Fig. 2.1
The structures of different follicles and its development are shown in a clockwise direction proceeding from the primordial follicle to pre-ovulatory follicle as well from ovulating to corpus luteum formation. (1) The primordial follicle contains the oocyte surrounded by flat, squamous granulosa cells that are segregated from the oocyte’s environment by the basal lamina. (2) The primary follicle begins with the change of granulosa cells from a flat to a cuboidal structure, and the oocyte genome is activated and genes become transcribed. In this stage, a glycoprotein polymer capsule, the zona pellucida, is formed around the oocyte, separating it from the surrounding granulosa cells. (3) The secondary follicle is surrounded by the outermost layer, the basal lamina, and undergoes cytodifferentiation to become the theca externa and theca interna. An intricate network of capillary vessels forms between these two thecal layers and begins to circulate blood to and from the follicle. (4) The tertiary follicle is the basic structure of antral follicle. Granulosa and theca cells continue to undergo mitosis concomitant with an increase in antrum volume. (5) The ovulating follicle is excreting the oocyte with a complement of cumulus cells by the surge of LH during menstrual cycle. (6) The corpus luteum is formed from the ruptured follicle, and a steroidogenic cluster of cells that maintains the endometrium of the uterus by the secretion of large amounts of progesterone. The figure was downloaded from the Web site. http://cnx.org/content/col11496/1.6/
FSH is not required for this transition as primordial follicles do not express FSH receptors [17]. Resting follicles are likely to be under constant inhibitory influences of systemic or local origins to remain dormant [6]. A decrease of inhibitory influences or an increase of stimulatory factors allows the initiation of follicle growth. Anti-mullerian hormone (AMH) is involved in the control of primordial follicle activation by inhibiting the recruitment of primordial follicles into the growing pools. In adult females, AMH is produced by the granulosa cells of growing follicles, its expression decreasing in large antral follicles, and serum AMH is a useful biomarker of the ovarian reserve of growing follicles in human [18]. Recent studies on genetically modified mice have revealed that there are indeed some inhibitory signals that maintain primordial follicles in the dormant state. Loss of function of any of the inhibitory molecules for follicular activation, including Tsc-1, Pten, Foxo3a, and Foxl2, leads to premature activation of the primordial follicle pool [19–21]. With aging, as follicles continuously leave the resting pool, the number of growing follicles decreases, but the proportion of primary and early growing follicles increases in primates [22] as in mice [23]. This increase may be triggered by the progressive disappearance of an inhibitory influence on primary follicle [6].
In addition to inhibitory signals that inhibit premature activation of primordial follicles, there are some other signals in the ovary that promote the transition of primordial follicles to primary follicles. With synergistic actions of these signals, growth is initiated in primordial follicles [24]. According to studies on transgenic animal models and on the human ovary, several members of the TGF-β super family, such as BMP-4, BMP-7 [25, 26], and GDF-9, play critical roles in this process. Other growth factors and cytokines also act at the formation of primary follicles, such as kit-ligand, leukemia inhibitory factor (LIF) [27], basic fibroblast growth factor (bFGF) [28], and BMP-15 [29].
Several transcription factors that might regulate this early step of folliculogenesis have been identified, illuminating key signaling pathways responsible for the maintenance at the resting stage or the recruitment of primordial follicles. Recently, four main transcription factors have been identified by using mutant mice: Nobox (newborn ovary homeobox), Sohlh-1 and Sohlh-2 (spermatogenesis and oogenesis helix–loop–helix 1), and Lhx8 [30–32]. The phenotype of the four gene mutants is very similar [31], as a failure in the primordial to primary follicle transition. Further studies are needed to reveal potential inhibitory factors or intra-ovarian stimulating factors that are involved in the initial stage of follicle recruitment.
Pre-antral Follicle Growth and Differentiation
Pre-antral follicular development includes the primary to secondary follicle transition and the development of secondary follicles to the per-antral stage. When follicles leave the resting pool, the granulosa cells become cuboidal and begin to express markers of cell proliferation. The transition from the primordial to primary stage can be very prolonged. When primary follicles enter the growth phase their size increases, both by enlargement of the oocyte and proliferation of granulosa cells, single-layered primary follicles are transformed into multilayered secondary follicles. As they enlarge, the surrounding layer of stroma cells stratifies and differentiates in two parts: the outer part is the theca externa and the inner part is the theca interna [33]. The oocyte continues to grow, the zona pellucida is formed, theca condenses around the pre-antral follicle, and the vascular supply develops [29]. The zona pellucida consisted by a glycoprotein polymer capsule around the oocyte, separating it from the surrounding granulosa cells. The zona pellucida, which remains with the oocyte after ovulation, contains enzymes that catalyze with sperm to allow penetration. From a follicular diameter of about 0.15 mm in humans [34], the theca interna commences its epithelioid differentiation and the follicle is defined as a secondary follicle and constitutes the first category of growing follicles in a classification based on morphological aspect and total number of granulosa in each individual follicle [34]. Stroma-like theca cells are recruited by oocyte-secreted signals. They surround the follicle’s outermost layer, the basal lamina, and undergo cytodifferentiation to become the theca externa and theca interna. An intricate network of capillary vessels forms between these two thecal layers and begins to circulate blood to and from the follicle. The secondary follicle is marked histologically by a fully grown oocyte surrounded by a zona pellucida, approximately nine layers of granulosa cells, a basal lamina, a theca interna, a capillary net, and a theca externa.
