a sagittal MRI image through the superficial portion of the inferior frontal gyrus, where pars triangularis and pars opercularis are identified. The vPMC is located just posterior to the precentral sulcus, and just posterior to vPMC is the inferior portion of the postcentral gyrus.
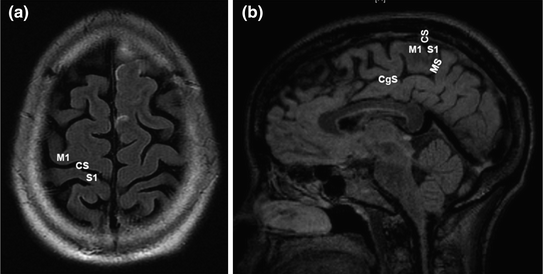
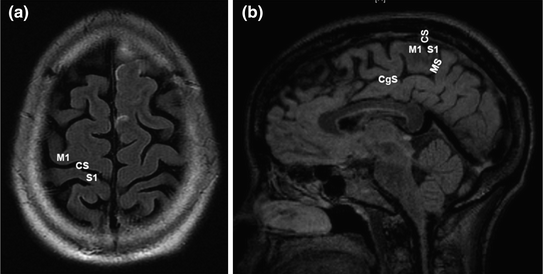
Fig. 3
Primary motor and sensory cortex landmarks on MRI. a Axial plane cut near the vertex illustrating hand knob within primary motor area (M1) of precentral gyrus and primary sensory area (S1) of postcentral gyrus anterior and posterior to central sulcus (CS), respectively. b Sagittal MRI slice just off midline showing paracentral lobule containing M1, S1, and CS. In this view, the cingulate sulcus (CgS) at its posterior extent angles superiorly to form the marginal sulcus (MS), which is continuous with the postcentral sulcus that forms the posterior border of S1
In addition to these topographic assessments of sensorimotor function, diffusion tensor imaging (DTI) is emerging as a routine part of preoperative evaluation of brain tumor patients as well as an important intraoperative adjunct to DCS. DTI fundamentally measures the degree of water diffusion within brain compartments, with more anisotropic regions such as white matter tracts having restricted directionality. The diffusion tensor can be evaluated for each MRI voxel of interest and a three-dimensional map is generated which accurately displays major white matter bundles, such as the CST, AF, SLF, IFOF, ILF, optic radiations, corpus callosum, and cingulum. While the process is associated with some inherent error and must be verified with direct stimulation in the operating room, in our experience DTI is helpful in determining the mapping strategy for a given tumor, discussing expectations with patients, and improving the efficiency of mapping in the operative room. As a general rule, DTI maps tend to be larger than that observed by direct stimulation, suggesting that resection of a significant part of a DTI-defined tract can be well tolerated in terms of maintaining function and highlighting the importance of direct stimulation during resection.
In contrast to preoperative localization of cortical and subcortical motor function, for which the standard MRI, DTI, and fMRI findings are relatively consistent between patients, functional language circuits are more diffuse and variable, making anatomic localization preoperatively more difficult. One particularly promising strategy is the use of preoperative DTI to localize language circuits. Recent data from a series of 230 patients undergoing glioma resection for whom the concordance of preoperative DTI with direct subcortical stimulation during surgery demonstrated a high concordance rate for the CST (motor), SLF (phonemic language), and frontal occipital fasciculus (semantic language) [32]. Thus, DTI is becoming an important preoperative and intraoperative adjunct to direct cortical mapping.
3.2.2 Functional Magnetic Resonance Imaging (fMRI)
fMRI is based on the principle that neurons of more active brain regions receive increased blood flow, which results in a localized increase of oxyhemoglobin (diamagnetic) relative to deoxyhemoglobin (paramagnetic). While localization of primary motor and sensory cortices by fMRI is accurate, several issues plague its widespread clinical use in brain tumor patients. First, at its most basic level, fMRI only demonstrates areas of increased blood flow, which is an indirect measure of neuronal activity. Also, the time lag between neuronal firing and diverted blood flow may be problematic for interpretation, particularly for complex actions involving several brain regions or which have overlapping neural circuitry, such as language or higher order cognitive function. fMRI at best illustrates which brain regions are involved in a particular task, but it does not indicate which regions are functionally necessary.
