Fluid and Electrolyte Balance
Sharon M. Weinstein
KEY TERMS
Active Transport
Dehydration
Diffusion
Filtration
Glycosuria
Homeostatic Mechanisms
Hyperkalemia
Hypernatremia
Hypokalemia
Hyponatremia
Metabolic Acidosis
Metabolic Alkalosis
Osmosis
Respiratory Acidosis
Respiratory Alkalosis
Stress Response
OVERVIEW OF PHYSIOLOGY
An imbalance of fluid or electrolyte levels is recognized as a threat to life. Never before has the nurse’s responsibility for fluid and electrolyte administration and monitoring been more intense, and this applies to all clinical settings in which care is delivered. Patient outcomes depend in large part on specialty practice skills, which are based on a sound knowledge of physiology, in particular of fluid and electrolyte interplay.
Understanding fluid and electrolyte balance begins with understanding the intake, output, and utilization of water and electrolytes. Regulating mechanisms include osmosis, diffusion, filtration, and active transport, all of which affect the movement of water and electrolytes within the body.
Regulating Mechanisms
OSMOSIS
Osmosis is a passive transport mechanism that allows the movement of water freely through the semipermeable membrane. During this process, fluid moves in relation to the concentration of the solutes, taking it from an area of low solute concentration and continuing until both sides of the membrane are of equal concentration.
DIFFUSION
Diffusion is also a passive transport mechanism and represents the random movement of molecules and ions from an area of higher concentration to an area of lower concentration in a solution. An example is the exchange of oxygen and carbon dioxide between the alveoli and capillaries in the lungs (Chernecky, Macklin, & Murphy-Ende, 2002).
FILTRATION
Filtration refers to the movement of solutes and water through selectively permeable membranes, always moving from an area of higher pressure to an area of lower pressure. Filtration involves the movement of solutes and water in relation to hydrostatic pressure. While osmosis and diffusion are a response to concentrations, filtration is a response to pressure (Alexander, Corrigan, Gorski, Hankins, & Perucca, 2010).
ACTIVE TRANSPORT
Fluids passively move from higher concentrations/pressures to lower concentrations/pressure to equalize the concentrations on either side of a semipermeable membrane with osmosis, diffusion, and filtration.
At times, a higher concentration is required on one side of the membrane than on the other. Active transport is a mechanism that utilizes energy to achieve equilibrium. Particles are actually pushed out of the cells through a membrane while other particles are drawn in (Elkin, Perry, & Potter, 2007). Two types of active transport are known: primary and secondary. Adenosine triphosphate is the energy source for primary active transport, an example of which is the sodium-potassium pump. When there is an increase of sodium concentration within the cell, this usually activates the “pump” and a sodium/potassium ion exchange begins.
There are two forms of secondary active transport including cotransport and countertransport. With cotransport, the molecule or ion with a higher concentration outside the cell creates a storehouse of energy that will be used for its transport into the cell (Guyton & Hall, 2006).
Dehydration
Dehydration lowers the blood volume, decreasing renal blood flow, and the kidneys produce less of the ammonia needed to maintain acid-base balance. Severe dehydration may lower the blood volume enough to cause circulatory shock and oliguria (Collins & Claros, 2011).
Water is one of the most important nutrients for maintaining life and ensuing healthy aging. Body fluids move nutrients, gases, and wastes throughout the body; are essential in metabolizing food into energy; and assist in the body’s overall functions. The body loses water from the lungs, skin, gastrointestinal tract, and kidneys on a regular basis. There is a small, but significant, amount of water lost in the stool in normal conditions. The renal and stool loss of water is known traditionally as sensible water loss. Although the kidney can minimize the loss of fluid, it can never totally conserve water (Klotz, 1998).
The evaporative water loss that occurs through the lungs and skin is known as insensible water loss. Insensible water loss is independent of the body water and solute (electrolytes) and content. It does, however, depend on the patient’s body surface area and environmental temperature (Adelman & Solhung, 1996).
At least 80% of all fluids administered today contain some electrolytes. Because electrolyte therapy is often a lifesaving procedure, its safe and successful administration is essential. Knowledge of the fundamentals of fluid and electrolyte metabolism contributes to safe electrolyte therapy.