The response of follicles to gonadotropins depends on the number of receptors for gonadotropins present on follicle cells and the transducing mechanisms to which these receptors couple. Available data in humans indicate that the number of FSH receptors on granulosa cells seem to be unchanged [10]. During this phase, follicles less than 2 mm exhibit a slight steroidogenic activity, the progressively increased mitotic activity of granulosa cells is not mediated by the actions of gonadotropins [35]. FSH may have a permissive role rather than being essential in pre-antral follicle growth [36]. In contrast to the debatable role of FSH in pre-antral follicle growth, there is firm evidence that certain members of the TGF-β super family locally produced from follicles [33, 37, 38], theca cells (BMP-4 and BMP-7) or both (TGF-β), or oocytes (GDF-9 and BMP-15), play crucial roles in the growth of primary follicles into pre-antral and antral stages [25, 39, 40]. Because EGF/TGF-α and their receptors, as well as IGF-R, have been detected in pre-antral and small antral human follicles, it is likely that these factors may also play a positive role in sustaining growth of small follicles [6].
BMP-4 and BMP-7 modulate FSH signaling in a way that promotes estradiol production while inhibiting progesterone synthesis, acting as a luteinization inhibitor [41]. In vitro exposure of ovarian cortical samples to oocyte-derived recombinant GDF-9 has been shown to increase the number of primary and secondary follicles in human and rodents suggesting an important role, at least under in vitro conditions, for this growth factor in the initiation and progression of follicle growth [27, 42]. Another research has found that BMP-15 can stimulate granulosa cell mitosis in pre-antral follicles during the FSH-independent stages. BMP-15 can also inhibit FSH receptor expression [38].
From Antral to Pre-ovulatory Follicles
In humans, follicles pass from pre-antral to early antral stage at a follicular diameter comprised between 0.18 and 0.25 mm. It is also during this stage that the follicle begins to exhibit some fluid-filled spaces within the granulosa cell layers, which will coalesce to form the antral cavity, along with increased vascularization of the theca layer, continued growth of oocytes and proliferation of granulosa and theca cells [24]. Stroma-like theca cells are recruited by oocyte-secreted signals. They surround the follicle’s outermost layer, the basal lamina, and undergo cytodifferentiation to become the theca externa and theca interna. An intricate network of capillary vessels forms between these two thecal layers and begins to circulate blood to and from the follicle.
The time required by a follicle to grow from the pre-antral stage to a size of 2-mm antral follicle is of about 70 days [2], and this part of folliculogenesis is named basal follicular growth. The formation of a fluid-filled cavity adjacent to the oocyte called the antrum designates the follicle as an antral follicle, in contrast to a so-called pre-antral follicle that still lacks an antrum. An antral follicle is also called a Graafian follicle. Once entering the growing pool, most growing follicles progress to the antral stage, at which point they inevitably undergo atresia. After pubertal onset, a small number of the antral follicles can be rescued by gonadotropins to continue growth, and normally only one antral follicle is further developed each month in preparation for ovulation. Antral follicles (2–5 mm diameter) develop into pre-ovulatory follicles (16–29 mm diameter) in 14 days during the follicular phase of the menstrual cycle (Fig. 2.2).
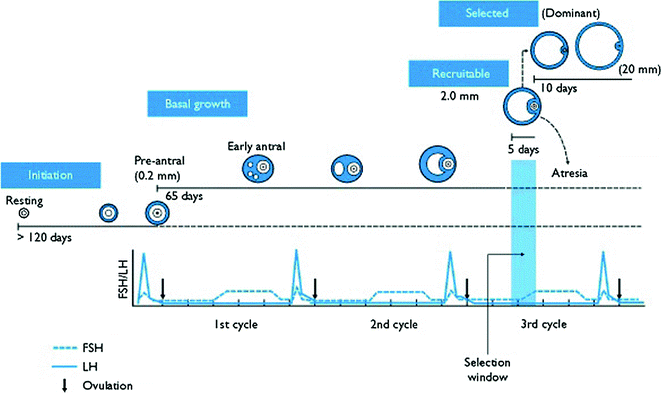
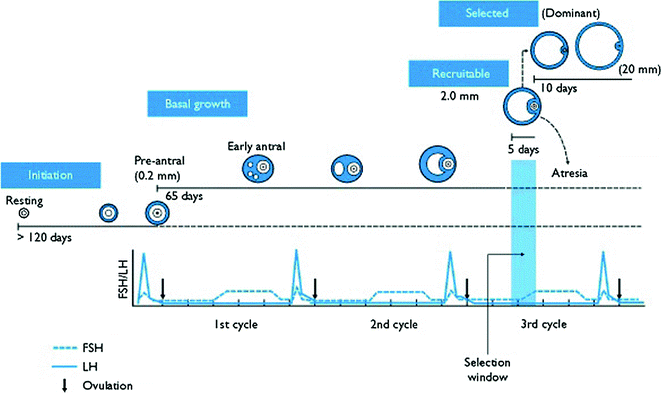
Fig. 2.2
Diagram for folliculogenesis. The development of a primordial follicle to a pre-ovulatory follicle takes in excess of 120 days. After it has become a pre-antral follicle of about 0.2 mm diameter, it takes about 65 days to develop into a pre-ovulatory follicle. Cohorts of follicles continually develop but only one is ‘selected’ and becomes the dominant follicle. All others undergo atresia. The figure was downloaded from the Web site. http://www.ncbi.nlm.nih.gov/books/bv.fcgi?rid=endocrin.box.1226/
Antral Follicle Recruitment
Follicles in diameter of about 2–5 mm are present throughout the menstrual cycle. During the late luteal phase, the 2–5 mm follicles, which have entered the pre-antral stage 70 days earlier, become selectable follicles for further development [34]. Recruitment of the follicular cohort occurs in response to a transient elevation in circulating FSH. After increases in circulating FSH during the peri-menstrual period, a cohort of antral follicles escapes apoptosis due to the survival action of FSH (Fig. 2.2). In this period, the granulosa and theca cells continue to undergo mitosis concomitant with an increase in antrum volume, and those follicles are defined as tertiary follicles. In the tertiary follicle, the basic structure of the antral follicle has formed and no novel cells are detectable. Tertiary follicles can attain a tremendous size that is hampered only by the availability of FSH, which it is now dependent on.