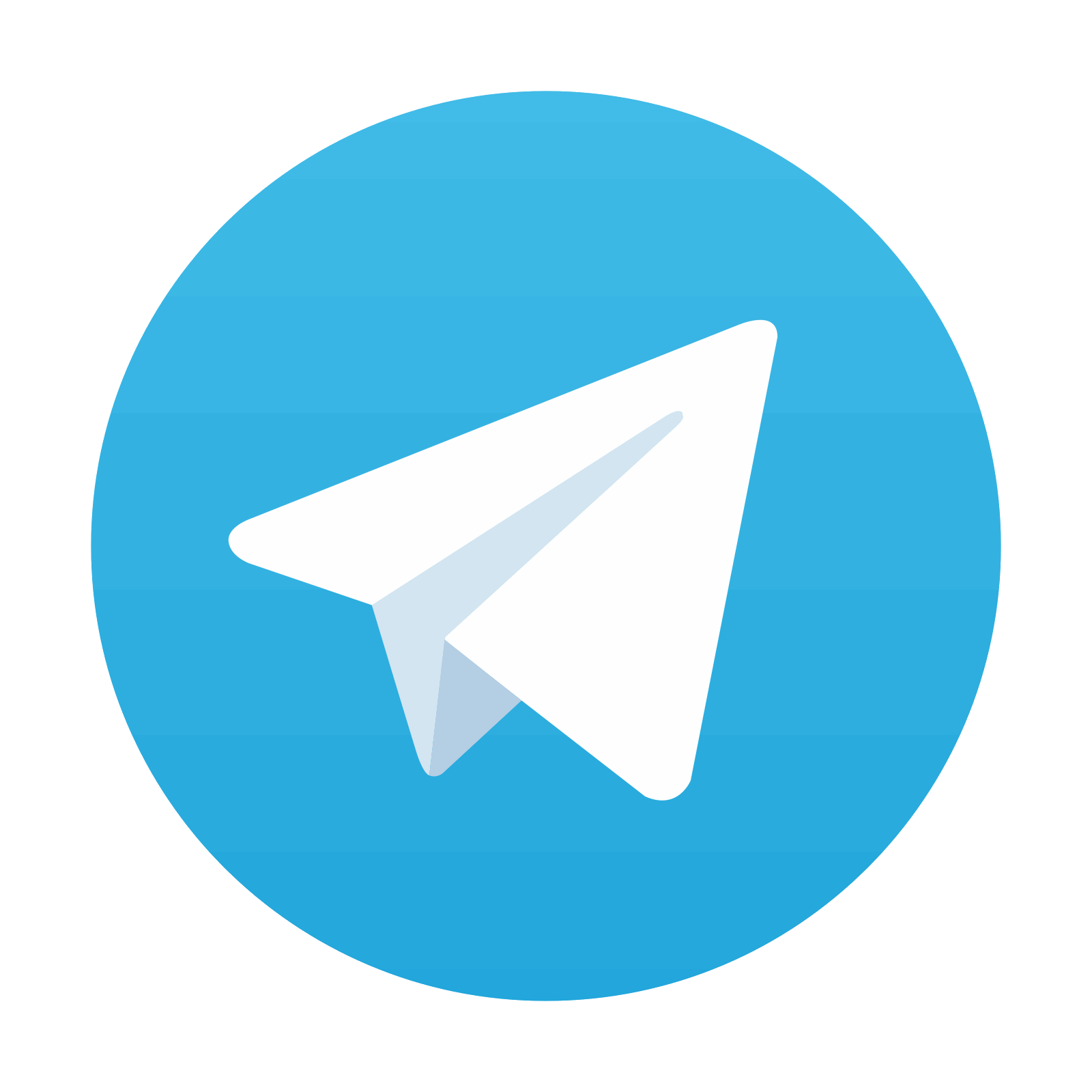
Stay updated, free articles. Join our Telegram channel

Full access? Get Clinical Tree
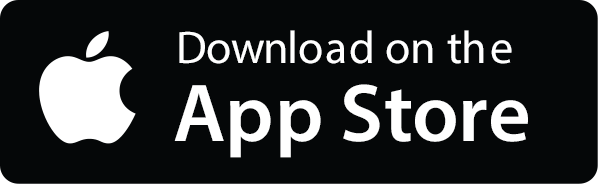
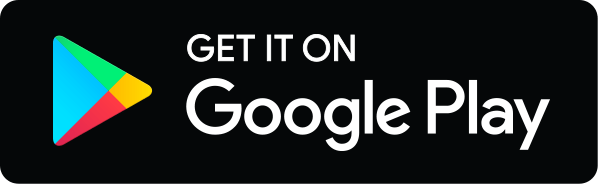