Abnormalities of body fluid and electrolyte metabolism present therapeutic problems. Treatment is complex when the mechanisms that normally regulate fluid volume, electrolyte composition, and osmolality are impaired. An understanding of these metabolic abnormalities enables the nurse to recognize the problems involved. Such clinical challenges arise in patients with renal insufficiency, adrenal insufficiency, adrenal hyperactivity, and other kinds of impaired organ function.
The two following examples illustrate how knowledge of fluid and electrolyte metabolism contributes to safe, successful therapy in the critically ill patient. Correction of a severe potassium deficit resulting from vomiting and diarrhea presents a problem in the dehydrated patient. Potassium replacement is imperative. However, potassium administered to patients with renal insufficiency results in potassium toxicity because the kidneys are unable to excrete electrolytes. The adverse effects of excess potassium on the heart muscle are arrhythmia and heart block. The nurse must recognize the importance of both hydrating the patient before potassium can be administered safely and watching for diminished diuresis that could necessitate a change in therapy. Once antidiuresis occurs, the potassium infusion must be interrupted and the physician/licensed independent practitioner (LIP) notified. Potassium is the chief cation of body cells (160 mEq/L of intracellular water) and is concerned with the maintenance of body fluid composition and electrolyte balance. Potassium participates in carbohydrate utilization and protein synthesis and is critical in the regulation of nerve conduction and muscle contraction, particularly in the heart. Chloride, the major extracellular anion, closely follows the metabolism of sodium, and changes in the acid-base of the body are reflected by changes in the chloride concentration.
Normally about 80% to 90% of the potassium intake is excreted in the urine and the remainder in the stools and, to a small extent, in the perspiration. The kidney does not conserve potassium well so that during fasting, or in patients on a potassium-free diet, potassium loss from the body continues resulting in potassium depletion. A deficiency of either potassium or chloride will lead to a deficit of the other.
Another example relates to therapeutic problems in patients with impaired liver function. When excessive loss of gastric fluid occurs, replacement fluid is necessary. Most deficits caused by gastric suction, unless severe, are treated with 0.9% sodium chloride in 5% dextrose in water or 0.33% sodium chloride in 5% dextrose in water. However, severe loss may call for gastric replacement fluids containing ammonium chloride, which can be potentially
dangerous when administered to patients with impaired liver function. Ammonium chloride administered to a patient with severe liver damage may result in ammonia intoxication because of the liver’s inability to convert ammonia to hydrogen ions and urea.
dangerous when administered to patients with impaired liver function. Ammonium chloride administered to a patient with severe liver damage may result in ammonia intoxication because of the liver’s inability to convert ammonia to hydrogen ions and urea.
Fluid Content of the Body
The total body water content of a person varies with age, weight, and sex. The amount of water depends on the amount of body fat. Body fat is essentially water free; the greater the fat content, the lower the water content.
In contrast to infants, who have a high body fluid content (approximately 70% to 80% of the body weight), approximately 60% of a typical adult’s weight consists of fluid (water and electrolytes; Figure 8-1). After 40 years of age, mean values for total body fluid in percentage of body weight decrease for both men and women; a sex differentiation, however, remains. After 60 years of age, the percentage may decrease to 52% in men and 46% in women. With aging, lean body mass decreases in favor of fat (Metheny, 2012).
Fluid Compartments
The total body fluid is functionally allocated into two main compartments: the intracellular and the extracellular compartments (Figure 8-2). The intracellular compartment consists of the fluid inside the cells and comprises approximately two thirds of the body fluid, or 40% of the body weight. The extracellular compartment consists of the fluid outside the body cells—the plasma, representing 5% of the body weight, and the interstitial fluid (fluid in tissues), representing 15% of the body weight (Metheny, 2012).
In newborn infants, the proportion is approximately three-fifths intracellular and twofifths extracellular. This ratio changes and reaches the adult level by the time the infant is approximately 30 months of age.