Under action of an oocyte-secreted morphogenic gradient, the granulosa cells of the tertiary follicle undergo differentiation into four distinct subtypes: corona radiata, surrounding the zona pellucida; membrana, interior to the basal lamina; peri-antral, adjacent to the antrum; and cumulus oophorus, which connects the membrana and corona radiate granulosa cells together (Fig. 2.3). Each type of the cells behaves differently in response to FSH. Theca cells express receptors for luteinizing hormone (LH). LH induces the production of androgens by the theca cells, most notably androstenedione, which are aromatized by granulosa cells to produce estrogens, primarily estradiol. Consequently, estrogen levels begin to rise.
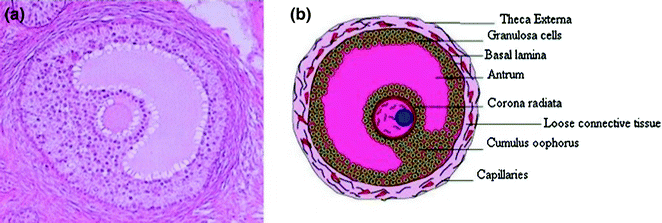
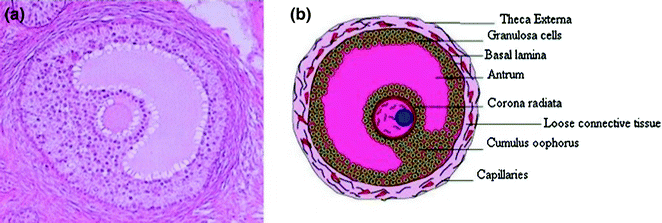
Fig. 2.3
The structure of human antral follicle. The figures were downloaded from Web site: a http://resources.ama.uk.com/glowm_www/uploads/1211558633_graafian_follicle.jpg; b http://biology4isc.weebly.com/1-human-reproduction.html
Although traditional thinking proposes a single wave of cyclic follicular recruitment and growth, recently it has been suggested that multiple waves of follicle development may occur in the human ovary [43]. The selectable follicles become more responsive to gonadotropins, but their FSH-induced aromatase remains poorly expressed [33]. Available data in humans indicate that the number of FSH receptors does not change during antral development, at least until 12 mm [44]. This does not signify that responsiveness of granulosa cells to FSH is unchanged, since mechanisms of autocrine/paracrine changes may occur [45]. Once the follicles reach antral and larger sizes, multiple intra-follicular factors are produced locally to ensure successful maturation and ovulation, such as factors produced from granulosa cells (activins, BMP-6), theca cells (BMP-2, BMP-4 and BMP-7) or both (TGF-β), or oocytes (GDF-9 and BMP-15). Those factors act alone or synergistically in human selectable follicles, having the potential to increase the FSH, and induce the proliferation of granulosa cells when FSH levels increase. The increasing follicular vascularization may also increasing the blood flow and then increase the amount of FSH reaching the follicle, all above mechanisms may enhance granulosa cell proliferation [46].
Dominant Follicle Selection
Follicle selection is the process by which a single dominant follicle is chosen from the recruited cohort for preferential growth [34, 47, 48]. At the time of selection, the dominant follicle continues to grow while the subordinate follicles undergo atresia [47, 49]. Divergence occurs when the dominant follicle reaches a diameter of about 10 mm on day 6–9 of the follicular phase in women [44, 47, 50, 51].
There is evidence in women that the dominant follicle has an early size advantage and the lowest FSH ‘threshold’ over subordinate follicles [52, 53]. Using the bovine model, it is now clear that each follicular wave is necessarily preceded by a surge in circulating FSH [54]. It has been suggested that the future dominant follicle may contain more granulosa cells and FSH receptors, making it more sensitive to FSH, compared with the subordinate follicles [55]. The increased responsiveness of dominant follicles to FSH stimulates the expression of both FSH and LH receptors in the granulosa cells of this follicle [56, 57]. The follicle destined to become dominant then has more LH receptors and the ability to respond to LH imbues the follicle with the ability to survive without FSH [54]. Additionally, this rapidly growing follicle also produces higher levels of autocrine/paracrine growth factors. Multiple studies have demonstrated the importance of insulin-like growth factors (IGFs), TGF-β super family, and other local factors in the amplification of FSH action [40, 47], which constituting a local positive selection mechanism [58, 59]. It is proposed that differential exposure to these signaling molecules may be one of the ways in which the dominant follicle is sensitized to FSH and thereby selecting for preferential growth. Another mechanism recently has been proposed for dominant follicle selection is the possible differential regulation of blood vessel formation and permeability in the theca layers of cohort follicles [60, 61].
The dominant follicle may also suppress the growth of the subordinates in the existing wave and suppresses the emergence of the next follicular wave [54]. The follicle destined for ovulation grows faster than the rest of the cohort and changes from an androgen- to an estrogen-producing structure by expressing its FSH-induced aromatase activity. Estrogens and inhibins produced by this follicle suppress pituitary FSH released during the mid-follicular phase [6]. Negative selection against subordinate follicles is a result of estrogen and inhibins, the remaining growing antral follicles are deprived of adequate FSH stimulation required for survival [62]. Subordinate follicles are not able to thrive in an environment of declining FSH and undergo atresia [11, 51, 63]. So the process of selection has been described as a phenomenon of avoiding atresia [64, 65]. Those follicles are defined as atretic follicles. Nevertheless, it does not mean that the subordinate follicles are started atresia immediately even though they were not selected to become the dominant follicle in the same wave of cohort follicular pool. The surge of LH during the menstrual cycle may trigger the atresia of those atretic follicles in the same wave of follicular pool, but it seems that the oocytes contained in those atretic follicles do not lose their developmental competence at once. However, the mechanism of atresia for those atretic follicles needs to be further confirmed.
Pre-ovulatory Follicle Development
The dominant follicle continues to develop after it is selected and reaches pre-ovulatory status at a diameter of 16–29 mm in the late-follicular phase [34, 66, 67]. In parallel with its increasing size, high proliferative activity of granulosa cells in the pre-ovulatory follicle also undergoes marked changes in steroidogenic activity [6].
The preferential growth of the dominant follicle is associated with increased aromatase activity and a rapid elevation of circulating and follicular fluid estradiol-17β [8, 43]. The process of folliculogenesis indicates that follicular responsiveness to gonadotropins increases progressively as the follicle develops from the pre-antral to pre-ovulatory stage [6]. Greater gonadotropin responsiveness in the dominant follicle, compared with subordinate follicles, is responsible for mediating dominant follicle granulosa cell estradiol production, LH receptor expression, and continued pre-ovulatory growth [68, 69]. The dominant follicle is responsible for over 90% of the estrogen production in the pre-ovulatory period [64]. When folliculogenesis is completed, just before ovulation, the granulosa cells are highly differentiated in the pre-ovulatory follicle, having stopped to proliferate but producing high levels of steroids [6, 70].
Estradiol production from the dominant follicle peaks the day before the LH surge [2, 71] providing positive feedback at the hypothalamus and pituitary to stimulate the surge of LH necessary for inducing ovulation. The highly vascularized pre-ovulatory follicle, which has acquired LH receptors, is able to respond to the mid-cycle rise in LH [2]. Ovulation occurs, on average, within 24 h of the LH peak [62, 72]. Serum progesterone concentrations begin to rise after the pre-ovulatory estradiol peak but before the LH surge, and indicate the onset of follicular luteinization [73, 74].
Ovarian Follicular Wave Dynamics
Antral follicles 2–5 mm in diameter have been detected histologically and ultrasonographically throughout the human menstrual cycle [43]. The pattern of emergence of 2–5 mm follicles is a matter of long-standing debate. Some investigators have suggested that antral follicles 2–5 mm develop continuously, while others have proposed that ‘cohorts’ or ‘waves’ of antral follicles develop in a cyclic manner during the menstrual cycle [75]. A wave of follicular development has been defined as the synchronous growth of a group of antral follicles at regular intervals during the ovarian cycle. The traditional theory of human folliculogenesis holds that a single cohort of 4–14 antral follicles is recruited to grow in each ovary during the late luteal phase of the human menstrual cycle [1] and selection of one dominant follicle from this cohort for preferential growth in the early- to mid-follicular phase.
However, more recent results document that antral follicular growth may start in different phases of the menstrual cycle due to the balance of endocrine and intra-ovarian regulators, and selection of a dominant follicle can occur in anovulatory waves before the ovulatory follicle in women [44]. More research involving daily transvaginal ultrasonography and concurrent endocrine profiling has documented a wave pattern of antral follicle development during the menstrual cycle in women. A research has found that a cohort of 4–14 follicles 2–5 mm was recruited either two or three times during the inter-ovulatory interval in a study of 50 healthy women [8, 43]. The causes and consequences of two- or three-wave patterns are not understood clearly, but some researches found the correlate to the number of waves in an inter-ovulatory interval was the duration of follicular dominance of the first follicular wave after ovulation. Therefore, factors that influence the development of the dominant follicle of the first wave may be responsible for regulating the wave pattern. So far, it has not been established whether follicular wave dynamics are consistent within individual woman, are related with fertility, or change with age. The understanding of human ovarian folliculogenesis may have profound implications in ART and fertility preservation [76].
Follicle Atresia
In the humans, atresia causes the elimination of >90% of follicles entering the growth phase [6, 34, 77]. The phenomenon of atresia affects follicles at all stages of their development, may be considered as a normal process, which is a significant factor in determining the precise number of follicles that will ovulate in each cycle [34, 78]. Once a cohort of follicles is recruited to grow, they are destined to undergo apoptosis at the early antral stage unless rescued by survival factors. The selected follicles mature and ovulate in response to the pre-ovulatory gonadotropin surge. Following repeated cycles of recruitment, atresia, or ovulation, the follicle reserve is exhausted [79].