There is one additional compartment, the transcellular compartment. The transcellular fluid is the product of cellular metabolism and consists of secretions such as gastrointestinal secretions and urine. An analysis of the secretions may assist the practitioner in tracing lost electrolytes and prescribing proper fluid and electrolyte replacement. Excessive fluid and electrolyte loss must be replaced to maintain fluid and electrolyte balance in the two main
compartments. The amount of body water loss is easily computed by weighing the patient and noting loss of weight: 1 L body water is equivalent to 1 kg, or 2.2 lb, of body weight. Up to 5% weight loss in a child or adult may signify moderate fluid volume deficit— > 5% may indicate severe fluid volume deficit. Weight changes are also valuable as indicators of body water gains—acute weight gain may indicate water excess.
compartments. The amount of body water loss is easily computed by weighing the patient and noting loss of weight: 1 L body water is equivalent to 1 kg, or 2.2 lb, of body weight. Up to 5% weight loss in a child or adult may signify moderate fluid volume deficit— > 5% may indicate severe fluid volume deficit. Weight changes are also valuable as indicators of body water gains—acute weight gain may indicate water excess.
Body Fluid Composition
Body fluid contains two types of solutes (dissolved substances): the electrolytes and the nonelectrolytes. Electrolytes serve two main purposes: to act in controlling body water volume by osmotic pressure and to maintain the proper acid-base balance of the body. The nonelectrolytes are molecules that do not break into particles in solution but remain intact. They consist of dextrose, urea, and creatinine.
Electrolytes are molecules that break into electrically charged particles called ions. The ion carrying a positive charge is called a cation, and the ion with a negative charge is called
an anion. Potassium chloride is an electrolyte that, dissolved in water, yields potassium cations and chloride anions. Chemical balance is always maintained; the total number of positive charges equals the total number of negative charges. The quantity of charges and their concentration are expressed as milliequivalents (mEq) per liter of fluid. Because the number of negative charges must equal the number of positive charges for chemical balance, the milliequivalents of cations must equal the milliequivalents of anions.
an anion. Potassium chloride is an electrolyte that, dissolved in water, yields potassium cations and chloride anions. Chemical balance is always maintained; the total number of positive charges equals the total number of negative charges. The quantity of charges and their concentration are expressed as milliequivalents (mEq) per liter of fluid. Because the number of negative charges must equal the number of positive charges for chemical balance, the milliequivalents of cations must equal the milliequivalents of anions.
Electrolyte Composition
Each fluid compartment has its own electrolyte composition. The extracellular compartment (plasma and interstitial fluid) contains a high concentration of sodium, chloride, and bicarbonate and a low concentration of potassium. The composition of the intracellular fluid is quite different; the concentrations of potassium, magnesium, and phosphate are high, whereas the sodium and chloride concentrations are relatively low.
Electrolyte composition of the intracellular fluid is in part related to electrolyte composition of the plasma and interstitial fluids. Disturbances in the extracellular fluid (ECF) are reflected in the patient’s symptoms; this information, combined with an analysis of plasma, is thus a valuable guide to therapy.
Occasionally, however, the electrolyte determination of plasma may be misleading. For example, the concentration of potassium in plasma may be high while there is a body deficit. This surplus is due to the shift of potassium from intracellular to ECF in the process of large potassium losses through the kidneys. Determination of plasma sodium may also present a false picture. In the case of an edematous cardiac patient, the plasma concentration may be low despite excess body sodium because total body sodium is equal to the sum of the products of volume times concentration in the various compartments.
Characteristics of Fluids
Fluids are described in various ways. Significant characteristics of fluids include their concentration, or osmolality, as well as their acidity or alkalinity (pH).
OSMOLALITY
Osmolality is the total solute concentration and reflects the relative water and total solute concentration; it is expressed per liter of serum. Osmotic pressure is determined by the amount of solutes in solution. If the ECF contains a relatively large number of dissolved particles and the intracellular fluid contains a small amount of dissolved particles, the osmotic pressure causes water to pass from the less concentrated fluid to the more concentrated. Therefore, fluid from the intracellular compartment passes into the extracellular compartment until the concentration becomes equal.