Changes of Follicular Morphology and Metabolism During Atresia
The healthy follicles and atretic follicles have difference between morphological criteria and follicular metabolism. Early changes of atretic follicles are irregular shape of the follicle and of the oocyte and nuclear pyknosis in the granulosa cell layers [80, 81]. The pyknosis of granulosa cells is an apoptotic process [33, 82, 83]. The first morphological evidence of apoptosis is condensation of nuclear chromatin into crescentic caps at the periphery of the nucleus, at the same time, cytoplasmic condensation results in a reduction of total cell volume and a related increase in cell density [6].
It is generally assumed that atretic follicles possess an intra-follicular androgenic milieu that distinguishes them from healthy follicles [2], and aromatase activity is poorly expressed [72]. On the contrary, the healthy follicles larger than 8 mm differ strongly from atretic follicles of similar size, and they possess aromatase. Other alterations in follicular metabolism including the appearance of lipid droplets, 3β-HSD, glucose-6-phosphate dehydrogenase, acid phosphatase, and aminopeptidase, as well as a profound decrease in the levels of lactate denydrogenase in follicular fluid of human atretic follicle [6].
Hormonal Regulation of Follicle Atresia
Ovarian follicular atresia is a hormonally controlled apoptotic process. Atresia occurs at all stages of follicle development, although follicle growth continues in the absence of circulating FSH during the pre-antral stage, and FSH is necessary for the development of follicle antrum [84, 85], because sufficient exposure of antral follicles to FSH is the most critical stimulus for the follicles to escape atresia and reach the pre-ovulatory follicle stage. During the menstrual cycle, circulating levels of FSH exhibit important variations: FSH is highest during the first half of the follicular phase and lowest during the mid-luteal phase, and during this last phase, selectable follicles exhibit their highest rate of atresia. In the absence of FSH, steroids, and growth factors [77, 84], follicles undergo atresia [72, 83, 86]. As mentioned above, the surge of LH during the menstrual cycle may trigger the rest of tertiary follicles for atresia. However, it does not mean that the oocytes obtained from the follicles during the mid-luteal phase have lower developmental competence. Another word, atresia is a long process, and the atresia of selectable follicles in the ovary may need several menstrual cycles to be completed.
Importantly, follicles can be rescued at early phases of atresia by exogenous gonadotropins [87, 88]. Gonadotropins are the major survival factors that suppress granulosa cell apoptosis through the activation of the cAMP-dependent pathway, which verifies that the signaling is via the protein kinase A pathway [89]. The apoptosis-suppressing action of gonadotropins is augmented by local factors including interleukin-1/nitric oxide, estrogens, and insulin-like growth factor-1, which in turn prevent apoptosis by activating the cGMP-dependent pathway, nuclear estrogen receptor, and tyrosine phosphorylation, respectively [79]. Another pituitary hormone, growth hormone (GH), also affects follicular growth and differentiation and often augments the action of gonadotropins [90, 91]. When tested in the follicle culture system, GH also suppresses the spontaneous onset of apoptosis [91].
Molecular Mechanisms of Follicle Cell Apoptosis
The initiation phase of apoptosis within the granulosa cells can be promoted by extrinsic factors such as cytokines [e.g., tumor necrosis factor, Fas ligand and tumor necrosis factor-related apoptosis inducing ligand (TRAIL)] or the withdrawal of growth factors and is often mediated by membrane death receptors (e.g., tumor necrosis receptor family) [92–94]. Alternatively, cell death can also be induced by intrinsic factors including oxidative stress or activation of tumor suppressor gene (e.g., p53) [11, 95].
Oxidative stress may induce the granulosa cell apoptosis via alteration of the cellular ionic environment, which activates a Ca2+/Mg2+ -sensitive endonuclease resulting in activation of the apoptotic cascade [96, 97]. In addition, DNA damage, such as that initiated by oxidative free radicals, may be a primary stimulus for increased p53 expression in the granulosa cells [98]. It has been suggested that p53 has the potential to amplify the negative effect of oxidative free radicals on granulosa viability. On the contrary, the apoptosis-suppressing action of gonadotropins is augmented by local factors including interleukin-1/nitric oxide, estrogens, and IGF-1, which in turn prevent apoptosis by activating the cGMP-dependent pathway, nuclear estrogen receptor, and tyrosine phosphorylation, respectively.
Although the exact signals, receptors, and intracellular signaling pathways leading to apoptosis within the granulosa cells are not understood completely, it is likely that multiple molecules include both survival (such as gonadotropins, insulin-like growth factor-1, interleukin-1, epidermal growth factor, basic fibroblast growth factor, TGF-α, bcl-2, and bcl-xlong) and atretogenic factors (TGF-β, interleukin-6, androgens, reactive oxygen species, bax, Fas antigens, p53, TNF, and caspases) are involved [82, 98–100], and these diverse hormonal signals probably converge on selective intracellular pathways (including genes of the bcl-2 and ICE families) to regulate apoptosis, the outcome depends upon a delicate balance between these molecules [79].
Oocyte Growth
Size of Oocytes and Follicles
During folliculogenesis, human oocyte grows from 35 to 120 μm in diameter [6]. Oocyte growth is interdependent with the development and differentiation of the follicles [101]. When follicles enter the growth phase, they enlarge, both by proliferation of granulosa cells and by an increase in size of the oocyte. At the end of oocyte growth, it has acquired the capacity to resume meiosis. Normally, it has been accepted the notion that the oocyte growth is already finalized at the antral stage, significantly before follicle development is completed. In fact, while for several days the antral follicle experiences a further expansion in preparation for ovulation, no increase in oocyte size is observed [102]. Early studies indicated that the size-dependent ability for meiotic competence depends not only on the sizes of the follicle and oocyte but also on the stage of the menstrual cycle.
Most mRNA and protein are synthesized during the period of oocyte growth. Meanwhile, macromolecules and organelles are produced and stored in very large amounts [103]. Normally, it is believed that the ability to complete maturation to metaphase II and developmental competence is acquired progressively with increasing follicular size. In mice, it has been reported that developmental competence is dependent on both the size of the follicle and the size of oocytes [104]. It has been reported that the human oocyte has a size-dependent ability to resume meiosis from 90 to 120 μm in diameter [105], non-full-size oocytes should not be considered when assessing developmental competence, because the non-full-size oocytes have less products (mRNA and protein) stored in the cytoplasm during oocyte growth. The relationship between oocyte size and methylation imprints could indicate that imprint establishment requires the accumulation of proteins involved in the enzymatic process, in support of this hypothesis, the expression of the DNA methyltransferase genes, Dnmt3a, Dnmt3b, and Dnmt3L, peaked in oocytes from postnatal day 15 ovaries [106].
In clinical treatment, the use of gonadotropins has resulted in asynchrony of follicular development [2]. The size of the leading follicle seems do not affect the fertilization and cleavage rates of cohort oocytes from gonadotropin stimulated cycles [107]. However, it has been reported that fertilization rates are lower in oocytes obtained from the size of follicles <10 mm in diameter than in those retrieved from larger follicles [108]. It must be noted that immature oocytes are retrieved frequently after human chronic hormone (HCG) administration even from the size of follicles >10 mm in diameter, and these immature oocytes can be matured and developed in vitro following in vitro fertilization [109]. Importantly, some observations suggest that germinal vesicle (GV) stages of oocytes obtained from antral follicles are apparently morphologically similar, but in fact, they can be developed differently. This has important implications for human IVM because it underpins the concept that meiotic failure can reflect an intrinsic oocyte characteristic, irrespective of the ability of current IVM systems to fully support maturation.
Mechanism of Oocyte Maturation
Follicle development is accompanied by a parallel phase of oocyte growth, by which the oocyte reaches full size in preparation for fertilization and pre-implantation development. Fully growing oocytes prepare for fertilization and embryonic development by accumulating essential maternal materials and by undergoing genomic modifications during oocyte growth. The fully growing oocytes can be entered to maturation process in vivo by LH surge. Normally, the oocyte maturation can be divided into nuclear and cytoplasmic maturation, as well as membrane maturation and genomic maturation. Nuclear maturation refers to the resumption of meiosis and progression to metaphase II. Cytoplasmic maturation is a term that refers to preparation of oocyte cytoplasm for fertilization and embryonic development [109, 110]. Oocyte membrane maturation can be referred the process of the oocytes need to be primed with steroids to develop Ca2+ oscillations during maturation [111, 112]. Genomic maturation refers to the establishment of the correct epigenetic status. Modifications of the oocyte chromosome complement and rearrangements of cytoplasmic components are also crucial for the achievement of developmental competence. Several factors determine the ultimate competence of the oocyte, and these have been investigated and attempts made to mimic these conditions in vitro.
The details of mechanism for oocyte maturation will be discussed in Chap. 3; therefore, here we will briefly mention about the mechanism of oocyte maturation.
Nuclear Maturation
Nuclear maturation is defined as to the resumption of meiosis and completion of the first meiotic division from the GV stage to metaphase II (M-II). The oocyte acquires developmental competence during its growth within the follicle, and then, the pre-ovulatory surge of LH in vivo initiates germinal vesicle breakdown (GVBD). The mechanisms involved in GVBD are not fully understood. For many years, different lines of evidence generated mainly in the mouse model have indicated cAMP as the fundamental factor by which meiotic arrest is ensured before ovulation. It has been hypothesized that breakdown in gap junctional communication between the oocyte and granulosa cells at the time of the pre-ovulatory LH surge results in a decrease in cAMP levels within the oocyte, leading to the inactivation of the PKA pathway [110, 111, 113].
Many potential factors mediate the cumulus cell control of GVBD. The gap junctions permit regulatory molecules, such as steroids, Ca2+, inositol 1,4,5-trisphosphate, cAMP, and purines, to pass freely between the cytoplasm of the oocyte and cumulus cells [114]. Some elements of this regulatory network (e.g., NPPC and EGF family members) have been identified in the mouse model and are under close scrutiny as candidates for the development of more advanced IVM systems [115]. Protein synthesis is needed for the progression of oocytes from the GV stage to M-II [114, 116, 117], as well as for the maintenance of M-II arrest [118]. Cytoplasmic proteins, maturation promoting factor (MPF), and cytostatic factor (CSF) regulate oocyte nuclear maturation [119]. Inhibition of protein synthesis in oocytes results in failure to activate MPF activity [120].