The unit of osmotic pressure is the osmole, and values are expressed in milliosmoles (mOsm). Normal blood plasma has an osmolality of approximately 290 mOsm/kg water. The determination of serum osmolality is sometimes used to detect dehydration or overhydration. Measurement of sodium concentration also indicates the water needs of the body. At times, the osmolality reading may falsely indicate dehydration. Because the osmolality is the total solute concentration, nonelectrolytes are included in the reading. An elevated blood urea level can therefore increase the osmolality without exerting osmotic pressure.
A determination of blood urea nitrogen (BUN) may supply a correction to the osmolality reading in cases of increased serum urea.
A determination of blood urea nitrogen (BUN) may supply a correction to the osmolality reading in cases of increased serum urea.
CONCENTRATION AS A METRIC VALUE
Concentrations of solutes may be expressed in several ways in addition to milliequivalents per liter, such as milligrams per deciliter (mg/dL) or millimoles per liter (mmol/L). Each of these units of measure may be used in a clinical setting. A milliequivalent of an ion is its atomic weight expressed in milligrams divided by the valence. This is the measure most often used for expressing small concentrations of electrolytes in body fluids because it emphasizes the principles that ions combine milliequivalent for milliequivalent.
CONCENTRATION AS AN SI VALUE
Milligrams per 100 mL (deciliter) express the weight of the solute per unit volume. In the International System of Units (Système Internationale, SI), electrolyte content in body fluids is expressed in millimoles. For practical purposes, 1 mole (mol) of a substance has a mass equal to its atomic weight, expressed in grams. For example, a mole of sodium has a mass of 23 g (the atomic weight of sodium is 23). A millimole is one thousandth of a mole or the molecular or atomic weight expressed in milligrams. Therefore, a millimole of sodium equals 23 mg. Sometimes, it is necessary to convert from millimoles per liter to milliequivalents per liter (Figure 8-3). The following formula applies:
mEq/L = mmol/L × valence
ACID-BASE BALANCE
The acidity or alkalinity of a solution depends on the degree of hydrogen ion concentration. An increase in the hydrogen ions results in a more acid solution; a decrease results in a more alkaline solution. Acidity is expressed by the term pH, which refers to the amount of hydrogen ion concentration. A solution having a pH of 7 is regarded as neutral.
The ECF has a pH ranging from 7.35 to 7.45 and is slightly alkaline. When the pH of the blood is > 7.45, an alkaline condition exists; when < 7.35, an acid condition exists.
The biologic fluids, both extracellular and intracellular, contain a buffer system that maintains the proper acid-base balance. This buffer system consists of fluid with salts of a weak acid or weak base. A base or hydroxide neutralizes the effect of an acid. These weak acids and bases maintain pH values by soaking up surplus ions or releasing them; acids yield hydrogen ions; bases accept hydrogen ions.
The carbonic acid-sodium bicarbonate system is the most important buffer system in the extracellular compartment. The normal ratio is 1 part of carbonic acid to 20 parts of base bicarbonate, which represents 1.2 mEq carbonic acid to 24 mEq base bicarbonate.
ACID-BASE IMBALANCE
Acid-base imbalances are normally the result of an excess or a deficit in either base bicarbonate or carbonic acid. Deviations of pH from 7.35 to 7.45 are combated by the buffer system and by the respiratory and renal regulatory mechanisms. Two types of disturbance can affect the acid-base balance: respiratory and metabolic. A diagrammatic presentation of these disturbances is presented in Figure 8-4.
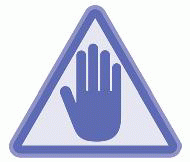
The patient with electrolyte imbalance may have a disturbance of sensorium, putting him or her at risk for falls. All precautions should be taken to protect the patient.
RESPIRATORY DISTURBANCES
Respiratory disturbances affect the carbonic side of the balance by increasing or decreasing carbonic acid; when carbon dioxide unites with ECF, carbonic acid is produced.
Respiratory alkalosis results when excess carbon dioxide is exhaled during rapid or deep breathing. Carbonic acid is depleted because of the carbon dioxide loss. Respiratory alkalosis may occur as the result of emotional disturbances, such as anxiety and hysteria, and also from lack of oxygen or from fever (Metheny, 2012).
Symptoms are convulsions, tetany, and unconsciousness. Laboratory determination is a urinary pH > 7 and a plasma bicarbonate concentration < 24 mEq/L. The body attempts to restore the ratio to normal by depressing the bicarbonate to compensate for the deficit in the carbonic acid.