Throughout oocyte growth, prophase arrest was thought to be correlated with low levels of cell cycle regulatory proteins, such as MPF. Molecular characterization of MPF has shown that active MPF is a protein dimer composed of catalytic p34cdc2 serine/threonine kinase, and regulatory cyclin B subunits [121]. The p34cdc2 serine/threonine kinase is the product of the cdc2 gene, and the p34cdc-cyclin heterodimer, a protein kinase, has four phosphorylation sites that are regulated by kinase and phosphatase activities. The concentration of cyclin increases steadily through interphase, peaks at the G2/M phase transition, and falls precipitously at each mitosis. Cyclins have been divided into three classes, G1, A, and B, based on their amino acid similarity and timing of their appearance during the cell cycle [122]. Two isoforms of cyclin B have been described in the mouse [123]. The expression patterns of cyclin B1 and B2 differ, with the cyclin B1 isoform predominantly expressed in the oocytes. Cyclin B is phosphorylated and dephosphorylated during oocyte maturation [124]. It is known that the product of the c–mos proto-oncogene is a protein–serine/threonine kinase and has the same effect as CSF. The product of c–mos is expressed early in oocyte maturation and disappears immediately after fertilization [125]. Therefore, M-II arrest may be due to the transcription of c–mos as the oocyte mature.
Mitogen-activated protein kinase (MAPK) has been revealed as central to the regulation of meiotic arrest in oocytes. MAPK is also a serine/threonine kinase but is activated, not inhibited, by tyrosine phosphorylation. Activation of MAPK precedes activation of p34cdc2. Blocking MAPK activity prevents GVBD. However, MAPK is not necessarily required for GVBD in mouse oocytes [126]. A product of c–mos stimulates MAPK activity, but does not activate p34cdc2 [127, 128]. The phosphorylation cascade of c–mos product and MAPK may play an important role in meiotic and mitotic cell cycles. In humans, MAPK is inactive in immature oocytes, active in mature oocytes, and the activity decreases after pronuclear formation after fertilization [129]. However, the mechanisms involved in GVBD, as well as the cell signaling pathways driving the oocyte into M-II in response to pre-ovulatory LH surge, are not fully understood [110].
Cytoplasmic and Membrane Maturation
Cytoplasmic maturation is a term that refers to preparation of oocyte cytoplasm for fertilization and embryonic development [87, 130]. RNA molecules, proteins, and imprinted genes are accumulated in the oocyte cytoplasm during its growth phase and are used to sustain the early phase of embryonic development before embryo DNA transcription begins [9, 33]. Rapid initiation of expression and high rates of transcription and translation during oocyte growth and folliculogenesis are followed by differential translation silencing and degradation of many mRNA species at the time of ovulation [131], so the oocyte and early pre-embryo are dependent upon the pool of mRNA and protein accumulated during the pre-ovulatory period [132]. Some maternal transcripts are even stored after the maternal to embryonic transition of gene expression has been completed [133]. Cytoplasmic factors of the oocyte may be responsible for maternal effects on de novo methylation and gene expression [99]. It is also known that insufficient cytoplasmic maturation of the oocyte will fail to promote male pronuclear formation and will thus increase chromosomal abnormalities after fertilization [134].
During oocyte growth, acute activation of a variety of signal transduction pathways and opening of ion channels has been observed in target cells within a few minutes of steroid exposure [135]. Many of these rapid steroid actions are non-genomic and initiated at the surface of the target cell by binding to membrane receptors [136]. It has been suggested that estrogen may act at the oocyte surface by producing changes in reactivity of its Ca2+ release system during cytoplasmic maturation [137, 138]. Oocytes need to be primed with estradiol to develop Ca2+ oscillations during maturation. Therefore, the process can be referred as oocyte membrane maturation [111, 112].
The preferential growth of the dominant follicle is associated with increased aromatase activity and a rapid elevation of circulating and follicular fluid estradiol-17β. The actions of estrogen are mediated through binding specifically to nuclear estrogen receptors, ligand-activated regulatory proteins that act as dimers on specific target genes containing defined DNA sequences called estrogen response elements [139]. Estrogen receptor binding to estrogen response elements can result in induction or suppression of responsive genes. Therefore, estrogen may be involved in the events of cytoplasmic maturation of the oocyte.
It seems that oocytes also require a specific intra-follicular progesterone environment for the inductive signals of cytoplasmic and membrane maturation, because pre-ovulatory follicular fluid contains certain concentrations of progesterone [140, 141]. Besides non-genomic effects of progesterone, the actions of progesterone are mediated through binding specifically to nuclear progesterone receptors [142]. The maturation of granulosa cells is associated with stimulation of the phosphatidylinositol pathway, involving the mobilization of intracellular Ca2+ and an increase in protein kinase C, which together stimulate a reduction in progesterone. Therefore, oocyte maturation is associated with a shift from estradiol to progesterone production by the granulosa cells; it is possible that progesterone may be involved in the development of an oocyte membrane Ca2+ release system. However, the role of progesterone in the oocyte cytoplasmic and membrane maturation is not fully understood.