Respiratory acidosis occurs when exhalation of carbon dioxide is depressed and the excess retention of carbon dioxide increases the carbonic acid. It may occur in conditions that interfere with normal breathing, such as emphysema, asthma, and pneumonia. Symptoms are weakness, disorientation, depressed breathing, and coma. Urinary pH is below 6, and plasma bicarbonate concentration is above 24 mEq/L. The bicarbonate increase is due to the body’s attempt to restore the carbonic acid-bicarbonate ratio.
METABOLIC DISTURBANCES
Metabolic disturbances affect the bicarbonate side of the balance. Kidney function controls the bicarbonate concentration by regulating the amount of cations (hydrogen, ammonium, and potassium) in exchange for sodium ions to combine with the reabsorbed bicarbonate in the distal tubular lumen. As hydrogen ions are excreted, bicarbonate is generated, maintaining the proper acid-base balance of the blood. Ammonia excretion is increased in response to a high acidity; bicarbonate replaces the ammonia.
Metabolic alkalosis is a condition associated with excess bicarbonate; it occurs when chloride is lost. Chloride and bicarbonate are both anions, and the total number of anions must equal the total number of cations. When chloride anions are lost, the deficit must be
made up by an equal number of anions to maintain electrolyte equilibrium; bicarbonate increases in compensation and alkalosis occurs.
made up by an equal number of anions to maintain electrolyte equilibrium; bicarbonate increases in compensation and alkalosis occurs.
Metabolic alkalosis is also associated with decreased levels of intracellular potassium. Potassium escapes from the cell into the ECF and is lost through the transcellular fluid. When body potassium is lost, the shift of sodium and hydrogen ions from the ECF causes alkalosis, whereas the increase of hydrogen ions in the intracellular fluid causes cellular acidosis.
Muscular hyperactivity, tetany, and depressed respiration are symptoms of metabolic alkalosis. Muscular hyperactivity and tetany are symptoms of the deficit in ionized calcium that exists in alkalosis. Laboratory determinations are urinary pH > 7, plasma pH > 7.45, and bicarbonate level > 24 mEq/L.
Treatment consists of the administration of fluids containing chloride to replace bicarbonate ions. An excess of bicarbonate ions is accompanied by potassium deficiency, so potassium must also be replaced.
Metabolic acidosis is a condition associated with a deficit in the bicarbonate concentration. This occurs when
Excessive amounts of ketone acids accumulate, as in uncontrolled diabetes or starvation.
Inorganic acids, such as phosphate and sulfate, accumulate, as in renal disease.
Excessive losses of bicarbonate occur from gastrointestinal drainage or diarrhea.
Acidosis may occur also from intravenous (IV) administration of excessive amounts of sodium chloride or ammonium chloride, causing chloride ions to flood the ECF.
Stupor, shortness of breath, weakness, and unconsciousness are the symptoms of metabolic acidosis, and laboratory determinations of metabolic acidosis are a urinary pH < 6, plasma pH < 7.35, and plasma bicarbonate concentration < 24 mEq/L.
Treatment and clinical management consist of increasing the bicarbonate level. Solutions of sodium lactate are often used, but because lactate ion must be oxidized to carbon dioxide before it can affect the acid-base balance, it is advisable to use sodium bicarbonate solutions, which are effective even when the patient is suffering from oxygen lack.
Homeostatic Mechanisms
The body uses regulating mechanisms called homeostatic mechanisms to maintain the constancy of body fluid volume, electrolyte composition, and osmolality. These mechanisms function through the renocardiovascular, endocrine (adrenal, pituitary, and parathyroid), and respiratory systems. The kidneys, skin, and lungs are the main regulating agents (Metheny, 2012).
KIDNEY FUNCTION
The kidney plays a major role in fluid and electrolyte balance. To function adequately, the kidney depends on its own soundness as well as on the coordination of all the regulating organs. The distal renal tubules in the kidney are important in regulating body fluid. They selectively retain or reject electrolytes and other substances to maintain normal osmolality and blood volume. Sodium is retained, and potassium is excreted.