Endocrine control of oocyte growth by gonadotropins rests on a network of intra-follicular paracrine interactions. Normally, it has been thought that FSH is essential for ovarian follicular development, whereas LH is primarily responsible for ovulation and transformation of follicles into the corpus luteum. Although the importance of gonadotropins in gonadal development and reproductive function has been established, the mechanism of gonadotropins on follicle growth and oocyte maturation is not fully understood. In the ovary, FSH binds to FSH receptors located on mural granulosa cells and acts via the cAMP-dependent protein kinase pathway. In the follicle, the enhanced FSH responsiveness of pre-ovulatory follicles also appears to result from an increase in the content of the stimulatory G protein of the adenyl cyclase system [120, 143]. The induction of LH receptors by FSH is one of the hallmarks of the differentiating mural granulosa cells [119]. Theca cells constitutionally contain LH receptors. LH is capable of stimulating androgen substrate production from theca cells into FSH-stimulated granulosa cells to transform estrogen [144] and that the thecal layer is the major cellular source of follicular androgen. In addition, LH is thought to stimulate progesterone production of mural granulosa and cumulus cells in pre-ovulatory follicles [145, 146]. LH may synergize with FSH to sustain follicle development as well as to prepare it for the mid-cycle LH surge that triggers ovulation [147].
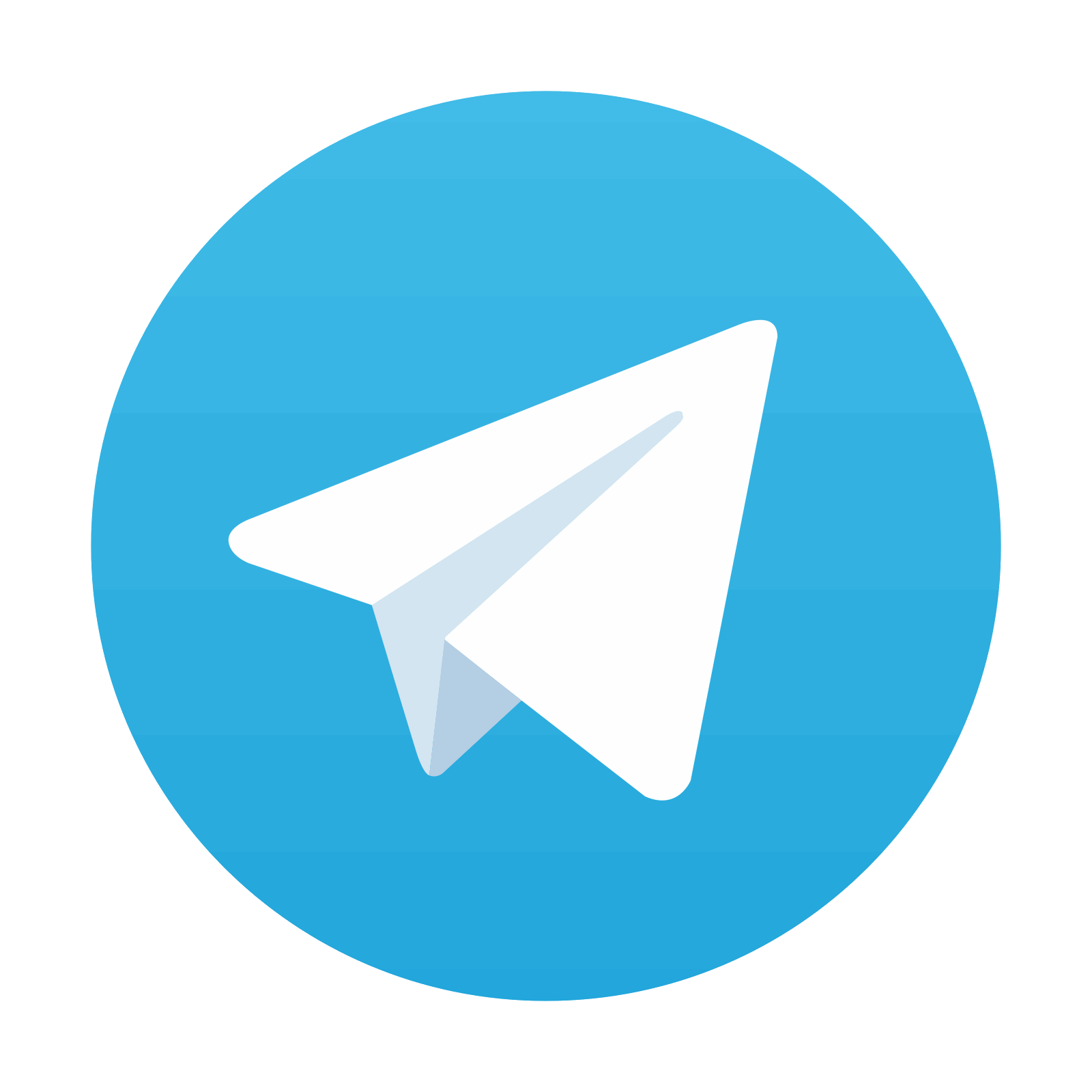
Stay updated, free articles. Join our Telegram channel

Full access? Get Clinical Tree
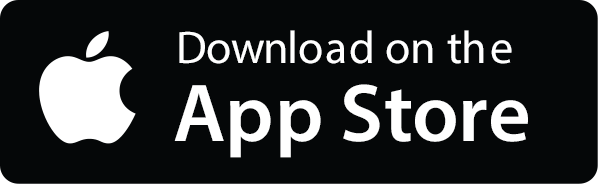
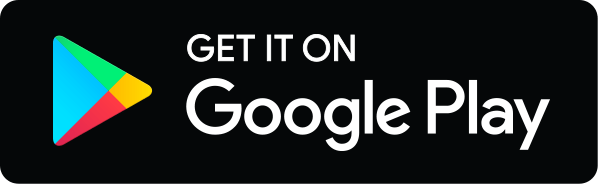