The kidneys also play an important part in acid-base regulation. The distal tubule has the ability to form ammonia and exchange hydrogen ions (in the form of ammonia) for bicarbonate to maintain the carbonic acid-bicarbonate ratio.
SKIN AND LUNG FUNCTION
The skin and lungs play an important role in fluid balance—the skin in loss of fluid through insensible perspiration and the lungs in loss of fluid by expiration. Normal intake of 2,500 mL from all sources delivers a loss of approximately 1,000 mL in breath and perspiration, 1,400 mL in urine, and 100 mL in feces.
FUNCTIONS OF OTHER SYSTEMS AND ORGANS
The renocardiovascular system maintains fluid balance by regulating the amount and composition of urine. Plasma must reach the kidneys in sufficient volume to permit regulation of water and electrolyte balance. Renal disease, cardiac failure, shock, postoperative stress, and alarm impair this regulating mechanism.
The adrenal glands influence the retention or excretion of sodium, potassium, and water. These glands secrete aldosterone, a hormone that increases the reabsorption of sodium from the renal tubules in exchange for potassium, thus maintaining normal sodium concentration. Any stress, such as surgery, increases the secretion of aldosterone, thus increasing the reabsorption of sodium bicarbonate. Adrenal hyperactivity also increases the secretion of aldosterone and causes excess sodium retention. Excess loss of sodium occurs with adrenal insufficiency.
The pituitary gland is another important organ in the control of fluid and electrolyte balance. The posterior lobe of the pituitary releases antidiuretic hormone (ADH), which inhibits diuresis by increasing water reabsorption in the distal tubule. Increased concentration of sodium in the ECF stimulates the pituitary to release ADH. This hormone increases the reabsorption of water to dilute the sodium to the normal level of concentration. Increased body fluid osmolality, decreased body fluid volume, stress, and shock are conditions that increase ADH secretion. Increased body fluid volume, decreased osmolality, and alcohol inhibit ADH secretion.
The parathyroid glands, pea-sized glands embedded in the corners of the thyroid gland, regulate calcium and phosphate balance by means of parathyroid hormone (PTH). When the calcium level is low, PTH secretion is stimulated. This acts on bone to increase reabsorption of bone salts, releasing large amounts of sodium into the ECF. When the extracellular calcium concentration is too high, PTH secretion is depressed so that almost no bone reabsorption occurs (Metheny, 2012).
The pulmonary system regulates acid-base balance by controlling the concentration of carbonic acid through exhalation or retention of carbon dioxide.
Electrolytes of Biologic Fluids
Electrolyte content of the intracellular fluid differs from that of the ECF. Because specialized techniques are required to measure electrolyte concentration in the intracellular fluid, it is customary to measure the electrolytes in the ECF, chiefly plasma. Plasma electrolyte concentrations may be used to assess and manage patients with a diversity of electrolyte imbalances. Although some tests are performed on serum, the terms serum electrolytes and
plasma electrolytes are used interchangeably. Table 8-1 identifies intracellular and extracellular concentrations and serum values. Table 8-2 lists plasma electrolyte values.
plasma electrolytes are used interchangeably. Table 8-1 identifies intracellular and extracellular concentrations and serum values. Table 8-2 lists plasma electrolyte values.
TABLE 8-1 INTRACELLULAR AND EXTRACELLULAR CONCENTRATIONS AND RELATED SERUM VALUES | |||||||||||||||||||||||||||||||||||||||||
---|---|---|---|---|---|---|---|---|---|---|---|---|---|---|---|---|---|---|---|---|---|---|---|---|---|---|---|---|---|---|---|---|---|---|---|---|---|---|---|---|---|
|
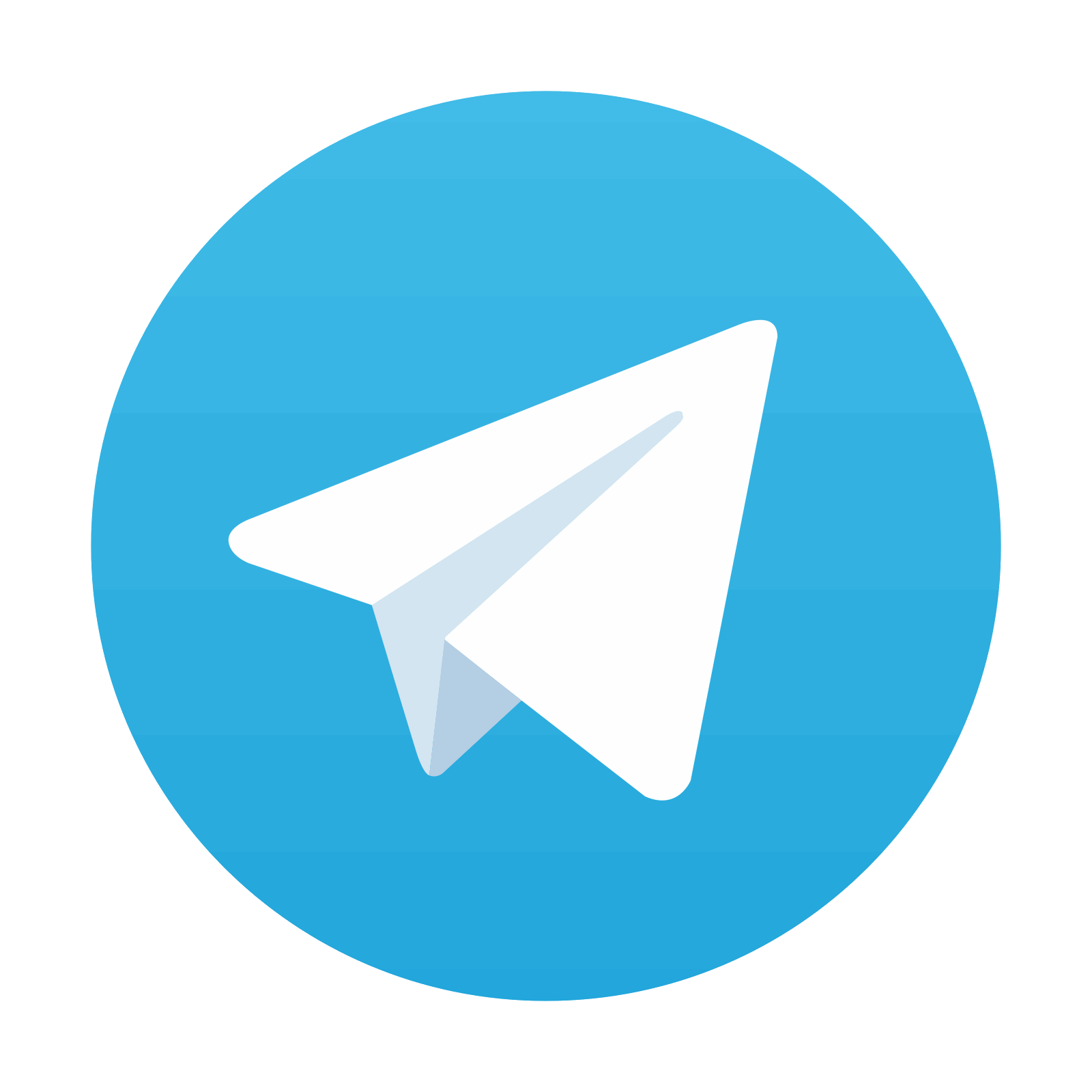
Stay updated, free articles. Join our Telegram channel

Full access? Get Clinical Tree
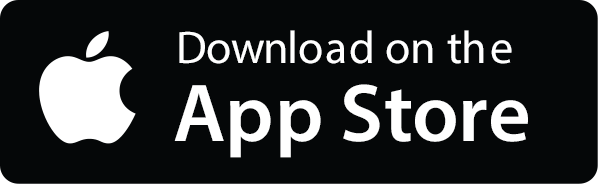
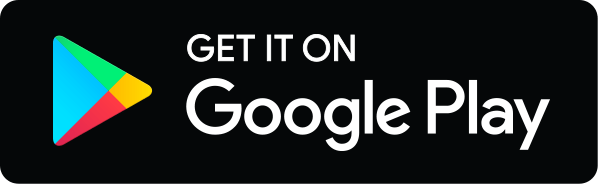